Abstract
Activated macrophages contribute to endothelial dysfunction; however, it is unclear how peroxynitrite contributes to macrophage-mediated human cardiac microvascular endothelial cell (HCMEC) injury in hypoxia. In macrophage-HCMEC co-cultures subjected to hypoxia, there was an increase in hypoxia-inducible factor (HIF)-1α, HIF-2α, inducible nitric oxide synthase (iNOS), endothelin-converting enzyme (ECE)-1 and cyclooxygenase-2 (COX-2), and concomitant decrease in prostacyclin synthase (PGIS). This was mimicked by a peroxynitrite donor and attenuated by its decomposition catalyst. Tongxinluo (TXL) could decrease HIF-2α, iNOS, ECE-1 and COX-2 and increase PGIS in a dose-dependent manner, with increase of vascular endothelial growth factor. The protein alterations verified the remarkably affected mRNAs, indicating that the effects of TXL were similar to but better than that of peroxynitrite decomposition catalyst. Furthermore, TXL inhibited macrophage-mediated nitrotyrosine accumulation and attenuated HCMEC injury. The results suggest that peroxynitrite contributes to macrophage-mediated HCMEC injury in hypoxia, and TXL attenuates HCMEC injury mainly by inhibiting peroxynitrite.
INTRODUCTION
Cardiovascular disease is a leading cause of death worldwide. Atherosclerosis and the subsequent vessel destruction are the primary cause of ischemic diseases, such as stroke and coronary heart disease (Schiano et al., Citation2014; Tano & Gollasch, Citation2014). Microvascular endothelial dysfunction is believed to be the initiating process and pathological basis of cardiovascular disease (Ding et al., Citation2014; Steyers & Miller, Citation2014; Tousoulis et al., Citation2014).
Hypoxia-inducible factors (HIFs) are transcription factors that respond to hypoxia. Mammalian HIFs function as heterodimers composed of either HIF-1α or HIF-2α bound to HIF-1β. HIF-1α appears to be ubiquitously expressed, whereas HIF-2α is more restricted to vascular endothelial cells (Skuli et al., Citation2012; Kapitsinou et al., Citation2014). HIFs control a series of genes that help in adaptation to hypoxia. Vascular endothelial growth factor (VEGF) is a major target gene of HIFs, which promotes angiogenesis in hypoxia (Oh et al., Citation2015). Oxidative stress and inflammation have been widely accepted to be the key pathological processes of cardiac microvascular endothelial cells (Liu et al., Citation2014; Mangge et al., Citation2014). Inflammation and oxidative stress-related genes can be activated by hypoxia (Shweta et al., Citation2014). Both inducible nitric oxide synthase (iNOS) and cyclooxygenase-2 (COX-2) are inducible enzymes in inflammation and oxidative stress, and can cause pathological damage (Li et al., Citation2008; Tunctan et al., Citation2013). In contrary to inducible iNOS, constitutive expression of endothelial NOS (eNOS) is necessary for physiological vasodilation (Liu et al., Citation2014). Endothelin-converting enzyme-1 (ECE-1) is a crucial component of the endothelin signaling pathway, and is an important regulator of vascular tone (Robinson et al., Citation2014). In contrast to COX-2, prostacyclin synthase (PGIS) antagonizes inflammation and has cytoprotective properties (He et al., Citation2010; Camacho et al., Citation2011). In addition to VEGF, HIFs also contribute to the trans-activation of iNOS, ECE-1 and COX-2, which leads to injury (Branco-Price et al., Citation2012; Khamaisi et al., Citation2015; Zhao et al., Citation2015).
It has been reported that macrophages, which can be activated by hypoxia, have the ability to alter gene expression of endothelial cells (Weldy et al., Citation2011; Mei et al., Citation2014). Once macrophages are activated, iNOS is robustly induced to produce excess peroxynitrite (Cunningham-Bussel et al., Citation2013; Schirmer et al., Citation2015). The most conspicuous effect of peroxynitrite is the nitration of tyrosine residues in proteins to form nitrotyrosine, which is thought to be a marker of peroxynitrite-induced injury (Li et al., Citation2008). Since peroxynitrite can easily diffuse between cells, it is unknown whether macrophages aggravate endothelial cell injury in hypoxia through peroxynitrite.
Tongxinluo (TXL) is a compound made from a group of herbal medicines, such as ginseng, radix paeoniae rubra, borneol and spiny jujuba seed, and contains multiple active components that may be responsible for its antianginal effects (Li et al., Citation2010; Jia et al., Citation2011; Li et al., Citation2013). TXL is then concentrated and freeze-dried before use. TXL was registered in 1996 in the State Food and Drug Administration of China for the treatment of angina pectoris. Our previous study revealed that ginsenoside, the main active component of TXL, could inhibit COX-2 and iNOS in an L-methionine-induced rat vascular lesion model (Li et al., Citation2008). Furthermore, we found that TXL could modulate gene expression in hypoxia-treated human cardiac microvascular endothelial cells (HCMECs) (Li et al., Citation2015). However, many factors affect HCMECs during hypoxia, including activated macrophages (Tausendschön et al., Citation2015; Yang et al., Citation2015). It is unknown if TXL attenuates the macrophage-mediated HCMEC injury in hypoxic conditions. In addition, the underlying mechanism of action of TXL has not been clarified.
In this study, HCMECs were used to investigate whether macrophages in co-culture exacerbate HCMEC injury via peroxynitrite during hypoxia, and to determine the mechanism of action of TXL. And peroxynitrite donor 3-morpholinosy-dnonimine (SIN-1) and its decomposition catalyst 5,10,15,20-Tetrakis-(4-sulfonatophenyl)porphyrinato-iron(III) (FeTPPS) were used as tool drugs.
MATERIALS AND METHODS
Cells and treatment
HCMECs were purchased from ScienCell and cultured in Endothelial Cell Medium ECM (ScienCell, Carlsbad, CA). Bone marrow-derived macrophages were a gift from Professor Jinkun Wen (Zhang et al., Citation2014). Initially, macrophages were treated with CoCl2 (dissolved in phosphate-buffered saline (PBS), resulting in 100 μmol/L final concentration) to induce hypoxia (Li et al., Citation2015), and gene expressions of HIF-1α, HIF-2α, VEGF, iNOS, COX-2 and PGIS measured at different times to reflect macrophage activation. Subsequently, after HCMECs were co-cultured with macrophages and treated with CoCl2, HCMEC mRNA of HIF-1α, HIF-2α, VEGF, eNOS, iNOS, ECE-1, COX-2 and PGIS was measured at 24 h to verify the effects of macrophages in hypoxia-treated HCMECs.
In another set of experiments, HCMECs were divided into control (Con), hypoxia plus macrophages (Hx + Mϕ), SIN-1, Hx + Mϕ + FeTPPS, Hx + Mϕ + low-dose-TXL (200 μg/mL), Hx + Mϕ + middle-dose-TXL (400 μg/mL), Hx + Mϕ + high-dose-TXL (600 μg/mL) and middle-dose TXL groups. After 24 h treatment, mRNA levels were measured. In addition, protein level of the remarkably altered genes was also measured. Furthermore, cell morphological alterations were observed by light microscope, and nitrotyrosine content was evaluated at 24 h treatment.
Real-time RT-PCR
The mRNA expression of HIF-1α, HIF-2α, VEGF, eNOS, iNOS, ECE-1, COX-2 and PGIS in HCMECs and the mRNA expression of HIF-1α, HIF-2α, VEGF, iNOS, COX-2 and PGIS in macrophages was quantified by real-time RT-PCR. Total RNA was isolated using Trizol reagent (Takara, Dalian, China) and reverse transcribed to cDNA using RevertAid First Strand cDNA synthesis Kit (Fermentas, Shanghai, China), followed by real-time PCR amplification using specific primers (). Actin primers were used as an internal standard.
Table 1. Primers used for real time PCR detection.
Western blotting
After 24 h treatment, the protein levels of HIF-2α, VEGF, iNOS, ECE-1, COX-2 and PGIS were measured by western blotting, using a previously described protocol (Li et al., Citation2015). Briefly, cells were lyzed in lysis buffer (RIPA, Solarbio, Beijing, China) to extract total proteins. Protein content was determined by NanoDrop-1000 (Thermo Scientific, Shanghai, China). Then the samples were separated by sodium dodecyl sulfate polyacrylamide gel electrophoresis and transferred to the polyvinylidene fluoride membrane (Millipore, Darmstadt, Germany). After the membranes were blocked with milk, anti-HIF-2α (Sangon Biotech, Shanghai, China), anti-VEGF (Sangon Biotech, Shanghai, China), anti-iNOS (Santa Cruz, Texas, USA), anti-ECE-1 (Santa Cruz, Texas, USA), anti-COX-2 (Santa Cruz, Texas, USA), anti-PGIS (Santa Cruz, Texas, USA), or anti-beta actin (Sangon Biotech, Shanghai, China) antibodies were used. Band intensities were quantified and calculated.
Immunofluorescence
Nitrotyrosine content was measured by immunofluorescence using a procedure that was described previously (Li et al., Citation2013). After incubation with anti-nitrotyrosine antibody (Abcam, Shanghai, China) and fluorescein isothiocyanate-conjugated secondary antibody (Zhongshan, Beijing, China), the cells were observed under a fluorescence microscope (Olympus IX51, Tokyo, Japan). PBS was used instead of the primary and secondary antibodies as control.
Statistical analysis
Statistical analysis of the data was performed using SPSS software and presented as means ± SD. One-way analysis of variance was used to analyze the differences among the groups, and the differences between two groups were evaluated by least-significant-difference (LSD). The experiments were repeated three times and p < 0.05 was considered statistically significant.
RESULTS
Macrophage co-culture aggravated gene expressions in hypoxia-treated HCMECs
The cells were divided into Con, Hx, Hx + Mϕ and Mϕ groups, and after 24 h treatment, mRNAs of HIF-1α, HIF-2α, VEGF, eNOS, iNOS, ECE-1, COX-2 and PGIS in HCMECs were measured by real-time RT-PCR (). Compared with Con, HIF-2α and VEGF rose obviously in Hx group, HIF-2α was induced with slight increase of HIF-1α in Hx + Mϕ group, and VEGF increased significantly in Mϕ group. Compared with Hx, VEGF decreased significantly in Hx + Mϕ group (). Compared with Con, the oxidative stress-related iNOS elevated sharply in Hx and Hx + Mϕ groups, but ECE-1 increased in all groups, with no significant changes of eNOS. Compared with Hx, ECE-1 even elevated significantly in Hx + Mϕ group (). Compared with Con, COX-2 increased and PGIS had a tendency to decrease in Hx and Hx + Mϕ groups, while PGIS was induced significantly in Mϕ group ().
Figure 1. Macrophage co-culture aggravated gene expressions in hypoxia-stimulated HCMECs. The gene mRNAs were measured by real-time RT-PCR. (A) The changes of HIF-1α, HIF-2α and VEGF in control (Con), hypoxia (Hx), hypoxia plus macrophage co-culture (Hx + Mϕ) and macrophage co-culture (Mϕ) groups. (B) The changes of eNOS, iNOS and ECE-1 in Con, Hx, Hx + Mϕ and Mϕ groups. (C) The changes of COX-2 and PGIS in Con, Hx, Hx + Mϕ and Mϕ groups. **p < 0.01 or *p < 0.05, versus Con group. ##p < 0.01 or #p < 0.05, versus Hx group. (D) The time-dependent expression of iNOS in hypoxia-activated macrophages. **p < 0.01 or *p < 0.05, versus 0 h group. The data were repeated three times and represented as mean ± SD.
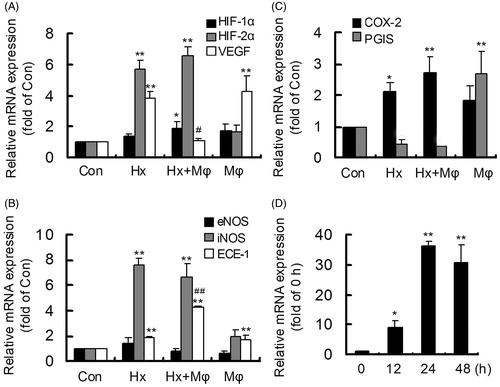
In addition, except the endothelial cell mainly expressed eNOS and ECE-1, other gene mRNAs in hypoxia-activated macrophages were also detected (supplemental Figure 1). Relative to other genes, iNOS mRNA increased sharply in hypoxia-activated macrophages, especially after 24 h hypoxia treatment, indicating the optimal co-culture time (). The results showed that relative to hypoxia-induced HIF-2α, VEGF, iNOS, ECE-1 and COX-2, macrophage co-culture sharply triggered ECE-1, but suppressed VEGF with a slight increase of HIF-1α, thus exacerbating HCMEC injury in hypoxia.
TXL adjusted the macrophage-aggravated genes mainly via inhibiting peroxynitrite
After 24-h treatment, mRNAs of HIF-1α, HIF-2α, VEGF, eNOS, iNOS, ECE-1, COX-2 and PGIS were measured by real-time RT-PCR. Compared with Con, both HIF-1α and HIF-2α increased in Hx + Mϕ and SIN-1 groups. Compared with Hx + Mϕ, HIF-1α decreased in Hx + Mϕ + FeTPPS, Hx + Mϕ + low dose TXL (LT) and Hx + Mϕ + middle dose TXL (MT) groups, HIF-2α decreased in Hx + Mϕ + FeTPPS, Hx + Mϕ + middle dose TXL (MT) and Hx + Mϕ + high dose TXL (HT) groups, and VEGF increased in Hx + Mϕ + LT and Hx + Mϕ + MT groups. Compared with Hx + Mϕ +FeTPPS, HIF-2α was high in Hx + Mϕ + LT group and low in Hx + Mϕ + HT group, and VEGF was elevated in Hx + Mϕ + LT and Hx + Mϕ + MT groups. The results showed that macrophage co-culture in hypoxia induced HIF-1α and especially HIF-2α, which could be mimicked by SIN-1 and inhibited by FeTPPS. The elevated HIF-2α could be inhibited by TXL in a concentration-dependent manner, while VEGF increased significantly for LT and MT treatments. Besides elevation of VEGF, the effects of MT were most similar to that of FeTPPS ().
Figure 2. Gene expression of HIF-1α, HIF-2α and VEGF in treated HCMECs. **p < 0.01, versus Con group; ##p < 0.01 or #p < 0.05, versus Hx + Mϕ group; ††p < 0.01, versus Hx + Mϕ+ FeTPPS group. The data were repeated three times and represented as mean ± SD. Con, control; Hx + Mϕ, hypoxia plus macrophage co-culture; LT, low dose TXL; MT, middle dose TXL; HT, high dose TXL.
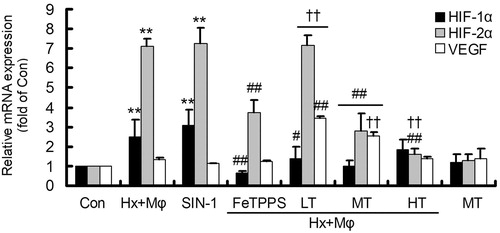
Compared with Con, iNOS and ECE-1 increased in Hx + Mϕ and SIN-1 groups, without distinct changes of eNOS. Compared with Hx + Mϕ, both iNOS and ECE-1 decreased in Hx + Mϕ + FeTPPS and all dose of TXL groups, with eNOS increased in Hx + Mϕ + HT group. Compared with Hx + Mϕ + FeTPPS, iNOS and ECE-1 was high in Hx + Mϕ + LT group. The results revealed that the increased iNOS and ECE-1 in Hx + Mϕ group could be mimicked by SIN-1 and inhibited by FeTPPS. The induced iNOS and ECE-1 could be suppressed by TXL in a concentration-dependent manner and the MT-caused effects were most similar to that of FeTPPS ().
Figure 3. Gene expression of eNOS, iNOS and ECE-1 in treated HCMECs. **p < 0.01, versus Con group; ##p < 0.01 or #p < 0.05, versus Hx + Mϕ group; ††p < 0.01, versus Hx + Mϕ + FeTPPS group. The data were repeated three times and represented as mean ± SD. Con, control; Hx + Mϕ, hypoxia plus macrophage co-culture; LT, low dose TXL; MT, middle dose TXL; HT, high dose TXL.
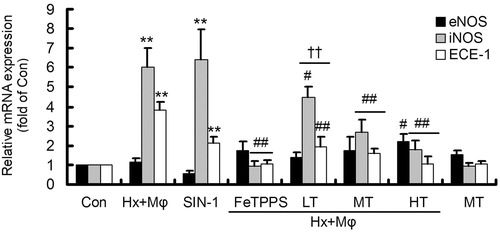
Compared with Con, COX-2 increased and PGIS had a tendency to decrease in Hx + Mϕ and SIN-1 groups. Compared with Hx + Mϕ, COX-2 decreased and PGIS increased in Hx + Mϕ + FeTPPS and all dose of TXL groups. Compared with Hx + Mϕ + FeTPPS, COX-2 was low in Hx + Mϕ + MT and Hx + Mϕ + HT groups, and PGIS was even low in Hx + Mϕ + LT group. The results suggested that the induced COX-2 in Hx + Mϕ group could be mimicked by SIN-1 and inhibited by FeTPPS. TXL concentration-dependently suppressed COX-2 and elevated PGIS, similar to the effects of FeTPPS ().
Figure 4. Gene expression of COX-2 and PGIS in treated HCMECs. **p < 0.01, versus Con group; ##p < 0.01 or #p < 0.05, versus Hx + Mϕ group; ††p < 0.01, versus Hx + Mϕ + FeTPPS group. The data were repeated three times and represented as mean ± SD. Con, control; Hx + Mϕ, hypoxia plus macrophage co-culture; LT, low dose TXL; MT, middle dose TXL; HT, high dose TXL.
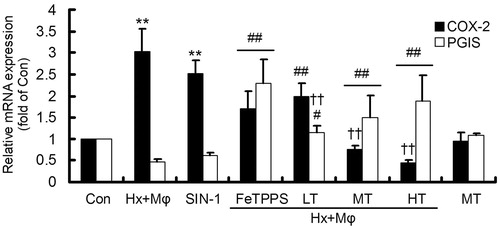
In addition, MT itself had no effect on the gene mRNAs. These results manifested that the macrophage-mediated gene mRNAs could be mimicked by the peroxynitrite donor SIN-1 and reversed by its decomposition catalyst FeTPPS, indicating that peroxynitrite may be an intermediary in the macrophage-mediated changes in mRNAs in hypoxia-stimulated HCMECs. Similar to the effects of FeTPPS, TXL could reverse the macrophage-mediated HIF-1α, HIF-2α, iNOS, ECE-1, COX-2 and PGIS, with increase of VEGF in hypoxia-stimulated HCMECs, to play a protective role.
Protein levels confirmed the remarkably changed gene mRNAs
The cells were divided into Con, Hx + Mϕ, SIN-1, Hx + Mϕ + FeTPPS and Hx + Mϕ + MT groups, and after 24 h treatment, protein levels of HIF-2α, VEGF, iNOS, ECE-1, COX-2 and PGIS were measured by western blotting (). The increased HIF-2α in Hx + Mϕ group could be mimicked by SIN-1 and inhibited by FeTPPS, and besides inhibiting HIF-2α, MT significantly increased VEGF (). The induced iNOS and ECE-1 in Hx + Mϕ group could be mimicked by SIN-1 and inhibited by FeTPPS and MT (). The elevated COX-2 and inhibited PGIS in Hx + Mϕ group could be mimicked by SIN-1 and reversed by MT, but FeTPPS treatment only significantly reversed the reduced PGIS (). The results ascertained that the macrophage-mediated HIF-2α, iNOS, ECE-1 and PGIS in hypoxia-stimulated HCMECs may be mediated by peroxynitrite, and TXL could adjust the affected HIF-2α, iNOS, ECE-1, COX-2 and PGIS, with increase of VEGF, to block HCMEC injury.
Figure 5. Protein levels of the remarkably altered genes in treated HCMECs. (A) Protein levels were detected by western blotting. (B) The changes of HIF-2α and VEGF in control (Con), hypoxia plus macrophage co-culture (Hx + Mϕ), SIN-1, Hx + Mϕ + FeTPPS and Hx + Mϕ + MT groups. (C) The changes of iNOS and ECE-1 in Con, Hx + Mϕ, SIN-1, Hx + Mϕ + FeTPPS and Hx + Mϕ + MT groups. (D) The changes of COX-2 and PGIS in Con, Hx + Mϕ, SIN-1, Hx + Mϕ + FeTPPS and Hx + Mϕ + MT groups. **p < 0.01 or *p < 0.05, versus Con group; ##p < 0.01 or #p < 0.05, versus Hx + Mϕ group. The data were repeated three times and represented as mean ± SD. MT, middle dose TXL.
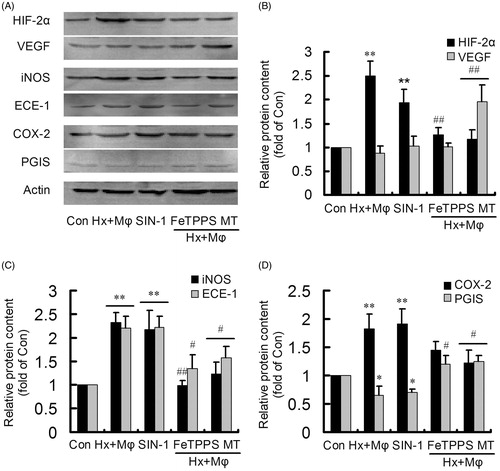
TXL inhibited macrophage-induced nitrotyrosine in hypoxia-stimulated HCMECs
After the macrophage co-cultured HCMECs in hypoxia were treated with MT for 24 h, nitrotyrosine content was analyzed by immunofluorescence (). As illustrated, in contrast to Con group, cells shrank, turned round and seem to die in Hx + Mϕ group, but for MT treatment, the morphological cell injury was overtly attenuated. Correspondingly, the nitrotyrosine staining was not evident in Con group, but conspicuous in Hx + Mϕ group. The macrophage-induced nitrotyrosine accumulation could be inhibited obviously by MT treatment. The results demonstrated that TXL could inhibit the macrophage-induced nitrotyrosine accumulation in hypoxia-stimulated HCMECs to attenuate cell injury.
Figure 6. TXL inhibited nitrotyrosine formation to attenuate HCMEC injury (400×). The nitrotyrosine content was evaluated by immunofluorescence. The upper ones were HCMEC morphological alterations and the lower showed nitrotyrosine fluorescence. Con, control; Hx + Mϕ, hypoxia plus macrophage co-culture; MT, middle dose TXL.
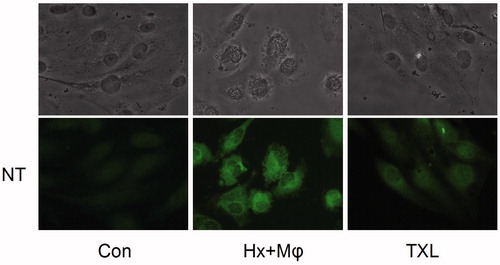
DISCUSSION
Besides VEGF, HIFs contrarily induce oxidative stress and inflammation-related proteins to drive the pathological process of endothelial dysfunction, which is considered as the initiating process and pathological basis of cardiovascular disease. Since activated macrophages contribute to endothelial cell injury, it is unclear if peroxynitrite underlies the macrophage-mediated HCMEC injury in hypoxia. TXL has been shown to effectively improve cardiac microcirculation and block the effects of hypoxia; however, its underlying mechanism of action is not fully known. In this study, we found that the macrophage-induced gene activation and protein expression in hypoxia-stimulated HCMECs is mediated by peroxynitrite. TXL could reverse the macrophage-mediated changes in hypoxia-stimulated HCMECs, and its effects were similar to but better than that of peroxynitrite decomposition catalyst. Furthermore, TXL inhibited the macrophage-induced nitrotyrosine accumulation in hypoxia-stimulated HCMECs to attenuate cell injury. The results indicate that peroxynitrite may contribute to the macrophage-mediated HCMEC injury in hypoxia, and TXL could attenuate cell injury mainly via inhibiting peroxynitrite production.
The immense endothelium surface area in the microcirculation blood vessels makes this tissue more vulnerable to injury. Since the endothelial cell layer constitutes the primary internal barrier of blood vessels, microvascular endothelial dysfunction is believed to be the initiating process and pathological basis of cardiovascular disease (Ding et al., Citation2014; Steyers & Miller, Citation2014; Tousoulis et al., Citation2014; Hald et al., Citation2014). Although activated macrophages aggravate endothelial cell injury in hypoxia, the mediator has not been defined (Weldy et al., Citation2011; Mei et al., Citation2014). In this study, HCMECs were co-cultured with macrophages in a hypoxia model to investigate whether peroxynitrite contributes to the macrophage-mediated heart microvascular endothelial cell injury, and if injury could be prevented by treatment with TXL.
HIF-1α and HIF-2α are induced by hypoxia, which can drive VEGF expression, resulting in angiogenesis (Skuli et al., Citation2012; Kapitsinou et al., Citation2014; Oh et al., Citation2015). Contrary to iNOS, eNOS plays constitutive roles in physiological vasodilatation and ECE-1 is also an important regulator of vascular tone (Liu et al., Citation2014; Robinson et al., Citation2014). COX-2 and PGIS are enzymes with opposing actions in inflammation (He et al., Citation2010; Camacho et al., Citation2011). Besides VEGF, iNOS, ECE-1 and COX-2 are other target genes of HIFs in hypoxia (Branco-Price et al., Citation2012; Khamaisi et al., Citation2015; Zhao et al., Citation2015). Whether activated macrophages affect these factors in hypoxia-stimulated HCMECs via peroxynitrite is unknown.
At first, mRNAs of HIF-1α, HIF-2α, VEGF, eNOS, iNOS, ECE-1, COX-2 and PGIS were detected in HCMECs co-cultured with macrophages and exposed to hypoxia. The results showed that relative to hypoxia-induced activation of HIF-2α, VEGF, iNOS, ECE-1 and COX-2, macrophage co-culture sharply triggered ECE-1, but suppressed VEGF with a slight increase of HIF-1α in hypoxia-treated HCMECs, resulting in oxidative stress, inflammation and insufficient angiogenesis. Then it was found that the macrophage-mediated mRNA could be mimicked by the peroxynitrite donor SIN-1 and reversed by its decomposition catalyst FeTPPS. The parallel changes in protein levels ascertained the remarkably affected mRNAs, indicating that macrophages could exacerbate gene expression in hypoxia-stimulated HCMECs possibly via peroxynitrite, leading to more severe injury.
TXL has satisfactory beneficial effects on cardiovascular disease, particularly in the modulation of microcirculation (Li et al., Citation2010; Li et al., Citation2013; ia et al., 2011; Cui et al., Citation2014). With regard to the mechanism underlying TXL, several researchers have focused on the regulation of eNOS, and still others explore its effect on micro RNAs (Zhang et al., Citation2014; Wang et al., Citation2014). Although HIFs, oxidative stress and inflammation are considered as the key pathological processes of HCMEC injury in hypoxia, the effects of TXL on these aspects have not been explored.
In this study, changes in both mRNA and protein levels showed that TXL could reverse the macrophage-affected HIF-1α, HIF-2α, iNOS, ECE-1, COX-2 and PGIS, attenuating oxidative stress and inflammation, similar to the effects of peroxynitrite decomposition catalyst FeTPPS. Moreover, TXL elevated VEGF in HCMECs co-cultured with macrophage in hypoxia to promote angiogenesis, superior to the effects of FeTPPS. The results manifested that TXL may adjust the disordered factors mainly though inhibiting peroxynitrite. However, opposing effects of HIF-2α inhibition and VEGF elevation by TXL in macrophage-stimulated HCMECs in hypoxia need further clarification of the underlying mechanism.
Furthermore, the morphological alterations illustrated that although hardly seen in Con group, nitrotyrosine content was conspicuous in macrophage co-cultured HCMECs in hypoxia, and the macrophage-induced nitrotyrosine accumulation could be inhibited obviously by TXL treatment, consistent with cell morphological changes. The results demonstrated that TXL could inhibit the macrophage-triggered nitrotyrosine accumulation in hypoxia-stimulated HCMECs to attenuate cell injury, suggesting a protective mechanism of TXL.
CONCLUSIONS
Peroxynitrite mediated the macrophage co-culture aggravated HIF-1α, HIF-2α, iNOS, ECE-1, COX-2 and PGIS in hypoxia-treated HCMECs, triggering oxidative stress and inflammation to cause HCMEC injury, and TXL could reverse the disordered genes with elevation of VEGF to attenuate HCMEC injury, mainly via inhibiting peroxynitrite.
Supplementary Material
Download PDF (338.4 KB)Acknowledgments
The authors thank Professor Jinkun Wen for offering macrophages.
Disclosure statement
The authors report no declarations of interest. The authors alone are responsible for the content and writing of the paper. This work was supported by grants from the Major State Basic Research Development Program of China (973 Program) (No. 2012CB518601), the National Natural Science Foundation of China (No. 81470595), the Hebei Natural Science Foundation (No. H2015206101).
References
- Branco-Price C , Zhang N , Schnelle M , Evans C , Katschinski DM , and Liao D. (2012). Endothelial cell HIF-1α and HIF-2α differentially regulate metastatic success. Cancer Cell. 21: 52–65.
- Camacho M , Rodríguez C , Guadall A , Alcolea S , Orriols M , and Escudero JR. (2011). Hypoxia upregulates PGI-synthase and increases PGI2 release in human vascular cells exposed to inflammatory stimuli. J Lipid Res. 52: 720–731.
- Cui H , Li X , Li N , Qi K , Li Q , Jin C. (2014). Induction of autophagy by tongxinluo through the MEK/ERK pathway protects human cardiac microvascular endothelial cells from hypoxia/reoxygenation injury. J Cardiovasc Pharmacol. 64: 180–190.
- Cunningham-Bussel A , Zhang T , Nathan CF. (2013). Nitrite produced by Mycobacterium tuberculosis in human macrophages in physiologic oxygen impacts bacterial ATP consumption and gene expression. Proc Natl Acad Sci USA. 10: E4256–E4265.
- Ding Y , Zhang B , Zhou K , Chen M , Wang M , Jia Y. (2014). Dietary ellagic acid improves oxidant-induced endothelial dysfunction and atherosclerosis: Role of Nrf2 activation. Int J Cardiol. 175: 508–514.
- Hald BO , Jacobsen JC , Sandow SL , Holstein-Rathlou NH , Welsh DG. (2014). Less is more: minimal expression of myoendothelial gap junctions optimizes cell-cell communication in virtual arterioles. J Physiol. 592: 3243–3255.
- He C , Choi HC , Xie Z. (2010). Enhanced tyrosine nitration of prostacyclin synthase is associated with increased inflammation in atherosclerotic carotid arteries from type 2 diabetic patients. Am J Pathol. 176: 2542–2549.
- Jia Y , Bao F , Huang F , Leung SW. (2011). Is tongxinluo more effective than isosorbide dinitrate in treating angina pectoris? A systematic review and meta-analysis of randomized controlled trials. J Altern Complement Med. 17: 1109–1117.
- Kapitsinou PP , Sano H , Michael M , Kobayashi H , Davidoff O , Bian A. (2014). Endothelial HIF-2 mediates protection and recovery from ischemic kidney injury. J Clin Invest. 124: 2396–2409.
- Khamaisi M , Toukan H , Axelrod JH , Rosenberger C , Skarzinski G , Shina A. (2015). Endothelin-converting enzyme is a plausible target gene for hypoxia-inducible factor. Kidney Int. 87: 761–770.
- Li XD , Yang YJ , Cheng YT , Dou KF , Tian Y , Meng XM. (2013). Protein kinase A-mediated cardioprotection of Tongxinluo relates to the inhibition of myocardial inflammation, apoptosis, and edema in reperfused swine hearts. Chin. Med. J 126: 1469–1479.
- Li XD , Yang YJ , Geng YJ , Jin C , Hu FH , Zhao JL. (2010). Tongxinluo reduces myocardial no-reflow and ischemia reperfusion injury 526 by stimulating the phosphorylation of eNOS via the PKA pathway. Am J Physiol Heart Circ Physiol. 299: H1255–H1261.
- Li Y , Zhao M , Li B , Qi J. (2013). Dynamic localization and functional implications of C-peptide might for suppression of iNOS in high glucose-stimulated rat mesangial cells. Mol Cell Endocrinol. 381: 255–260.
- Li YN , Wang XJ , Li B , Liu K , Qi JS , Liu BH , Tian Y. (2015). Tongxinluo inhibits cyclooxygenase-2, inducible nitric oxide synthase, hypoxia-inducible factor-2α/vascular endothelial growth factor to antagonize injury in hypoxia-stimulated cardiac microvascular endothelial cells. Chin Med J. 128: 1114–1120.
- Li YN , Wu YL , Jia ZH , Qi JS. (2008). Interaction between COX-2 and iNOS aggravates vascular lesion and antagonistic effect of ginsenoside. . J Ethnopharmacol. 119: 305–311.
- Liu B , Kuang L , Liu J. (2014). Bariatric surgery relieves type 2 diabetes and modulates inflammatory factors and coronary endothelium eNOS/iNOS expression in db/db mice. Can J Physiol Pharmacol. 92: 70–77.
- Liu Y , Lian K , Zhang L , Wang R , Yi F , Gao C. (2014). TXNIP mediates NLRP3 inflammasome activation in cardiac microvascular endothelial cells as a novel mechanism in myocardial ischemia/reperfusion injury. Basic Res Cardiol. 109: 415
- Mangge H , Becker K , Fuchs D , Gostner JM. (2014). Antioxidants, inflammation and cardiovascular disease. World J Cardiol. 6: 462–477.
- Mei Y , Thompson MD , Shiraishi Y , Cohen RA , Tong X. (2014). Sarcoplasmic/endoplasmic reticulum Ca(2+) ATPase C674 promotes ischemia- and hypoxia-induced angiogenesis via coordinated endothelial cell and macrophage function. J Mol Cell Cardiol. 76: 275–282.
- Oh SY , Seok JY , Choi YS , Lee SH , Bae JS , Lee YM. (2015). The histone methyltransferase inhibitor BIX01294 inhibits HIF-1α stability and angiogenesis. Mol Cells. 38: 528–534.
- Robinson AS , Materna SC , Barnes RM , De VERSUS , Xu SM , Black BL. (2014). An arterial-specific enhancer of the human endothelin converting enzyme 1 (ECE1) gene is synergistically activated by Sox17, FoxC2, and Etv2. Dev Biol. 395: 379–389.
- Schiano C , Casamassimi A , Vietri MT , Rienzo M , Napoli C. (2014). The roles of mediator complex in cardiovascular diseases. Biochim Biophys Acta. 1839: 444–451.
- Schirmer SH , Millenaar DN , Werner C , Schuh L , Degen A , Bettink SI. (2015). Exercise promotes collateral artery growth mediated by monocytic nitric oxide. Arterioscler Thromb Vasc Biol. 35: 1862–1871.
- Shweta M,KP , Chanda S , Singh SB , Ganju L. (2014). A comparative immunological analysis of CoCl2 treated cells with in vitro hypoxic exposure. Biometals. 28: 175–185.
- Skuli N , Majmundar AJ , Krock BL , Mesquita RC , Mathew LK , Quinn ZL. (2012). Endothelial HIF-2α regulates murine pathological angiogenesis and revascularization processes. J Clin Invest. 122: 1427–1443.
- Steyers CM 3rd. , Miller FJ. Jr. (2014). Endothelial dysfunction in chronic inflammatory diseases. . Int J Mol Sci. 15: 11324–11349.
- Tano JY , Gollasch M. (2014). Hypoxia and ischemia-reperfusion: a bik contribution? Am J Physiol Heart Circ Physiol. 307: 811–817.
- Tausendschön M , Rehli M , Dehne N , Schmidl C , Döring C , Hansmann ML , Brüne B. (2015). Genome-wide identification of hypoxia-inducible factor-1 and -2 binding sites in hypoxic human macrophages alternatively activated by IL-10. Biochim Biophys Acta. 1849: 10–22.
- Tousoulis D , Simopoulou C , Papageorgiou N , Oikonomou E , Hatzis G , Siasos G. (2014). Endothelial dysfunction in conduit arteries and in microcirculation. Novel therapeutic approaches. Pharmacol Ther. 144: 253–267.
- Tunctan B , Korkmaz B , Sari AN , Kacan M , Unsal D , Serin MS. (2013). Contribution of iNOS/sGC/PKG pathway, COX-2, CYP4A1, and gp91(phox) to the protective effect of 5,14-HEDGE, a 20-HETE mimetic, against vasodilation, hypotension, tachycardia, and inflammation in a rat model of septic shock. Nitric Oxide. 33: 18–41.
- Wang B , Yang Q , Bai WW , Xing YF , Lu XT , Sun YY. (2014). Tongxinluo protects against pressure overload-induced heart failure in mice involving VEGF/Akt/eNOS pathway activation. PLoS One. 9: e98047.
- Weldy CS , Wilkerson HW , Larson TV , Stewart JA , Kavanagh TJ. (2011). DIESEL particulate exposed macrophages alter endothelial cell expression of eNOS, iNOS, MCP1, and glutathione synthesis genes. Toxicol In Vitro. 25: 2064–2073.
- Yang F , Bai Y , Jiang Y. (2015). Effects of Apelin on RAW264.7 cells under both normal and hypoxic conditions. Peptides. 69: 133–143.
- Zhang RN , Zheng B , Li LM , Zhang J , Zhang XH , Wen JK. (2014). Tongxinluo inhibits vascular inflammation and neointimal hyperplasia through blocking positive feedback loop between miR-155 and TNF-α. Am J Physiol Heart Circ Physiol. 307: H552–H562.
- Zhao CX , Luo CL , Wu XH. (2015). Hypoxia promotes 786-O cells invasiveness and resistance to sorafenib via HIF-2α/COX-2. Med Oncol. 32: 419.