Abstract
Levamisole has been employed as an immunomodulatory agent in conjunction with 5-fluorouracil in the treatment of colon cancer relapse. At high doses, levamisole has been shown to have both anti-cancer and immunosuppressive activities. In vitro, levamisole has been shown to potentiate the anti-proliferative effect of 5-fluorouracil in several types of tumor cell lines; however, its mechanism of cytotoxic action and its molecular targets in cells remains to be elucidated. Here, the effect of levamisole on the proliferative response of the human multiple myeloma cell lines RPMI 8226 and U266B1 was studied in vitro. Treatment of both lines with varying concentrations of levamisole for 48 and 72 h in culture resulted in a significant inhibition of proliferation (unstimulated) in a dose-dependent manner, as assessed by an 3-[(4,5-dimethylthiazole-2-yl)-2,5-diphenyltetrazolium bromide dye assay. Furthermore, measurements of cell viability (using a trypan blue dye exclusion assay) clearly showed that the levamisole was cytotoxic. The preliminary evaluation of the mechanism of this cytotoxic effect revealed that this drug induced apoptosis in the myeloma cells, as evidenced by increases in the levels of DNA fragmentation, release of cytochrome c into the cytoplasm, and the activation of caspase-3 activity in the cells. The results of these studies strongly suggest that levamisole could be a potent anti-myeloma agent and might be considered in the treatment of multiple myeloma in the future.
Introduction
Levamisole, originally used as an anti-helminthic drug, has also been employed as an immunomodulatory agent in conjunction with 5-fluorouracil (as an adjuvant) in the treatment of colon cancer relapse (Moertel et al., Citation1995; Yip et al., Citation2000). At high concentrations, levamisole has been shown to have both anti-cancer and immunosuppressive activities. In vitro, levamisole has been demonstrated to potentiate the anti-proliferative effect of 5-fluorouracil in several types of tumor cell lines (de Waard et al., Citation1998). However, the mechanism of this drug’s cytotoxic action and its molecular targets in multiple myeloma cells still remains to be elucidated.
One possible mechanism for this cytotoxic property of levamisole could be an ability to induce apoptosis. An earlier study had shown that levamisole induced apoptosis in cultured vascular endothelial cells (Artwohl et al., Citation2000). Studies from our own laboratory have shown that levamisole dose-dependently inhibited the proliferative response and alkaline phosphatase (APase) activity of lipopolysaccharide-stimulated murine B-lymphocytes (Padmaja and Ramanadham, Citation1998). Furthermore, the presence of an antibody to APase inhibited the proliferative response in mitogen-induced B-lymphocytes, suggesting a role for APase in the cell cycle (Marty and Feldbush, Citation1993, Padmaja, Citation1999).
High levels of APase activity have been observed in different types of malignant cells and in serum samples of cancer patients (Millán Citation1992; Millán and Fishman, Citation1995). In addition, the tumorogenicity of cell lines has been correlated with high levels of APase expression (Latham and Stanbridge, Citation1990). The enhancement of APase activity could be due to a functional involvement of APase isoenzymes in tumorigenesis. As such, APase might be a crucial factor involved in the etiology of certain cancers. Mammalian APase activity in liver, bone, and/or kidney cell types has been demonstrated to be uncompetitively inhibited by levamisole (Borgers Citation1973; De Broe and Van Hoof, Citation1991). Levamisole has also been shown to inhibit the proliferation of cultured bone cells, with concomitant inhibition in APase activity (Garba and Marie, Citation1986).
Preliminary studies from our laboratory have indicated that APase is constitutively expressed in malignant human B-lymphocyte cell lines, but in not in malignant T-lymphocyte lines (Padmaja, Citation1999). Hence, in the present study, we sought to investigate the effect of levamisole on the proliferative (unstimulated) response of the myeloma cell lines RPMI 8226 and U266B1 in vitro, using APase as a purported molecular target. The effect of levamisole in terms of the growth, viability, and proliferation of these myeloma lines was evaluated in vitro, and an initial definition of its cytotoxic mechanism (in terms of induction of apoptosis) was deduced.
Materials and methods
Cell lines and culture conditions
U266B1 and RPMI 8226 cell lines were obtained from the National Center for Cell Science, Pune, India. Each line was maintained in RPMI-1640 tissue culture medium (Sigma-Aldrich, Bangalore, India) supplemented with L-glutamine, and 10% heat-inactivated fetal calf serum (Biological Industries, S.A., Kibbutz Beit Haemek, Israel) in 25 cm2 tissue culture flasks. All cells were incubated at 37°C in a humidified atmosphere of 95% air and 5% CO2.
Viability assay
Cell viability was assessed by exclusion of trypan blue dye. For each evaluation, a small volume of the cell suspension was diluted appropriately in trypan blue solution (0.2% [w/v] in 0.9% NaCl). A minimum of 300 cells was then counted using a hemocytometer and the percentage of viable cells was calculated.
MTT assay
Unstimulated cell proliferative capacity—which can be used as an indirect measure of any potential cytotoxic effect of levimasole (or any test agent)—was determined using a colorimetric assay that employed 3-[(4,5-dimethylthiazole-2-yl)-2,5-diphenyltetrazolium bromide dye (MTT), as originally described by Mosmann (Citation1983). Briefly, the myeloma cells were seeded into the wells of 96-well tissue culture plates (Orange Scientific, Braine-l’Allued, Belgium) at a concentration of 0.05 × 106 in 200 µl culture medium supplemented with fetal calf serum. After allowing 4 h of pre-incubation, the cells were treated with varying concentrations of levamisole for specified time periods. At the end of each defined incubation period, the microplate was centrifuged at 250 × g for 10 min and the medium was removed. To each well, 100 µl of phosphate-buffered saline ([pH 7.40] supplemented with glucose and MgCl2) was added. An aliquot (20 µl) of a stock MTT solution (5 mg /ml; Himedia, Mumbai, India) was then added to each well and the plate incubated for 4 h at 37°C. At the end of this incubation period, 100 µl of acidified SDS (sodium dodecyl sulfate; 20% [w/v]) was added and the plate incubated overnight to solubilize the insoluble purple formazan product formed by reduction of MTT by mitochondrial dehydrogenases. The absorbance in each well was then measured at 570 and 630 nm using a Multiscan-EX ELISA plate reader (Labsystems, Oy, Vantaa, Finland) in a dual-wavelength mode. The differential absorbance of the product (i.e., A570 - A630 nm) was taken to be directly proportional to the unstimulated proliferative response of the cells in the well.
APase activity assay
Triplicate samples of myeloma cells (0.05 × 106/assay) were cultured with various concentrations of levamisole in 200 µl of complete medium. APase activity was determined using p-nitrophenylphosphate as substrate (p-NPP). Briefly, cells were dispensed into microtitre plate wells and centrifuged at 450 × g for 10 min at 4°C. The cells were then suspended in 0.9% NaCl and the centrifugation step was repeated. To the pellet was added 180 µl of 1 mg p-NPP/ml in 0.1 M bicarbonate buffer (with 2 mM MgCl2). Incubation was then carried out at 37°C in a humified incubator. After 30 min, the reaction was stopped by the addition of 20 µl of 1N NaOH and the absorbance was measured at 405 nm using an Multiscan-EX ELISA plate reader. The amount of p-NP released was extrapolated from a standard curve prepared in parallel. All results were then expressed as nmole p-NP released/culture.
Assay of caspase-3 activity
Caspase-3 activity was measured in cell extracts by lysing cells treated for 48 h with levamisole (1.0 mM or 2.5 mM), vincristine (1.0 µM), or vehicle (untreated) in a buffer containing 20 mM Tris-HCl (pH 7.8), 1 mM MgCl2, 1 mM dithiothreitol (DTT), 80 mM KCl, 10 µg pepstatin/ml, 10 µg leupeptin/ml, and 1 mM phenylmethanesulfonylfluoride. After the protein concentration was determined using the Lowry method, caspase-3 activity in each sample was determined by incubating cell lysate (bearing 150 µg protein) with 10 µg of the fluorogenic caspase-3 susbstrate (Ac(N-acetyl)-DEVD-AFC, 7-amino-4-trifluoromethyl-coumarin, referred to hereafter as AC-DEVD-AFC) in assay buffer (20 mM HEPES buffer [pH 7.5], 10 mM DTT, 1 mM EDTA, 0.1% Triton X-100, and 10% sucrose) for 1 h at 37°C. A control reaction was established in parallel by the addition of 10 µg of Ac-DEVD-CHO caspase-3 specific inhibitor, followed by incubation at 37°C for 30 min prior to the addition of 10 µg of AC-DEVD-AFC substrate. Fluorescence of the product was then measured in a Fluoromax-3 spectrofluorometer (Horiba Jobin Yvon, Edison, NJ) Specifically, Ac-DEVD-AFC hydrolysis was monitored by assessing the fluorescence emission of the released AFC product (excitation = 400 nm; emission = 500 nm) as described by Bhuyan et al. (Citation2001).
Assay of cyt c
For determining cytosolic cytochrome c (cyt c) concentration, cell extracts were prepared by lysing untreated and levamisole-treated U266B1 cells as described above. Cytosolic protein (50 µg) from each sample was then electrophoresed over a 10% polyacrylamide gel containing SDS. Thereafter, all proteins were transferred from the gel to a nitrocellulose membrane and subsequently probed with monoclonal anti-human cyt c antibody conjugated to APase (Bender Medsystems, Vienna, Austria). The presence of the target protein on the membrane was visualized via the use of a BCIP-NBT (5-bromo-4-chloro-3-indolyl phosphate + nitroblue tetrazolium) substrate for the conjugate enzyme, as described by Liu et al. (Citation1996).
DNA fragmentation analysis
Briefly, myeloma cells (1 × 106) that were treated with 1.0 mM levamisole for 48 and 72 h were centrifuged at 4000 × g for 5 min at 4°C. After the supernatant was discarded, the cells were resuspended (on ice) in 20 µl of lysis buffer (50 mM Tris-HCl [pH 8.0], 10 mM EDTA, 0.5% (w/v) sodium lauryl sarcosine, and 0.5 mg proteinase K/ml). The lysate was then incubated at 55°C for 1 h, after which all cell debris was pelleted and lysis buffer containing 0.5 mg RNase A/ml was added to the supernatant. After a further incubation for 1 h, the sample was briefly centrifuged at 15000 × g to pellet any remaining cell debris. The supernatant was then collected, heated to 70°C for a few minutes, and mixed with 10 µl of loading buffer. The sample was then placed into well(s) of a 2% agarose gel containing 0.5 µg/ml ethidium bromide, and electrophoresis carried out at 40 V for 2 h. After visualization under a UV light in a trans-illuminator, the resultant gel pattern was photographed.
Statistical analysis
The results are presented as the mean ± SEM for all variables examined. A Student’s t-test was used to determine the significance of any difference in values between given groups. Any difference was significant at p < 0.05.
Results
Effect of levamisole on viability
As shown in , the addition of levamisole significantly inhibited the viability of RPMI 8226 and U266B1 myeloma cell lines in a dose-dependent manner, as assessed by trypan blue dye exclusion. The cell viability was not affected at 0.5 mM concentration of levamisole. However, the viability was significantly decreased with 1.0 and 2.5 mM levamisole concentration. Treatment of the RPMI 8226 cells with 1 mM levamisole for 48 h resulted in a viability that was significantly decreased to ≈75% as compared to that of the control cells. At 72 h, the viability decreased further to 45% of control levels. With 2.5 mM levamsiole, the viability of these cells was decreased to 46% and 32% (of control values) at 48 and 72 h, respectively. Strikingly similar results were obtained with U266B1 cells. In general, these results indicate that levamisole is cytotoxic to both types of myeloma cells above 1.0 mM concentration.
Figure 1. Effect of levamisole on viability of multiple myeloma cell lines. Cells were incubated with the indicated concentrations of levamisole for 48 and 72 h in complete medium. (A) RPMI 8226 (B) U266B1. Cell viability was determined via a trypan blue dye exclusion assay. Each value represents the mean ± SEM of three expriments. *p < 0.05, levamisole-treated vs. no addition (control).
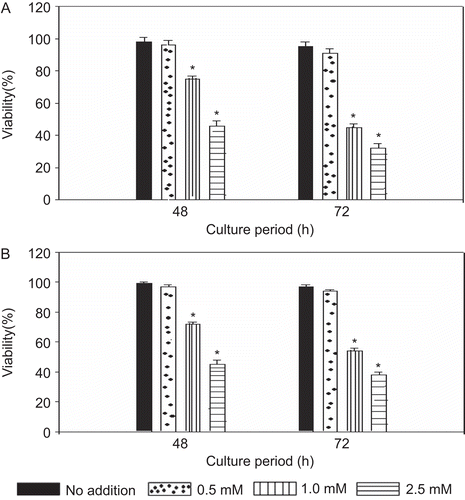
Cytotoxic effect of levamisole
The cytotoxic effect of levamisole on these two particular multiple myeloma cell lines was determined using an MTT assay. As with the first assay of cell viability above, there was again a significant dose-dependent cytotoxic effect from levamisole on both of the cell lines (). With the RPMI 8226 cells, the unstimulated cell proliferative capacity—an indirect measure of any potential cytotoxic effect of the levimasole—at 48 h decreased significantly by 33, 57, and 70% as compared to the control value with exposure to 0.5, 1.0, or 2.5 mM levamisole, respectively. After 72 h incubation, the proliferative responses, respectively, decreased to 62, 73, and 97% of control levels. Once again, similar results were noted with the U266B1 cells. Upon treatment with levamisole for 48 and 72 h, the unstimulated proliferative response was significantly decreased compared to the controls. This apparent time-related effect impacted the estimation of the IC50 value in these studies. For examples, the IC50 of the levamisole with the both the cell lines approximated 2.5 mM at 48 h but decreased to 1 mM after 72 h.
Figure 2. Effect of levamisole on the proliferation of myeloma cells. Levamisole was added at the indicated concentrations and the cells were cultured in complete medium. Cell proliferation was determined by an MTT assay at 48 and 72 h. (A) RPMI 8226 (B) U266B1. Each value represents the mean ± SEM of three experiments. *p < 0.05, levamisole-treated vs. no addition (control).
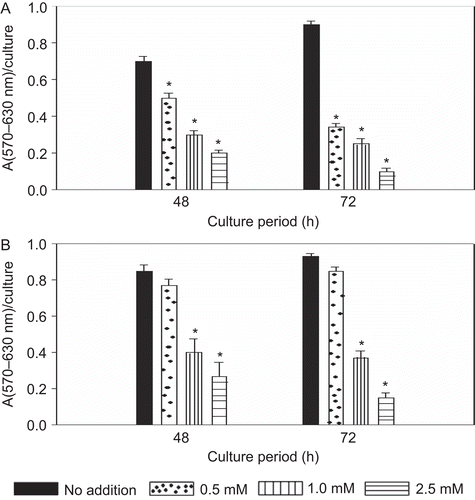
Effect of levamisole on APase activity in cell lines
Both myeloma cell lines (RPMI 8226 and U266B1) constitutively express APase activity. In the presence of levamisole, the APase activity was inhibited in a dose-dependent manner (). In RPMI 8226 cells, upon treatment with levamisole for 48 h, the APase activity was significantly inhibited (as compared to untreated cell levels) by 30% with the 1.0 mM dose and 70% with the 2.5 mM dose. The more prolonged exposure (i.e., 72 h) resulted in an APase activity that was inhibited by > 90% with all concentration of levamisole. The APase activity in the U266B1 cells was also found to be inhibited in a manner similar to that in the RPMI 8226 line.
Figure 3. Effect of levamisole on APase activity of myeloma cells. Cells were incubated with 0.5, 1.0, or 2.5 mM levamisole and APase activity was determined as described in the Materials and methods. (A) RPMI 8226, (B) U266B1, (C) no levamisole addition. Each value represents the mean ± SEM of three experiments. *p < 0.05, levamisole-treated vs. no addition (control).
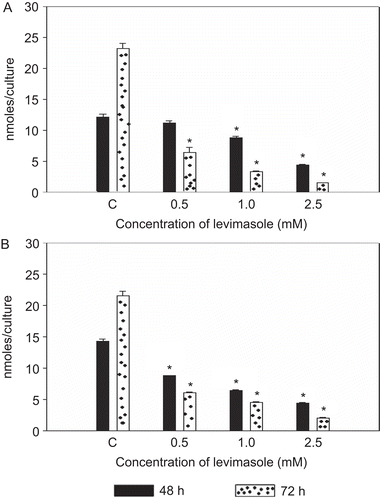
Assay of caspase activity
The caspase-3 activity of levamisole-treated U266 cells was measured using a fluorogenic substrate. In order to observe the specificity of the assay, DEVD-CHO (an inhibitor of caspase) was added prior to the addition of the substrate. Cells exposed to UV-B radiation—an agent known to induce apoptosis—were used as a positive control. The enhancement of caspase activity was completely abolished in presence of the specific DEVD-CHO inhibitor. The caspase activity was increased significantly (above backgound) after treatment with levamisole for 24 h as compared to the untreated cells. However, there was no notable difference in responses when the drug dose was increased from 1.0 to 2.5 mM. As expected, the caspase activity within the UV-B-exposed cell extracts was the highest of all the measures; specifically, the activity in these cells was found to be 3-fold higher than that observed in any of the levamisole-treated cells ().
Figure 4. Effect of levamisole on caspase-3 activity in myeloma cells. U266B1 cells were treated with 1.0 or 2.5 mM levamisole for 24 h and caspase-3 activity was then determined as described in the Materials and methods. (1) Blank, (2) untreated cells, (3) DEVD-CHO, (4) levamisole–1.0 mM, (5) levamisole–2.5 mM, (6) UV-B (60 s).
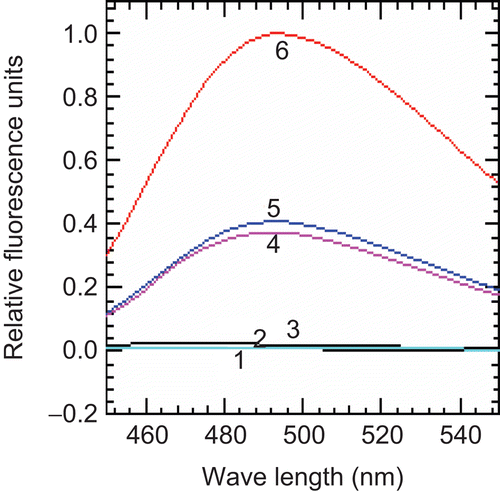
Identification of cytosolic cyt c
U266B1 cells were treated with 0.5 and 1.0 mM levamisole for 24 h and the cytosolic extracts were assayed for cyt c levels. cyt c was identified by western blotting of cell lysates using anti-human cyt c antibody. As indicated in , there was an increase in the level of cyt c seen with the levamisole-treated cells as compared to in the untreated cells. β-Actin was used to confirm that equal amounts of protein loading of the samples had in fact been carried out in these analyses.
DNA fragmentation assay
In order to examine the potential mechanism of cell death that might have been induced by levamisole, a DNA fragmentation assay was performed. DNA was isolated from the myeloma cell lines treated with 1.0 mM levamisole or 1.0 µM vincristine for 48 and 72 h. The results presented in show that DNA fragmentation indicative of apoptotic cell death—was readily observed in levamisole-treated RPMI 8226 and U266B1 cells, respectively. Under identical conditions of cell culture, the DNA from untreated cells remained intact. Vincristine, an anti-myeloma drug used as a positive control, induced DNA fragmentation with a ladder-like pattern.
Figure 6. Agarose gel electrophoresis of DNA extracted from myeloma cells treated with levamisole. (A) RPMI 8226 and (B) U266B1. Electrophoresis and visualization of the gels was carried out as described in the Materials and methods. Lane 1: DNA ladder (0.1–1.0 Kbp); Lane 2: no treatment, 48 h, Lane 3: no treatment, 72 h, Lanes 4 and 5: cells treated with 1.0 mM levamisole for 48 h and 72 h, respectively; Lanes 6 and 7: cells treated with vincristine (1 µM) for 48 and 72 h, respectively.
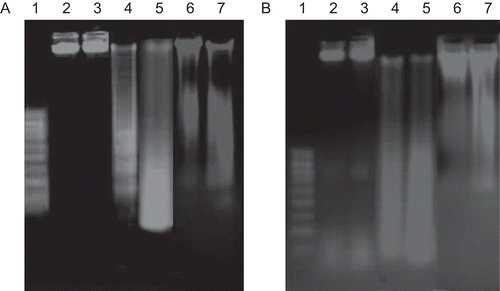
Discussion
In an experimental rat model of colonic inflammation, significant increase in APase activity was observed mainly in the epithelial cells and leukocyte population. Inhibition of enzyme activity using levamisole or a monoclonal antibody to APase resulted in significant protection from the inflammation (Sanchez de Medina et al., Citation2004). These observations strongly suggested that levamisole could be used as an inhibitor of APase in vivo.
Earlier studies on mitogen-stimulated B-lymphocytes have indicated that the APase activity may be involved in the proliferative response. Levamisole was found to be good inhibitor of tissue non-specific APase activity. This drug was also found to have anti-cancer properties; levamisole has been successfully used as an adjuvant with 5-fluorouracil in the post-surgical treatment of colorectal carcinoma (Amery, Citation1977; Cafiero et al., Citation2000).
Levamisole, an uncompetitive inhibitor, has also been shown to significantly inhibit the proliferation of myeloma cells. APase isoenzymes have been shown to be expressed by a variety of human cancer cell lines and also have been proposed as a marker of malignancy in colorectal cancer (Herz, Citation1985; Wei et al., Citation1993). This enzyme has also been proposed to be involved in the induction and maintenance of malignant states. In the present studies, APase activity has been shown to be present in appreciable amounts in the myeloma cell lines.
We have used levamisole in vitro to see its effect on myeloma cell lines. The results here indicated that viability of both cell lines tested was unaffected at a 0.5 mM concentration of levamisole. Levamisole was used at various concentrations to also evaluate its anti-proliferative activity. In addition to inhibiting the proliferation of the test cell lines, levamisole-induced cytotoxicity at ≥ 0.5 mM in RPMI 8226 cells and at ≥ 1.0 mM in U266B1 cells, respectively. This difference in effect indicates a differential sensitivity of the cell lines to levamisole. The possible mechanism for the cytotoxic effect of levamisole at higher concentrations, i.e., ≥ 1.0 mM, could be through the induction of apoptosis; indeed, an earlier study showed that levamisole had an apoptotic effect in vascular endothelial cells (Artwohl et al., Citation2000). Myeloma cells treated with levamisole were evaluated for apoptosis using caspase-3 activity, cytosolic cyt c release from mitochondria and DNA fragmentation analysis. The fragmentation analysis revealed a ladder pattern typical of cells undergoing apoptosis; the analyses of caspase-3 activity and cyt c release also indicated that levamisole-induced cell death followed the mitochondrial mediated pathway.
The observations reported here indicate that levamisole exerts a potent anti-proliferative activity and cytotoxic effect (by apoptosis) on myeloma cells. Thus, inhibition of APase could potentially have an important application in the management of pathological conditions associated with multiple myeloma. In the treatment of colorectal cancer relapse cases, levamisole is not used alone but as an adjuvant along with anti-cancer drugs like 5-fluorouracil. It is proposed that the mechanism of action of levamisole in colon cancer should be distinct from that in myeloma cells. We believe this is because: (A) the isozyme of APase expressed in myeloma cells is of tissue non-specific type, whereas either an intestinal or placental isozyme expression is present in colon cancer cells (Gum et al., Citation1990; Matsumoto et al., Citation1990); and, (B) levamisole is a potent inhibitor of the tissue non-specific isozyme as compared to the other two tissue-specific isozymes (Millán, Citation2006). Apart from this distinction, it is important to remember that levamisole also has a number of other properties that may also be involved in its anti-proliferative activity, such as its ability to exert potassium channel inhibition, induction of apoptosis, inhibition of nuclear factor-κB, etc. (Kim et al., Citation1999; Artwohl et al., Citation2000; Liu et al., Citation2004). Nevertheless, the fact that APase is expressed in human B-cell malignancies like myeloma and that levamisole has a significant inhibitory effect on myeloma cell proliferation/viability suggests that APase could constitute a relevant target for drug therapy.
Acknowledgements
We thank NCCS, Pune for cell lines.
Declaration of interest
Ms B.Nageshwari is supported by a fellowship from CSIR, India.
References
- Amery, W. K. 1977. Levamisole as an immunotherapeutic agent in the treatment of cancer. World J. Surg. 1:597–604.
- Artwohl, M., Hölzenbein, T., Wagner, L., Freudenthaler, A., Waldhäusl, W. and Baumgartner-Parzer, S. M. 2000. Levamisole-induced apoptosis in cultured vascular endothelial cells. Br. J. Pharmacol. 131:1577–1583.
- Bhuyan, A. K., Varshney, A. and Mathew, M. K. 2001. Resting membrane potential as a marker of apoptosis: Studies on Xenopus oocytes microinjected with cytochrome c. Cell Death Differ. 8:63–69.
- Borgers, M. 1973. The cytochemical application of new potent inhibitors of alkaline phosphatases. J. Histochem. Cytochem. 21:812–824.
- Cafiero, F., Gipponi, M., Peressini, A., Bertoglio, S. and Lionetto, R. 2000. Preliminary analysis of a randomized clinical trial of adjuvant postoperative RT vs. postoperative RT plus 5-FU and levamisole in patients with TNM Stage II-III resectable rectal cancer. J. Surg. Oncol. 75:80–88.
- De Broe, M. E. and Van Hoof, V. O. 1991. Multiple forms of alkaline phosphatase in plasma of hemodialysis patients. Clin. Chem. 37:783–784.
- de Waard, J. W., de Man, B. M., Wobbes, T., van der Linden, C. J. and Hendriks, T. 1998. Inhibition of fibroblast collagen synthesis and proliferation by levamisole and 5-fluorouracil. Eur. J. Cancer. 34:162–167.
- Garba, M. T. and Marie, P. J. 1986. Alkaline phosphatase inhibition by levamisole prevents 1,25-dihydroxyvitamin D3-stimulated bone mineralization in the mouse. Calcif. Tissue Int. 38:296–302.
- Gum, J. R., Hicks, J. W., Sack, T. L. and Kim, Y. S. 1990. Molecular cloning of complementary DNAs encoding alkaline phosphatase in human colon cancer cells. Cancer Res. 50:1085–1091.
- Herz, F. 1985. Alkaline phosphatase isozymes in cultured human cancer cells. Experientia 41:1357–1361.
- Kim, J. A., Kang, Y. S., Lee, S. H., Lee, E. H., Yoo, B. H. and Lee, Y. S. 1999. Glibenclamide induces apoptosis through inhibition of cystic fibrosis transmembrane conductance regulator (CFTR) Cl− channels and intracellular Ca2+ release in HepG2 human hepatoblastoma cells. Biochem. Biophys. Res. Commun. 261:682–688.
- Latham, K. M. and Stanbridge, E. J. 1990. Identification of the HeLa tumor-associated antigen, p75/150, as intestinal alkaline phosphatase and evidence for its transcriptional regulation. Proc. Natl. Acad. Sci. U.S.A. 87:1263–1267.
- Liu, X., Kim, C. N., Yang, J., Jemmerson, R. and Wang, X. 1996. Induction of apoptotic program in cell-free extracts: requirement for dATP and cytochrome c. Cell 86:147–157.
- Liu, C. Y., Lai, Y. Y. and Lo, C. J. 2004. Levamisole modulates prostaglandin E2 production and cyclooxygenase II gene expression in human colonic cancer cells. J. Surg. Res. 117:223–231.
- Marty, L. M. and Feldbush, T. L. 1993. Effect of anti-alkaline phosphatase monoclonal antibody on B-lymphocyte function. Immunol. Lett. 38:87–95.
- Matsumoto, H., Erickson, R. H., Gum, J. R., Yoshioka, M., Gum, E. and Kim, Y. S. 1990. Biosynthesis of alkaline phosphatase during differentiation of the human colon cancer cell line Caco-2. Gastroenterology 98:1199–1207.
- Millán, J. L. 1992. Alkaline phosphatase as a reporter of cancerous transformation. Clin. Chim. Acta 209:123–129.
- Millán, J. L. and Fishman, W. H. 1995. Biology of human alkaline phosphatases with special reference to cancer. Crit. Rev. Clin. Lab. Sci. 32:1–39.
- Millán, J. L. (Ed.) 2006. Mammalian Alkaline Phosphatases–From Biology to Applications in Medicine and Biotechnology. Weinheim, Germany: Wiley-VCH.
- Moertel, C. G., Fleming, T. R., Macdonald, J. S., Haller, D. G., Laurie, J. A., Tangen, C. M., Ungerleider, J. S., Emerson, W. A., Tormey, D. C., Glick, J. H., Veeder, M. H. and Mailliard, J. A. 1995. Fluorouracil plus levamisole as effective adjuvant therapy after resection of Stage III colon carcinoma: a final report. Ann. Intern. Med. 122:321–326.
- Mosmann, T. 1983. Rapid colorimetric assay for cellular growth and survival: application to proliferation and cytotoxicity assays. J. Immunol. Methods 65:55–63.
- Padmaja, K. and Ramanadham, M. 1998. Alkaline phosphatase activity is expressed in murine splenic B-lymphocytes sensitized in vivo with tetanus toxoid. Immunol. Lett. 61:175–178.
- Padmaja, K. 1999. Role of Alkaline Phosphatase in B-Lymphocyte Activation. University of Hyderabad. Hyderabad, India, Ph.D Thesis.
- Sanchez de Medina, F., Martinez-Augustin, O., Gonzalez, R., Ballester, I., Nieto, A., Galvez, J. and Zarzuelo, A. 2004. Induction of alkaline phosphatase in the inflamed intestine: A novel pharmacological target for inflammatory bowel disease. Biochem. Pharmacol. 68:2317–2326.
- Wei, J. S., Chung, N. C., Wei, L. L., Tzeng, W. F., Liu, T. Z. and Wang, J. Y. 1993. High-molecular-mass alkaline phosphatase as a tumor marker for colorectal cancer: Comparison of two test methods. Clin. Chem. 39:540–543.
- Yip, D., Strickland, A. H., Karapetis, C. S., Hawkins, C. A. and Harper, P. G. 2000. Immunomodulation therapy in colorectal carcinoma. Cancer Treat. Rev. 26:169–190.