Abstract
Saquinavir (SQV) is a protease inhibitor that binds to the protease active site of the human immunodeficiency virus and prevents the cleavage of viral polyproteins resulting in the formation of non-infectious virus particles. The purpose of these studies was to determine the potential effects of SQV on the immune system in female B6C3F1 mice. SQV was administered by gavage twice daily for 28 days at total doses of 300, 600, and 1200 mg/kg/day. No significant differences were observed in body weight, or the weights of spleen, thymus, liver, kidneys, or lungs. Exposure to SQV produced no biologically meaningful changes in hematological parameters. However, a statistically significant increase in the number of T-cells (23%) was observed at the high dose level of SQV. The number of splenic immature T-cells (CD4+CD8+ cells) also showed increases of 46% and 92% at the 600 and 1200 mg/kg dose levels, respectively. The immunoglobulin M antibody-forming cell (AFC) response was significantly increased by 41% when the data were expressed as AFC/106 spleen cells at the 1200 mg/kg dose level. Treatment with SQV had no effects on the mixed leukocyte response. Overall, the activities of natural killer cells and cytotoxic T-cells were not altered in SQV-treated animals when compared to vehicle controls. In addition, exposure to SQV did not affect host resistance in the B16F10 melanoma model. In conclusion, SQV produced an enhancement of the humoral immune response, possibly through modulating T-cell function in female B6C3F1 mice.
Introduction
Highly active anti-retroviral therapy (HAART), including reverse transcriptase inhibitors and at least one human immunodeficiency virus (HIV) protease inhibitor (PI), has proven to be extremely effective in treating HIV-1 infection (Gruber et al., Citation2001). The PIs bind to the catalytic site of the viral protease enzyme, which plays a critical role in cleaving the large polyproteins of the immature virus. Saquinavir (SQV) is the first PI prescribed in the United States and works by interfering with late events of the viral life cycle during cleavage of the final viral protein element. This mechanism of action is an important component of HAART because it can be used in combination with nucleoside analogues that act at different sites in the viral cycle.
Although HIV-1 PIs were designed to be highly specific for the HIV-1 aspartic protease, SQV treatment in HIV patients has been shown to increase the T-cell proliferative response (Lu and Andrieu, Citation2000), to increase CD4+ cell counts (Winston et al., Citation2007), and to increase neutralizing antibody titer against sequential autologous HIV-1 isolates (Sarmati et al., Citation1997; Binley et al., Citation2000). In addition, SQV treatment has been shown to affect cytokine production (Pacifici et al., Citation1997; Bucy et al., Citation1999; Gruber et al., Citation2001). The observed immunomodulatory effects of SQV in HIV patients following PI treatment might be due to either the direct effect of PIs on the immune system because these proteases were also involved in physiological processes concerning immune responses (Franzese et al., Citation2001) or their virus inhibition (indirect effects) because of the immunosuppressive effects of HIV. Although Viora et al. (Citation1998) reported a lack of SQV modulation of proliferative responses in human peripheral blood mononuclear cells (PBMCs) and lamina propia mononuclear cells as well as no modulation in natural killer (NK) cell-mediated cytotoxic activity in vitro, the immunomodulatory effects of SQV in vivo have not been studied in detail. This was important because it has been reported that the PIs were metabolized in the liver, and some PIs were potent inhibitors of cytochrome P450 (Ernest et al., Citation2005).
The purpose of this study was to evaluate the effects of SQV on the immune responses of female B6C3F1 mice using assays that were designed to detect chemical-induced changes in cellular, humoral and innate immunities. In addition, there was evidence to show that anti-retroviral therapy using PIs could block several pathways involved in tumor growth, invasion, and metastasis (Sgadari et al., Citation2003), thus, host resistance to the B16F10 melanoma tumor was also employed. The B6C3F1 mouse is the National Toxicology Program’s designated mouse strain. A large database exists on this strain, and the major components of the immune system in the mouse and human are the same; many agents that perturb the immune system in humans perturb the immune system in the mouse in a similar manner. In contrast to the in vitro study (Viora et al., Citation1998), several significant changes were produced following the in vivo SQV treatment.
Materials and methods
Animals and animal exposure
Female B6C3F1 mice were obtained from Charles River Breeding Laboratories (Raleigh, NC). Mice arrived at 4–6 week-of-age, and were quarantined upon arrival. The mice were between 8- and 10-wk-old at the beginning of the studies. Mice were housed four per cage in plastic shoebox cages with hardwood chip bedding and consumed Harlan Teklad Laboratory Diets (NIH 07; Madison, WI) and tap water from water bottles ad libitum. Animal holding rooms were kept at 21–24°C and 40–60% relative humidity with a 12-hr light/dark cycle. All animal procedures were conducted under an animal protocol approved by the Virginia Commonwealth University Institutional Animal Care and Use Committee (IACUC).
SQV was obtained from Hoffman-LaRoche (Nutley, NJ) and administered by gavage; 0.5% methylcellulose was used as the vehicle. Because SQV had a low oral bioavailability (Perry and Noble, Citation1998) and a short elimination half-life (Hsu et al., Citation1998), SQV was administered twice daily for 28 days with a minimum of 8 hr between the two treatments. The chemical solutions were prepared as 15, 30, and 60 mg/mL, and the resulted SQV treatment doses were 300, 600, and 1200 mg/kg/day, respectively. The mice were randomized and then assigned to one of four treatment groups, with eight animals in each group. Animals received 0.1 mL/10 g body weight by gavage for 28 days. It should be noted that, in humans, SQV is usually administered together with ritonavir, an inhibitor of CYP3A4 that enhances the bioavailability of SQV (Eagling et al., Citation1997). Because the original studies demonstrating immunomodulatory effects of SQV did not include ritonavir (Sarmati et al., Citation1997; Sparano et al., Citation1998), ritonavir was not used in the present studies.
Concentration and effect relationships have been observed for many of the anti-retroviral drugs, especially the PIs. In humans, the minimum recommended effective concentration for SQV is 0.1 mg/L in serum, and treatment combining 1600 mg SQV and 100 mg ritonavir once daily can produce a maximum concentration (Cmax) of 0.98–12.36 mg/L and a mean area under the curve (AUC) of 17.88–67.05 mg·h/L for SQV-depending on body weight, gender, and study site (Autar et al., Citation2005). SQV has also been used in HIV patients without ritonavir (Sarmati et al., Citation1997). For example, treatment with SQV at 1200 mg daily in the absence of ritonavir produced a SQV AUC of 1.67 mg·h/L (Acosta et al., Citation2001). In mice, an oral dose of 500 mg/kg of SQV resulted in a Cmax of 1.72 mg/L and a SQV AUC of 8.56 mg·h/L (Washington et al., Citation2000). Thus, SQV daily doses of 300–1200 mg/kg in our studies were reasonably anticipated to produce AUCs that are relevant to human exposure.
Toxicological studies
Animals were examined for gross pathology at the end of exposure period. The mice were anesthetized by CO2 inhalation and sacrificed by cervical dislocation. The thymus, lungs, liver, spleen, and kidneys with adrenals were removed, cleaned of connective tissue, and weighed.
On the day when the animals were euthanized, blood was collected by retro-orbital bleed into EDTA tubes after the animals were anesthetized by CO2 inhalation. The following hematological parameters were assessed: the number of erythrocytes and leukocytes, hemoglobin, hematocrit, mean corpuscular volume (MCV), mean cell hemoglobin (MCH), and mean cell hemoglobin concentration (MCHC). Analysis was conducted using a K-1000 Hematology analyzer (Sysmex America, Inc., Mundelein, IL). The number of reticulocytes was measured using a Retic-count Reagent stain (Thiazole Orange, Becton Dickinson, Mountain View, CA) followed by flow cytometric analysis. At the time of blood collection, a blood smear was prepared, and air-dried. After fixation with methanol and staining with Wright-Giemsa (Fisher Scientific, Pittsburgh, PA), the smear was used to determine leukocyte differentials in blood.
Immunological studies
Spleen collection and single-cell suspension preparation
Spleens were collected and placed in 3 mL Earle’s balanced salt solution (EBSS) with HEPES (GibcoBRL, Grand Island, NY). Splenocyte suspensions were prepared by pressing the spleen between the frosted ends of two microscope slides into a 60 × 15-mm petri dish. The slides were washed with the buffer using a pasture pipette, and then single-cell suspensions were placed into 4-mL plastic test tubes. Splenocytes were then centrifuged at 300 × g for 5 min and resuspended in 3 mL RPMI 1640 (GibcoBRL) complete medium with 10% fetal bovine serum (Hyclone, Waltham, MA). The cell count was determined using a ZBII Coulter counter in the presence of ZAP-OGLOBIN II lytic reagent (Coulter Corp., Miami, FL).
Flow cytometric analysis of T-cells, T-cell subsets, B-cells, NK cells, and monocytes
The respective cell types were labeled with an appropriate monoclonal antibody (mAb) conjugated with a fluorescent molecule for visualization. For T-cell enumeration, a fluorescein isothiocyanate (FITC) conjugated anti-mouse CD3e mAb (diluted 1:80; BD Pharmingen, San Diego, CA) was used. For B-cell enumeration, a FITC-conjugated affinity purified anti-mouse immunoglobulin (diluted 1:80) was used (Becton Dickinson). For CD4+ cells, a phycoerythrin (PE)-conjugated mAb specific for the L3T4 cell surface protein was used, and for CD8+ cells, a FITC-conjugated mAb specific for the CD8a (Ly-2) marker (BD Pharmingen) was used. Anti-mouse NK1.1 with PE and anti-mouse Mac-3 with PE were used to label NK cells (CD3−NK1.1+) and monocytes, respectively. Isotype-matched irrelevant antibodies were used as controls. Following the addition of the reagents, the cells were incubated at 4°C in the dark for at least 30 min. After incubation, the cells were washed twice and propidium iodide was added. Following incubation with propidium iodide for 5 min, the cells were washed and enumeration performed on a Becton Dickinson FACScan Flow Cytometer in which log fluorescence intensity was read gated on propidium iodide to eliminate dead cells and a forward scatter threshold high enough to eliminate red blood cells. Five thousand cells were counted for each sample. Absolute cell numbers were calculated using the percentage of cells and the total splenocyte counts.
Spleen IgM AFC response to the T-dependent antigen sheep red blood cells (SRBC)
The primary immunoglobulin M (IgM) response to SRBCs was performed using a modified hemolytic plaque assay of Jerne and Nordin (Citation1963). Mice were sensitized intravenously with 7.5 × 107 SRBC (Rockland, Gilbertsville, PA) on Day 25 of exposure. On Day 29, animals were euthanized, and a single spleen cell suspension was prepared in EBSS and counted. An aliquot of cells was added to a test tube containing guinea pig complement, SRBC, and warm agar (46–48°C). After thoroughly mixing, the test tube mixture was plated in a petri dish, covered with a microscope coverslip, and incubated at 37°C for 3 hr. The plaques formed were counted using a Bellco plaque viewer at 6.5 × magnification. The data were expressed as specific activity (AFC/106 spleen cells) and total spleen activity (AFC/spleen).
Mixed leukocyte response (MLR) to DBA/2 spleen cells
Female B6C3F1 mice were exposed to SQV for 28 days, and single-cell suspension of splenocytes prepared under sterile conditions as described. Spleen cells were counted and adjusted to 1 × 106/mL, and were then added to a U-bottom microtiter plate at a concentration of 1 × 105 cells per well as responders. DBA/2 spleen cells were used as the allogeneic cells (stimulator) for the B6C3F1 mice. The stimulator cells were treated with mitomycin C to render them unable to proliferate. The ratio of stimulators to responders has previously been optimized to be 4:1; therefore stimulator cells were adjusted to 4 × 106/mL and added to wells containing responder cells at 100 μL/well. The cells were cultured for 5 days, during the last 18 hr in the presence of 1 µCi [3H]-thymidine (Perkin Elmer, Waltham, MA). The cells were collected with a cell harvester and counted in a LKB liquid scintillation counter. The incorporation of [3H]-thymidine into the proliferating cells was used as the endpoint of the assay, and the data were expressed as cpm/105 cells.
NK cell activity
The activity of NK cells was assayed as described by Reynolds and Herberman (Citation1981) with modification. Single-cell suspensions from individual spleens were prepared aseptically. Following centrifugation, the pellet was resuspended in RPMI 1640 supplemented with 10% FBS. The cell suspensions were adjusted to six concentrations: 2 × 107, 1 × 107, 5 × 106, 2.5 × 106, 1.25 × 106, and 6.25 × 105 cells/mL. The target cells (YAC-1 cells) were adjusted to a concentration of 107 cells/mL and then labeled by incubating with 200 µCi of 51Cr (as Na2CrO4; Perkin Elmer) for 90 min in a 37°C incubator with frequent agitation. Following the incubation, the cells were washed three times in balanced salt solution plus 25 mM HEPES, counted, and adjusted to 105 nucleated cells/mL. The targets were added to each well in a volume of 0.1 mL. The effector cells (0.1 mL) were added to each of four replicate wells of target cells at each effector concentration to obtain effector:target ratios of 200:1, 100:1, 50:1, 25:1, 12.5:1, and 6.25:1. The spontaneous release and the maximum release were determined by adding 0.1 mL of medium and Triton X-100 (0.1%) to each of 12 replicate wells containing the target cells, respectively. The plates were incubated for 4 hr at 37°C at 5% CO2. Following the incubation, the plates were centrifuged at 250 × g for 10 min, and 0.1 mL of the supernatant was removed from each well and counted. The mean percent cytotoxicity at each effector:target ratio was determined.
Cytotoxic T-lymphocyte (CTL) activity
The assay for CTL activity was performed as described (Germolec et al., Citation1988). The splenocytes from control and treated mice were washed once with EBSS. The cells were sensitized in vitro with mitomycin C-treated P815 mastocytoma cells at a responder-to-sensitizer ratio of 50:1 for 5 days at 37°C in 5% CO2. For the preparation of mitomycin C-treated P815 cells, cells were incubated in the dark with mitomycin C at a concentration of 50 μg per 2 × 107 cells for 30 min at 37°C. The cells were then washed four times. Following the sensitization phase, cultured spleen cells were harvested and resuspended in Eagle’s Minimal Essential Medium (EMEM, Hazelton, Lenexa, KS) for determination of CTL activity. [51Cr]-labeled P815 cells (2 × 104 cells/ 100 µL) were co-cultured in duplicate with 100 µL of graded numbers of splenic effector cells in U-bottom microtiter culture plates to yield a serial half dilution of effector:target ratios from 25:1 to 0.75:1. For the preparation of [51Cr]-labeled P815 cells, 15 × 106 cells were incubated with 500 µCi of [51Cr] for 60 min at 37°C with light vortex every 10–15 min followed by four washes. After a 4-hr incubation at 37°C and 5% CO2, the plates were centrifuged for 10 min at 250g. An aliquot (100 μL) of supernatant from each well was removed and counted in a LKB gamma spectrophotometer. Controls for spontaneous and maximum release were generated by culturing labeled target cells in the presence of either EMEM medium or 0.1% Triton X-100, respectively.
Host resistance studies
Host resistance to the B16F10 melanoma tumor
The assay for host resistance to the B16F10 melanoma tumor model was assayed as described by Murray et al. (Citation1985). One day following the last exposure to vehicle or SQV, mice (12 mice/group) were challenged with either 1 × 105 or 3 × 105 B16F10 melanoma cells intravenously. One day prior to sacrifice, the mice were pulsed intravenously with [125I]-UdR (Perkin Elmer). Eighteen days after tumor cell challenge, mice were sacrificed by CO2 inhalation. The lungs were removed, placed in Bouin’s solution (Sigma, St Louis, MO) and radioassayed (cpm/lung), and then counted for nodules (Nodules/lung). Anti-asialo GM1 (rabbit) antibody (Wako BioProducts, Richmond, VA) was used as a positive control. The positive animals were injected (intravenously) with 0.2 mL of a 1:10 dilution of the antibody 24 hr prior to the administration of B16F10 tumor cells.
Statistical analysis
To determine the type of analysis to be used, the Bartlett’s Test for homogeneity was conducted. Homogenous data were analyzed using a one-way analysis of variance, and the Dunnett’s t-test was used to determine differences between the control and experimental groups. Non-homogenous data were analyzed using a non-parametric analysis of variance and the Wilcoxon rank test to determine differences between the vehicle control group and exposure groups. The Jonckheere’s Test was used for trend analysis. A group was considered statistically significant from the control group if P ≤ 0.05.
Results
To determine the immunomodulatory effect of SQV, a tiered approach was employed. In Tier I, we examined the SQV-treated animals for alterations in hematology, body and organ weights, and IgM AFC responses and NK cell activity. In Tier II, we further investigated the effects of SQV on the expression of splenocyte surface markers using flow cytometric analysis, the CTL activity and MLR. Furthermore, the host resistance to B16F10 melanoma tumor was evaluated to determine the overall state of the immune system following SQV exposure.
Mice treated for 28 days with SQV at 300–1200 mg/kg/day tolerated SQV well as reflected by no changes in overall behavior and fur status (e.g., no ruffling), and the lack of drainage from body orifices and eyes. No effect was observed in terminal body weights, liver weights, spleen weights, lung weights, thymus weights or kidney weights of the animals treated with any dose levels of SQV as compared to the vehicle control (data not shown).
Hematological parameters were evaluated from blood obtained by retro-orbital bleed. There were no significant differences in erythrocytes, hemoglobin, hematocrit, MCV, MCH, and platelet numbers (), or leukocytes including lymphocytes, neutrophils, and eosinophils (data not shown), between animals treated with SQV and control animals. The decreasing trends for hemoglobin, hematocrit and MCV were not considered biologically meaningful because the maximal percentages of decreases were only 1.9, 2.9, and 0.4%, respectively. A statistically significant increase was observed in MCHC at the 600 mg/kg dose; however, this change was minimal and not dose-related, and thus, was not considered to be biologically relevant. Moreover, a statistically significant decrease in the percentage of reticulocytes was only observed at the 1200 mg/kg dose, the highest dose administered (). Although trend analysis showed a decrease in hemoglobin, hematocrit and MCV, the changes were minimal (). Cyclophosphamide (CPS) treatment produced significant changes in all the parameters except for the MCHC, and the numbers of platelets and leukocytes (), consistent with previous reports (Auttachoat et al., Citation2009).
Table 1. Effect of saquinavir on the hematology of female B6C3F1 mice.
To determine the effect of SQV on innate immune responses, NK cell activity was evaluated. Although the % cytotoxicity was slightly, albeit significantly, decreased at the 300 mg/kg and 1200 mg/kg dose levels at several lower effector-to-target ratios, there were no significant changes at the middle dose level (). As expected, anti-asialo GM1 treatment produced significant decreases in all the effector-to-target ratios.
Table 2. Effect of saquinavir on the activity of natural killer cells from female B6C3F1 mice.
Exposure to SQV enhanced the humoral immune response as reflected by the increased primary IgM AFC response to the T-dependent antigen, SRBC (). There was an increased response when the data were expressed as specific activity (IgM AFC/106 spleen cells) and total activity (IgM AFC/spleen), with the specific activity at the 1200 mg/kg dose reaching the level of statistical significance (). CPS treatment almost completely abolished the AFC responses.
Figure 1. SQV effects on IgM AFC response in female B6C3F1 mice. Animals were treated with 0.5% methylcellulose (VH) or SQV, and the number of IgM AFC was determined as described. The positive control animals received 50 mg/kg of cyclophosphamide (CPS) on the last 4 days of the exposure period by intraperitoneal injection. Data are presented as (A) AFC/106 spleen cells and (B) AFC/spleen. Values represent the mean (±SE) derived from eight animals. *P ≤ 0.05 or **P ≤ 0.01 vs. VH control.
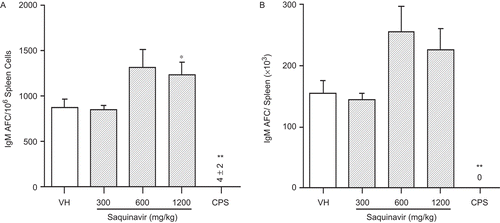
The percentages of specific leukocyte subpopulations were examined using flow cytometric analysis. The absolute cell numbers were calculated using the percentage of cells and the total splenocyte counts. When the data were expressed as absolute value (× 106), the number of T-cells (CD3+) was increased at the 1200 mg/kg dose (). Additionally, the number of splenic immature T-cells (CD4+CD8+ cells) also showed increases of 46% and 92% at the 600 and 1200 mg/kg dose levels, respectively (). No significant changes in other splenocyte subsets including B-cells (Ig+), CD4+ T-cells, CD8+ T-cells, NK cells (NK1.1+CD3−), and monocytes (Mac3+) were observed when the data were presented as either absolute value or percent value when compared to vehicle controls. CPS treatment produced significant changes in all the parameters except for the %CD4+CD8+.
Table 3. Effect of saquinavir on the number and percentage of differential splenocytes in female B6C3F1 mice.
Exposure to SQV did not affect the cell-mediated immune response as represented by negative findings in both the one-way MLR () and CTL assay (). In the MLR, neither the basal proliferation nor stimulated proliferation was changed by SQV treatment (). The CTL assay measures the final differentiation of T-cells to the cytotoxic effector cells. This occurs after the proliferative response takes place as evaluated by the MLR. Exposure to SQV appeared to suppress CTL activity at the 300 mg/kg dose level; however, there were no changes at the two higher dose levels (). CPS treatment produced significantly decreased activities in both assays.
Figure 2. SQV effects on (A) MLR and (B) CTL activity in female B6C3F1 mice. Animals were treated with 0.5% methylcellulose (VH) or SQV, and splenocytes were prepared and assayed as described. The positive control animals received 50 mg/kg of cyclophosphamide (CPS) on the last 4 days of the exposure period by intraperitoneal injection. cpm = counts per minute following incorporation of [3H]-thymidine. Values represent the mean (±SE) derived from eight animals. In panel B, an SE < 15% of value was not shown. *P ≤ 0.05 or **P ≤ 0.01 vs. VH control.
![Figure 2. SQV effects on (A) MLR and (B) CTL activity in female B6C3F1 mice. Animals were treated with 0.5% methylcellulose (VH) or SQV, and splenocytes were prepared and assayed as described. The positive control animals received 50 mg/kg of cyclophosphamide (CPS) on the last 4 days of the exposure period by intraperitoneal injection. cpm = counts per minute following incorporation of [3H]-thymidine. Values represent the mean (±SE) derived from eight animals. In panel B, an SE < 15% of value was not shown. *P ≤ 0.05 or **P ≤ 0.01 vs. VH control.](/cms/asset/74d7b0e5-f37d-4ba0-91b6-7f0c1dc1d095/iimt_a_495097_f0002_b.gif)
As SQV modulated a number of functional immune measures, host resistance assay of a tumor challenge model was utilized to further investigate its immunologic effects. Altered host resistance to tumor challenge is an in vivo manifestation of SQV-induced overall immunomodulation. Furthermore, anti-retroviral therapy using PIs has been shown to block various pathways of tumor growth, invasion, and metastasis (Sgadari et al., Citation2003). In the B16F10 melanoma model, no differences in lung tumor nodule formation were observed when compared to vehicle control animals following 28 days of SQV treatment with either a high tumor cell challenge level () or a low tumor cell challenge level (data not shown). When tumor burden was evaluated by measuring lung radioactivity (cpm/lung), no significant changes were observed as compared to vehicle control animals at low challenge level (data not shown). Although trend analysis showed an increase in the tumor burden at high challenge level, the overall response was not significant (). The positive control (anti-asialo GM1) animals showed increased tumor burden (both nodules/lung and cpm/lung) when compared to the vehicle controls at both challenge levels.
Figure 3. Effect of SQV on host resistance to B16F10 melanoma (A: nodules/lung; B: cpm/lung) in female B6C3F1 mice. Animals were treated with 0.5% methylcellulose (VH) or SQV, and then challenged with the pathogens as described. Rabbit anti-asialo GM1 (AAGM1) antibody was used as the positive control for B16F10 melanoma study, which was given to animals at 0.2 mL of 1:10 dilution by intravenous injection 24 hr prior to the assay. cpm = counts per minute of lung radioactivity following incorporation of [125I]-UdR. Values represent the mean (±SE) derived from N = 12 animals. The actual values obtained for the positive control groups were 475 ± 25 and 16.9 ± 3.5 for panels A and B, respectively. **P ≤ 0.01 vs. VH control.
![Figure 3. Effect of SQV on host resistance to B16F10 melanoma (A: nodules/lung; B: cpm/lung) in female B6C3F1 mice. Animals were treated with 0.5% methylcellulose (VH) or SQV, and then challenged with the pathogens as described. Rabbit anti-asialo GM1 (AAGM1) antibody was used as the positive control for B16F10 melanoma study, which was given to animals at 0.2 mL of 1:10 dilution by intravenous injection 24 hr prior to the assay. cpm = counts per minute of lung radioactivity following incorporation of [125I]-UdR. Values represent the mean (±SE) derived from N = 12 animals. The actual values obtained for the positive control groups were 475 ± 25 and 16.9 ± 3.5 for panels A and B, respectively. **P ≤ 0.01 vs. VH control.](/cms/asset/72fd4f41-6a62-4049-ba08-9d3da44f9b63/iimt_a_495097_f0003_b.gif)
Discussion
SQV is a PI commonly used in combination with reverse transcriptase inhibitors as part of HAART for individuals infected with HIV. HAART has significantly contributed to the reduction in mortality (Binley et al., Citation2000), and improved the quality of life of HIV-infected individuals (Hogg et al., Citation1997; Needham et al., Citation1998) with limited metabolic and cardiovascular toxicities (Lai et al., Citation2003; Schütt et al., Citation2004). However, these inhibited proteases have been categorized as aspartic proteinases, and cathepsins B, D, and L have been shown to participate in physiological processes such as antigen processing in antigen-presenting cells (Pacifici et al., Citation1997; Franzese et al., Citation2001). In addition, it was important to know if SQV could affect the immune responses in a host without HIV infection. Thus, the immunomodulatory effects of SQV in B6C3F1 mice were determined.
There is evidence that SQV treatment correlates with improved cell-mediated immunity in HIV patients (Angel et al., Citation1998; Lu and Andrieu, Citation2000; Matarrese et al., Citation2003). Chêne et al. (Citation1998) showed that treatment with PIs could increase the functionality of CD4+ T-cells, which may be partially responsible for a decreased incidence of opportunistic infections in HIV patients. In our studies, an increase in the number of T-cells at a SQV dose of 1200 mg/kg was observed. Moreover, SQV treatment produced a dose-related increase in the number of CD4+CD8+ T-cells, suggesting an enhanced T-cell development; however, SQV treatment in these studies did not affect cell-mediated immunity as represented by negative findings in both the CTL assay and MLR. Nonetheless, we could not rule out the possibility that a direct effect of SQV treatment on the function of activated T-cells in our mouse model is partially responsible for the enhanced humoral response as discussed below. Pacifici et al. (Citation1997) demonstrated an increase in interleukin-2 and interferon (IFN)-γ production in phytohaemagglutinin-stimulated mouse spleen cells following in vivo SQV exposure. An increase in IFN-γ production by activated T-cells has also been reported in human PBMC after in vitro SQV treatment (Comandini et al., Citation2001; Franzese et al., Citation2001). Furthermore, SQV treatment has been shown to inhibit activation-induced T-cell apoptosis, but not the apoptosis in resting cells (Matarrese et al., Citation2003). In future studies, the effect of SQV on T-cell function should be examined when the T-cells are in an activated state, e.g., in mice injected with either SRBC or pathogens (e.g., virus, bacteria).
In addition to cell-mediated immunity, the humoral immune response to HIV infection also plays an important role in determining disease progression (Sarmati et al., Citation1997). In this study, an enhancement of the humoral immune response was observed following SQV exposure in vivo. This observation is consistent with the report that a significant correlation was found between treatment with SQV and an increase in neutralizing antibody titer against sequential autologous HIV-1 isolates (Sarmati et al., Citation1997; Binley et al., Citation2000). Because the IgM AFC response requires competent antigen-presenting cells, T-helper cells, and B-cells in order to produce an effect, the enhancement in IgM AFC response could be related to the effects of SQV on one or more of these cell types. SQV modulation of the immune response is further supported by previously reported histopathological findings (Schapiro et al., Citation1997) that indicate that a prominent secondary immune reaction in the lymph nodes (Grade 1 histopathology) of HIV-positive patients appears to correlate with a more durable response to high dose SQV therapy.
Both NK cells and CD8+ T-cells have been shown to contribute to the host resistance against B16F10 melanoma (Parhar and Lala, Citation1987; Grundy et al., Citation2007). Exposure to SQV did not affect the host resistance to the B16F10 tumor in our study, which was consistent with the negative findings from the flow cytometric analysis of NK cells and CD8+ cells, as well as the cytotoxicity functional assays of NK cells and CTLs. There is increasing incidence of non-AIDS defining neoplasms in HIV-1+ individuals in the post HAART-era, but the pathogenicity has not yet been determined (Knysz et al., Citation2006). Our data do not suggest any associations between SQV exposure and an increased tumor incidence after HAART; this is further corroborated by the reports that SQV is a potent anti-angiogenic molecule (Sgadari et al., Citation2003) and a radiation sensitizer for non-HIV-associated cancers (Pajonk et al., Citation2002; Gupta et al., Citation2005).
Although anemia remains highly prevalent in HIV-infected patients, SQV treatment was not associated with this side effect of the antiviral therapy (Mildvan et al., Citation2007). Additionally, SQV use has not been reported to cause neutropenia (Sparano et al., Citation1998). These notions were further supported by our observations that exposure to SQV in female B6C3F1 mice had no biologically meaningful effects on various hematological parameters. However, it should be emphasized that these observations were made in adult patients and adult animals, as anemia and neutropenia may occur in infants following SQV treatment of their mothers (Zorrilla et al., Citation2007). Finally, it is worth mentioning that treatment with SQV did not affect the number of monocytes in our studies; this finding was in agreement with the observation that monocyte CD11b expression was not changed by SQV treatment (von Hentig et al., Citation2008).
In summary, the results presented here have demonstrated that treatment with SQV in vivo can affect the immune responses in female B6C3F1 mice. Stimulation of the humoral immune response following SQV treatment should benefit HIV-infected individuals. However, these observations do not explain all of the immune changes experienced by HIV patients following HAART. Therefore, some of these immunomodulatory effects might be due to either the virus-inhibitory effect of SQV or other components of HAART. Further study of SQV in combination with other PIs (e.g., ritonavir) on immune responses should shed more light on the mechanisms of action of these PIs in HIV patients.
Acknowledgements
The authors would like to thank D. L. Musgrove and R. D. Brown for their technical help, and Dr. Mark Cesta and Dr. June Dunnick for their comments and review of the manuscript.
Declaration of interest
This study was supported by the NIEHS contract NO1-ES-05454. Dr. Kimber L. White, Jr. is the owner of a company, ImmunoTox®, Inc., that conducts immunotoxicological studies under Good Laboratory Practices (GLP); however, none of the work presented here involved his company.
References
- Acosta, E.P., Zorrilla, C., Van Dyke, R., Bardeguez, A., Smith, E., Hughes, M., Huang, S., Pitt, J., Watts, H. and Mofenson, L. Pediatric AIDS Clinical Trials Group 386 Protocol Team. 2001. Pharmacokinetics of saquinavir-SGC in HIV-infected pregnant women. HIV Clin. Trials 2:460–465.
- Angel, J.B., Kumar, A., Parato, K., Filion, L.G., Diaz-Mitoma, F., Daftarian, P., Pham, B., Sun, E., Leonard, J.M. and Cameron, D.W. 1998. Improvement in cell-mediated immune function during potent anti-human immunodeficiency virus therapy with ritonavir plus saquinavir. J. Infect. Dis. 177:898–904.
- Autar, R.S., Boffito, M., Hassink, E., Wit, F.W., Ananworanich, J., Siangphoe, U., Pozniak, A., Cooper, D.A., Phanuphak, P., Lange, J.M., Ruxrungtham, K. and Burger, D.M. 2005. Interindividual variability of once-daily ritonavir boosted saquinavir pharmacokinetics in Thai and UK patients. J. Antimicrob. Chemother. 56:908–913.
- Auttachoat, W., Germolec, D.R., Collins, B.J., Luebke, R.W., White, K.L. Jr and Guo, T.L. 2009. Immunotoxicological profile of chloroform in female B6C3F1 mice when administered in drinking water. Drug Chem. Toxicol. 32:77–87.
- Binley, J.M., Trkola, A., Ketas, T., Schiller, D., Clas, B., Little, S., Richman, D., Hurley, A., Markowitz, M. and Moore, J.P. 2000. The effect of highly active anti-retroviral therapy on binding and neutralizing antibody responses to human immunodeficiency virus Type 1 infection. J. Infect. Dis. 182:945–949.
- Bucy, R.P., Hockett, R.D., Derdeyn, C.A., Saag, M.S., Squires, K., Sillers, M., Mitsuyasu, R.T. and Kilby, J.M. 1999. Initial increase in blood CD4(+) lymphocytes after HIV anti-retroviral therapy reflects redistribution from lymphoid tissues. J. Clin. Invest. 103:1391–1398.
- Chêne, G., Binquet, C., Moreau, J.F., Neau, D., Pellegrin, I., Malvy, D., Ceccaldi, J., Lacoste, D. and Dabis, F. 1998. Changes in CD4+ cell count and the risk of opportunistic infection or death after highly active anti-retroviral treatment. Groupe d’Epidémiologie Clinique du SIDA en Aquitaine. AIDS 12:2313–2320.
- Comandini, F.A., Lombardi, A., Saponiero, A. and Bonmassar, E. 2001. Saquinavir up-regulates telomerase activity in lymphocytes activated with monoclonal antibodies against CD3/CD28. J. Chemother. 13:384–388.
- Eagling, V.A., Back, D.J. and Barry, M.G. 1997. Differential inhibition of cytochrome P450 isoforms by the protease inhibitors, ritonavir, saquinavir and indinavir. Br. J. Clin. Pharmacol. 44:190–194.
- Ernest, C.S. 2nd, Hall, S.D. and Jones, D.R. 2005. Mechanism-based inactivation of CYP3A by HIV protease inhibitors. J. Pharmacol. Exp. Ther. 312:583–591.
- Franzese, O., Lombardi, A., Comandini, A., Cannavò, E., Testorelli, C., Cirello, I. and Bonmassar, E. 2001. Effect of Saquinavir on proliferation and telomerase activity of human peripheral blood mononuclear cells. Life Sci. 69:1509–1520.
- Germolec, D.R., Maronpot, R.R., Ackermann, M.F., Vore, S.J., Dittrich, K., Rosenthal, G.J. and Luster, M.I. 1988. Lack of a relationship between immune function and chemically induced hepatocarcinogenesis in B6C3F1 mice. Cancer Immunol. Immunother. 27:121–127.
- Gruber, A., Wheat, J.C., Kuhen, K.L., Looney, D.J. and Wong-Staal, F. 2001. Differential effects of HIV-1 protease inhibitors on dendritic cell immunophenotype and function. J. Biol. Chem. 276:47840–47843.
- Grundy, M.A., Zhang, T. and Sentman, C.L. 2007. NK cells rapidly remove B16F10 tumor cells in a perforin and interferon-gamma independent manner in vivo. Cancer Immunol. Immunother. 56:1153–1161.
- Gupta, A.K., Cerniglia, G.J., Mick, R., McKenna, W.G. and Muschel, R.J. 2005. HIV protease inhibitors block Akt signaling and radiosensitize tumor cells both in vitro and in vivo. Cancer Res. 65:8256–8265.
- Hogg, R.S., O’Shaughnessy, M.V., Gataric, N., Yip, B., Craib, K., Schechter, M.T. and Montaner, J.S. 1997. Decline in deaths from AIDS due to new anti-retroviral. Lancet 349:1294.
- Hsu, A., Granneman, G.R., Cao, G., Carothers, L., el-Shourbagy, T., Baroldi, P., Erdman, K., Brown, F., Sun, E. and Leonard, J.M. 1998. Pharmacokinetic interactions between two human immunodeficiency virus protease inhibitors, ritonavir and saquinavir. Clin. Pharmacol. Ther. 63:453–464.
- Jerne, N.K. and Nordin, A.A. 1963. Plaque formation in agar by single antibody-producing cells. Science 140:405.
- Knysz, B., Zalewska, M., Rzeszutko, M. and Gladysz, A. 2006. Lung cancer as an immune reconstitution disease in an HIV-1 positive man receiving HAART. Postepy. Hig. Med. Dosw. (Online). 60:181–183.
- Lai, S., Lai, H., Celentano, D.D., Vlahov, D., Ren, S., Margolick, J., Lima, J.A. and Bartlett, J.G. 2003. Factors associated with accelerated atherosclerosis in HIV-1-infected persons treated with protease inhibitors. AIDS Patient Care STDS 17:211–219.
- Lu, W. and Andrieu, J.M. 2000. HIV protease inhibitors restore impaired T-cell proliferative response in vivo and in vitro: A viral-suppression-independent mechanism. Blood 96:250–258.
- Matarrese, P., Gambardella, L., Cassone, A., Vella, S., Cauda, R. and Malorni, W. 2003. Mitochondrial membrane hyperpolarization hijacks activated T-lymphocytes toward the apoptotic-prone phenotype: Homeostatic mechanisms of HIV protease inhibitors. J. Immunol. 170:6006–6015.
- Mildvan, D., Creagh, T. and Leitz, G.; Anemia Prevalence Study Group. 2007. Prevalence of anemia and correlation with biomarkers and specific anti-retroviral regimens in 9690 human-immunodeficiency-virus-infected patients: Findings of the Anemia Prevalence Study. Curr. Med. Res. Opin. 23:343–355.
- Murray, M.J., Kerkvliet, N.I., Ward, E.C., and Dean, J.H. 1985. Models for the evaluation of tumor resistance following chemical or drug exposure. In: Immunotoxicology and Immunopharmacology (Dean, J.H., Luster, M.I., Munson, A.E., and Amos, H., Eds.), New York: Raven Press, pp. 113–122.
- Needham, D.M., Hogg, R.S., Yip, B., O’Shaughnessy, M., Schechter, M.T. and Montaner, J.S. 1998. The impact of anti-retroviral therapy on AIDS survival observed in a province-wide drug treatment programme. Int. J. STD AIDS 9:370–372.
- Pacifici, R., Di Carlo, S., Bacosi, A., Pichini, S. and Zuccaro, P. 1997. Cytokine production in saquinavir treated mice. Int. J. Immunopharmacol. 19:243–248.
- Pajonk, F., Himmelsbach, J., Riess, K., Sommer, A. and McBride, W.H. 2002. The human immunodeficiency virus (HIV)-1 protease inhibitor saquinavir inhibits proteasome function and causes apoptosis and radiosensitization in non-HIV-associated human cancer cells. Cancer Res. 62:5230–5235.
- Parhar, R.S. and Lala, P.K. 1987. Amelioration of B16F10 melanoma lung metastasis in mice by a combination therapy with indomethacin and interleukin 2. J. Exp. Med. 165:14–28.
- Perry, C.M. and Noble, S. 1998. Saquinavir soft-gel capsule formulation. A review of its use in patients with HIV infection Drugs 55:461–486.
- Reynolds, C.W. and Herberman, R.B. 1981. In vitro augmentation of rat natural killer (NK) cell activity. J. Immunol. 126:1581–1585.
- Sarmati, L., Nicastri, E., el-Sawaf, G., Ventura, L., Salanitro, A., Ercoli, L., Vella, S. and Andreoni, M. 1997. Increase in neutralizing antibody titer against sequential autologous HIV-1 isolates after 16 weeks saquinavir (Invirase) treatment. J. Med. Virol. 53:313–318.
- Schapiro, J.M., Kamel, O.W., Winters, M.A., Vierra, M., Efron, B., and Merigan, T.C. 1997. Lymph node histopathology in HIV-infected patients correlates with duration of response to anti-retroviral therapy. Conf. Retrovir. Oppor. Infect. 4th 1997, Washington DC. Jan 22-26; 4th:164 (Abstract #538).
- Schütt, M., Zhou, J., Meier, M. and Klein, H.H. 2004. Long-term effects of HIV-1 protease inhibitors on insulin secretion and insulin signaling in INS-1 beta cells. J. Endocrinol. 183:445–454.
- Sgadari, C., Monini, P., Barillari, G. and Ensoli, B. 2003. Use of HIV protease inhibitors to block Kaposi’s sarcoma and tumour growth. Lancet Oncol. 4:537–547.
- Sparano, J.A., Wiernik, P.H., Hu, X., Sarta, C., Henry, D.H. and Ratech, H. 1998. Saquinavir enhances the mucosal toxicity of infusional cyclophosphamide, doxorubicin, and etoposide in patients with HIV-associated non-Hodgkin’s lymphoma. Med. Oncol. 15:50–57.
- Viora, M., Di Genova, G., Quaranta, M.G., Boirivant, M. and Camponeschi, B. 1998. Lack of immunotoxicity of saquinavir (Ro 31-8959) used alone or in double or triple combination with AZT and ddC. J. Clin. Immunol. 18:346–354.
- von Hentig, N., Förster, A.K., Kuczka, K., Klinkhardt, U., Klauke, S., Gute, P., Staszewski, S., Harder, S. and Graff, J. 2008. Platelet-leucocyte adhesion markers before and after the initiation of anti-retroviral therapy with HIV protease inhibitors. J. Antimicrob. Chemother. 62:1118–1121.
- Washington, C.B., Wiltshire, H.R., Man, M., Moy, T., Harris, S.R., Worth, E., Weigl, P., Liang, Z., Hall, D., Marriott, L. and Blaschke, T.F. 2000. The disposition of saquinavir in normal and P-glycoprotein deficient mice, rats, and in cultured cells. Drug Metab. Dispos. 28:1058–1062.
- Winston, A., Mallon, P.W., Satchell, C., MacRae, K., Williams, K.M., Schutz, M., Law, M., Cooper, D.A. and Emery, S. 2007. The safety, efficacy, and pharmacokinetic profile of a switch in anti-retroviral therapy to saquinavir, ritonavir, and atazanavir alone for 48 weeks and a switch in the saquinavir formulation. Clin. Infect. Dis. 44:1475–1483.
- Zorrilla, C.D., Van Dyke, R., Bardeguez, A., Acosta, E.P., Smith, B., Hughes, M.D., Huang, S., Watts, D.H., Heckman, B., Jiménez, E., McSherry, G. and Mofenson, L. 2007. Clinical response and tolerability to and safety of saquinavir with low-dose ritonavir in human immunodeficiency virus Type 1-infected mothers and their infants. Antimicrob. Agents Chemother. 51:2208–2210.