Abstract
Mechanical ventilation with hyperoxia is a necessary treatment for patients with respiratory distress. However, patients on mechanical ventilation have increased susceptibility to infection. Studies including ours have shown that reactive oxygen species (ROS), generated by exposure to prolonged hyperoxia, can cause a decrease in the phagocytic activity of alveolar macrophages. Hydrogen peroxide (H2O2) is a form of ROS generated under hyperoxic conditions. In this study, we examined whether treatment with H2O2 directly affects macrophage phagocytic ability in RAW 264.7 cells that were exposed to either 21% O2 (room air) or 95% O2 (hyperoxia). Moderate concentrations (ranging from 10 to 250 µM) of H2O2 significantly enhanced macrophage phagocytic activity and restored hyperoxia-suppressed phagocytosis through attenuation of hyperoxia-induced disorganization of actin cytoskeleton and actin oxidation. These results indicate that H2O2 at low–moderate concentrations can be beneficial to host immune responses by improving macrophage phagocytic activity.
Abbreviations: | ||
H2O2, | = | hydrogen peroxide; |
ROS, | = | reactive oxygen species; |
O2, | = | molecular oxygen; O2−, super-oxide; |
PA, | = | pseudomona aeruginosa; |
VAP, | = | ventilator-associated pneumonia; |
AM, | = | alveolar macrophages; |
DAPI, | = | 4-6-diamidine-2-phenylindoledihydrochloride; |
MTT, | = | [3-(4,5-dimethylthia-zol-2-yl)-2,5-diphenyl tetrazolium bromide]; |
RA, | = | room air; |
OD, | = | optical density |
Introduction
Oxygen therapy (OT) using mechanical ventilation with hyperoxia is a necessary life-saving intervention for patients with respiratory distress (Make et al., Citation1998). However, a significant number of patients on OT suffer from adverse consequences, such as inflammatory lung injury and ventilator-associated pneumonia (VAP) (Nicasio et al., Citation2010). According to the National Nosocomial Infection Survey, 86% of nosocomial pneumonia is associated with OT (Richards et al., Citation1999). A postmortem study showed that pneumonia was present in up to 67% of patients who received OT (Marquette et al., Citation1995). A study with patients from 59 hospitals indicates that 29.3% of mortality rate is associated with bacteria culture–positive VAP (Kollef et al., Citation2005). In addition, high morbidity rate has been reported in patients with VAP (Ramirez Barba et al., Citation2006; Leblebicioglu et al., Citation2007). Many cases of VAP are attributed to Gram-negative aerobic organisms, among which Pseudomonas aeruginosa (PA) is responsible for 21% of the nosocomial pneumonia cases (Richards et al., Citation1999).
Prolonged exposure to hyperoxia can impair pulmonary host defense resulting in an increased susceptibility to Gram-negative bacteria such as Klebsiella pneumonia and PA, and exacerbates VAP (Baleeiro et al., Citation2003, Citation2006; Kikuchi et al., Citation2009; Reddy et al., Citation2009). Alveolar macrophages (AMs) are essential in lung innate immunity and play a critical role in the clearance of invading pathogens. Studies including ours indicate that AMs isolated from hyperoxic mice and cultured macrophages exposed to hyperoxia exhibit impaired ability in chemotaxis, adherence, phagocytosis, and pathogen-killing (Raffin et al., Citation1980; Rister, Citation1982; Suttorp and Simon, Citation1983; Crowell et al., Citation1995; Morrow et al., Citation2007).
Excessive amounts of reactive oxygen species (ROS) have been shown to mediate pathogenesis of various pulmonary diseases and tissue injury (Ciencewicki et al., Citation2008). Toxic levels of ROS generated under hyperoxic conditions can compromise phagocytic function of AMs (O’Reilly et al., Citation2003; Morrow et al., Citation2007). Besides superoxide, hydrogen peroxide (H2O2) is another form of ROS that is generated under hyperoxic conditions (Turrens et al., Citation1982). Although H2O2 has been used to induce oxidative stress to study the effects of ROS on various cellular functions (Lamb and Dixon, Citation1997; Svobodová et al., Citation2006), its role in phagocytic activity of phagocytes is unclear. H2O2 was shown to induce phagocytic function of human neutrophils (Bejarano et al., Citation2007). Oosting et al. (Citation1990), however, showed that H2O2 impaired the phagocytic activity of AMs. In this study, we examined whether the effect of H2O2 on the phagocytic activity of macrophages is concentration dependent. Furthermore, we examined whether H2O2 affects hyperoxia-induced macrophage dysfunction.
Materials and methods
Cell culture and reagents
Murine macrophage-like RAW 264.7 cells (American Type Culture Collection, Manassas, VA) were cultured in RPMI medium 1640 (Gibco BRL, Grand Island, NY) supplemented with 10% fetal bovine serum (Gemini Biological Produces, Catabasas, CA), 1% penicillin, and streptomycin (Life Technologies, Carlsbad, CA) at 37°C in 5% CO2/21% O2 (normoxia). Exposure to hyperoxia was performed in sealed humidified modular incubator chambers (Billups-Rothenberg Inc., Del Mar, CA) containing 95% O2/5% CO2 at 37°C, as described previously (Morrow et al., Citation2007). H2O2 (30%) was purchased from VWR International (West Chester, PA). All other chemicals/reagents were purchased from Sigma-Aldrich (St. Louis, MO), unless otherwise indicated.
Phagocytosis assay
PAO1, a non-mucoid strain of PA expressing green fluorescent protein, was grown to log phase in Lenox medium (Becton-Dickinson Inc., Sparks, MD). The phagocytosis assay was performed as described with minor modifications (Baleeiro et al., Citation2003; Morrow et al., Citation2007). In brief, following each specific exposure, RAW 264.7 cells were treated with various concentrations of H2O2, ranging from 0 to 1000 µM for 1 h, and then incubated with PAO1 or fluorescein isothiocyanate (FITC)-labeled latex beads (Polysciences, Warrington, PA) at 100:1 [particles (beads or bacteria)/cell] at 37°C for 1 h. The macrophages were then washed with cold phosphate-buffered saline (PBS) containing 0.02% EDTA to remove the unbound particles and then treated with 0.04% trypan blue to quench the fluorescence attributable to any extracellular adherent particles. The cells were then fixed with 4% paraformaldehyde for 10 min, washed with PBS, and then stained with 5 µg/ml of 4-6-diamidine-2-phenylindoledihydrochloride and Texas Red X-phalloidin (Molecular Probes, Eugene, OR) in 1% bovine serum albumin. The uptake of PAO1 or FITC-labeled latex beads was analyzed using fluorescence microscopy (Nikon Eclipse TE 2000-S; Nikon, Peabody, MA) and quantified by counting 100 macrophages/slide, and three slides each from three independent experiments.
Cell viability analyses
Cell viability assays (MTT reduction and trypan blue exclusion) were performed as previously described (Franek et al., Citation2004). For the MTT assay, RAW 264.7 macrophages were seeded on 48-well plates in RPMI 1640 medium. After the exposure or treatments, the RAW cells were incubated with 0.5 mg/ml of MTT [3-(4,5-dimethylthiazol-2-yl)-2,5-diphenyltetrazolium bromide] for 2 h at 37°C. The reaction was stopped by removing the resulting supernatant and the remaining cells were incubated with isopropanol. The amount of MTT formazan product was determined by measuring the absorbance in a microplate reader (Multiskan EX; Thermo Electron Corporation, Shanghai, China) at 569 nm. All data were presented as means ± standard error.
For the trypan blue exclusion assay, cell viability was assessed (following specific exposure or treatment) in hemocytometers placed using an inverted light microscope (Eclipse TS100/TS100-F; Nikon) with DS Camera control Unit DS-L2 (digital sight capture). The percentage of viable cells was determined by counting 100 macrophages/slide, and three slides each from three independent experiments were quantified. All data were presented as the ratio of the viable cells to the total number of cells counted.
Actin oxidation analysis
Oxidation of actin was determined by assessing carbonyl formation as described in our published studies (Franek et al., Citation2002; Morrow et al., Citation2007). RAW cells were lysed by sonication in hypotonic lysis buffer [50 mM NaCl, 50 µM MgCl2, 5 mM EGTA, 0.1% Triton X-100, 50 mM TRI (pH 7.4)], containing 50 mM dithiothreitol and protease inhibitors, and incubated with 20 µM cytochalasin D for 1 h on ice. The lysed cells were then centrifuged at 14,000g. Equal amounts of the resulting cell lysates were incubated with 20 µl/ml Protein A/G Plus Agarose (Santa Cruz Biotechnology, Santa Cruz, CA) and 2 µg/ml polyclonal goat anti-actin (Research Diagnostics Inc., Concord, MA) at 4°C. The precipitates were then washed with washing buffer [300 mM NaCl, 0.1% Triton X-100, 50 mM Tris (pH 7.4)], followed by washing buffer containing 0.1% sodium dodecyl sulfate (SDS) and 0.1% sodium deoxycholic acid, and then with PBS. The resulting pellets were re-suspended in 6% SDS solution and incubated with one volume of 20 mM 2,4-dinitrophenylhydrazine (DNPH) in 10% trifluoroacetic acid (TFA) for 20 min to convert all carbonyl groups to hydrazone derivatives. The reaction was terminated by addition of 0.75 volumes of 2 M Tris-30% glycerol-19% β-mercaptoethanol (Zhu et al., Citation2000). Controls for the derivation reactions were obtained by incubating pellets with TFA alone without DNPH. The resulting proteins were resolved over an 8% SDS-PAGE gel and transferred to a polyvinylidene fluoride membrane. Carbonyls in the samples were ultimately detected using rabbit anti-dinitrophenyl and horseradish peroxidase–conjugated goat anti-rabbit IgG antibodies (OxyBlot; Intergen, Purchase, NY), according to the manufacturer’s instructions.
Statistical analysis
All experiments were performed independently at least three times. The data are presented as means ± standard error, and analyzed for statistical significance using a Student’s t-test and analysis of variance. A p-value of ≤0.05 was considered significant.
Results
H2O2 has a differential effect on macrophage phagocytic activity
To test the effect of H2O2 on macrophage phagocytic activity, RAW 264.7 cells were exposed to a range of concentrations of H2O2 (50–1000 µM) and the uptake of latex beads by these macrophages was assessed. Data shown in indicate that H2O2 had a differential effect on the ability of macrophages to phagocytose latex beads. At concentrations from 50 to 250 µM, H2O2 significantly enhanced macrophage phagocytic activity (). The number of inert latex beads engulfed per macrophage was increased from 3.2 ± 0.2 (0 µM) to 4.1 ± 0.2 (50 µM), and 5.0 ± 0.4 (250 µM) beads/cell. Consistent with the observations by Oosting et al. (Citation1990), H2O2 at 750 µM significantly suppressed the ability of the RAW cells to phagocytose. The average number of ingested latex beads per macrophage was reduced from 3.2 ± 0.2 (0 µM) to 0.4 ± 0.2 beads/cell (750 µM; p < 0.001; ). At this concentration, H2O2 caused significant damage to macrophages, evidenced by the loss of cortical actin structures and increased amounts of stress fibers (). Similar results were obtained in macrophages treated with 1 mM H2O2 (data not shown). Therefore, these data suggest that the effect of H2O2 on macrophage phagocytosis is dose dependent and importantly, H2O2 can facilitate macrophage phagocytosis of latex beads.
Figure 1. The differential effect of H2O2 on macrophage phagocytic activity. RAW 264.7 cells were exposed to H2O2 at various concentrations for 1 h and then incubated with FITC-labeled latex beads (green) for 1 h. Cells were stained with rhodamine phalloidin (red) and DAPI (blue) to visualize the cells and nuclei in the field. (A) Immunofluorescence micrographs of RAW cells (magnification 200×). Each image represents those from three independent experiments. (B) Quantification of macrophage phagocytic activity. At least 100 cells per slide were counted. Each value represents mean ± SE. Value is significantly (*p ≤ 0.05, **p ≤ 0.01, and ***p ≤ 0.001) different from samples without H2O2 treatment.
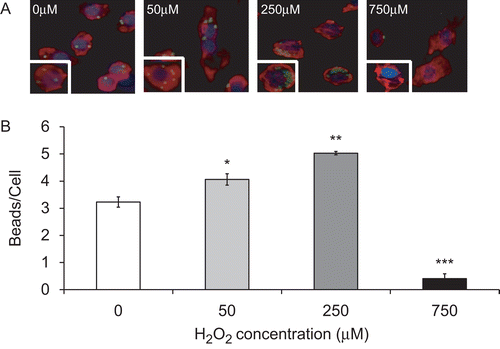
To determine whether the effect of H2O2 on macrophage function correlates with its effect on cell viability/cell death, MTT and trypan blue exclusion assays were performed. Although the percentage of viable cells exposed to H2O2 at ≤250 µM was not substantially changed, it was significantly decreased following exposure to H2O2 at ≥300 µM from 95.5 ± 0.3% (0 µM) to 91.5 ± 1.4% at 300 µM (p < 0.05) and to 41.0 ± 1.3% at 1000 µM (p < 0.001; ). The decrease in cell viability was also observed in data derived from MTT analysis (data not shown). To investigate whether H2O2 affects the viability of macrophages in hyperoxia, RAW 264.7 cells were cultured in 95% O2 for 24 h and then exposed to various concentrations of H2O2 for 1 h. Exposure to hyperoxia was toxic to RAW 264.7 cells as shown in , reducing the cell survival from 97.0 ± 1.26% in normoxic cells (RA—0 µM) to 83.8 ± 0.78% (O2—0 µM, p < 0.001; ). H2O2 at concentrations of 750 and 1000 µM significantly augmented the oxygen toxicity. The cell viability reduced from 83.8 ± 0.78% (O2—0 µM) to 50.7 ± 0.97% (O2—750 µM, p < 0.001) and 22.2 ± 0.95% (O2—1000 µM, p < 0.001), respectively (). In contrast, H2O2 at concentrations of 10–500 µM significantly reversed hyperoxia-induced loss of cell viability. The percentage of viable cells significantly increased from 83.8 ± 0.78% (O2—0 µM) to 98.7 ± 1.6% (O2—200 µM), and to 92.2 ± 0.47% at O2—500 µM H2O2 ().
Table 1. The effect of H2O2 on cell survival.
Table 2. Moderate concentrations of H2O2 reversed hyperoxia-induced suppression of cell survival in macrophages.
H2O2 restores hyperoxia-suppressed ability of macrophages to phagocytose microorganisms
Studies in our and other laboratories have shown that hyperoxia can impair the ability of AMs to phagocytose bacteria (Baleeiro et al., Citation2003; O’Reilly et al., Citation2003; Morrow et al., Citation2007). To determine whether H2O2 can enhance macrophage function in hyperoxia, RAW cells were exposed to H2O2, ranging from 50 to 750 µM, for 1 h following exposure to hyperoxia for 24 h. Interestingly, H2O2 at concentrations between 50 and 250 µM significantly restored the ability of hyperoxic macrophages to phagocytose latex beads. The number of beads phagocytosed per macrophage increased from 1.5 ± 0.3 (O2—0 µM) to 3.9 ± 0.2 (O2—50 µM), and to 4.0 ± 0.1 beads/cell (O2—250 µM; ). In addition, not only did H2O2 enhance the ability of hyperoxic macrophages to phagocytose, but this enhancement significantly surpassed the levels of normoxic (RA) macrophages too (2.7 ± 0.2, p < 0.01). In contrast, the number of latex beads engulfed by macrophages exposed to 750 µM H2O2 decreased significantly from 1.5 ± 0.3 (O2—0 µM) to 0.2 ± 0.02 beads/cell (O2—750 µM, p < 0.01), suggesting that H2O2 at 750 µM is cytotoxic to RAW cells under both normoxic and hyperoxic conditions ( and ). Similarly, treatment with moderate concentrations of H2O2 reversed any hyperoxia-suppressed macrophage phagocytosis following exposure to 48 h 95% O2; the end-point values increased from 0.8 ± 0.2 (O2—0 µM) to 4.8 ± 0.1 beads/cell (O2—50 µM) to 6.2 ± 0.1 beads/cell (O2—250 µM, p < 0.001; ).
Figure 2. H2O2 rescues hyperoxia-suppressed phagocytic activity. RAW 264.7 cells were exposed to 95% O2 for 24 h or remained in normoxia (RA), then treated with various concentrations of H2O2 for 1 h, and incubated with FITC-labeled latex beads (green). Cells were stained with rhodamine phalloidin (red) and DAPI (blue) to visualize the cells and nuclei in the fields. (A) Immunofluorescence micrographs of RAW cells (magnification 200×). Each Image represents those from three independent experiments. (B) Quantification of phagocytic ability. At least 100 cells per slide were counted. Each value represents mean ± SE. Value is significantly (*p ≤ 0.05, **p ≤ 0.01, ***p ≤ 0.001) different from normoxia (RA) control; value is significantly (++p ≤ 0.01, +++p ≤ 0.001) different from 95% O2 sample.
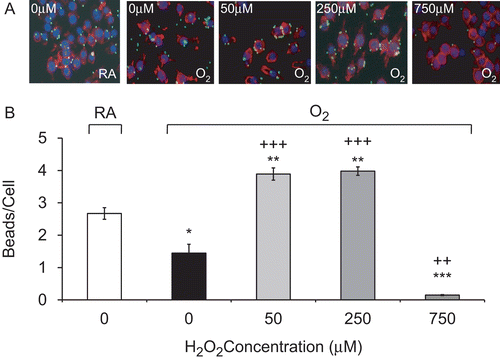
Figure 3. H2O2 rescues prolonged hyperoxia-impaired phagocytosis. RAW 264.7 cells were exposed to 95% O2 for 48 h or remained in normoxia (RA), then treated with various concentrations of H2O2 for 1 h, and incubated with FITC-labeled latex beads (green). Cells were stained with rhodamine phalloidin (red) and DAPI (blue) to visualize the cells and nuclei in the fields. (A) Immunofluorescence micrographs of RAW cells (magnification 400×). Each image represents those from three independent experiments. (B) Quantification of the phagocytic efficiency of RAW cells. At least 100 cells per slide were counted. Each value represents mean ± SE, in triplicate of three independent experiments. Value is significantly (*p ≤ 0.05, **p ≤ 0.01, ***p ≤ 0.001) different from normoxia (RA) samples; value is significantly (+++p ≤ 0.001) different from 95% O2 samples.
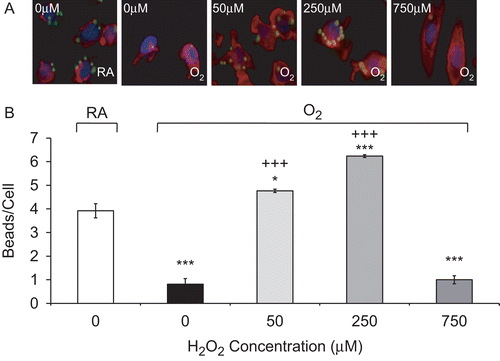
We next tested whether H2O2 could restore the hyperoxia-suppressed ability of macrophages to phagocytose bacteria PA. shows that H2O2 at 10–250 µM enhanced the ability of hyperoxic macrophages to phagocytose PA in a dose-dependent manner. The number of bacteria phagocytosed per macrophage increased from 4.6 ± 0.7 at 0 µM to 29.4 ± 1.9 bacteria/cell at 250 µM (p < 0.001). H2O2 at >250 µM still enhanced the phagocytic activity of the hyperoxic macrophages. The number of bacteria phagocytosed by hyperoxic RAW cells treated with 750 µM of H2O2 was significantly greater than that ingested by hyperoxic cells without H2O2 treatment (7.2 ± 0.6, p < 0.05 vs 4.6 ± 0.7 at 0 µM; ).
Figure 4. H2O2 rescues hyperoxia-suppressed phagocytosis of Pseudomonas aeruginosa (PA) by macrophages. RAW 264.7 cells were exposed to 95% O2 for 24 h or remained in normoxia (RA), then treated with different concentrations of H2O2 for 1 h, and incubated with PA (green). Cells were stained with rhodamine phalloidin (red) and DAPI (blue) to visualize the cells and nuclei in the fields. (A) Immunofluorescence micrographs of RAW cells (magnification 600×). Each image represents those from three independent experiments. (B) Quantification of the phagocytic ability. At least 100 cells per slide were counted. Data are representative of three independent experiments. Each value represents mean ± SE. Value is significantly (*p ≤ 0.05, **p ≤ 0.01, ***p ≤ 0.001) different from 95% O2 samples; value is significantly (+p ≤ 0.05, ++p ≤ 0.01, +++p ≤ 0.001) different from normoxia (RA) samples.
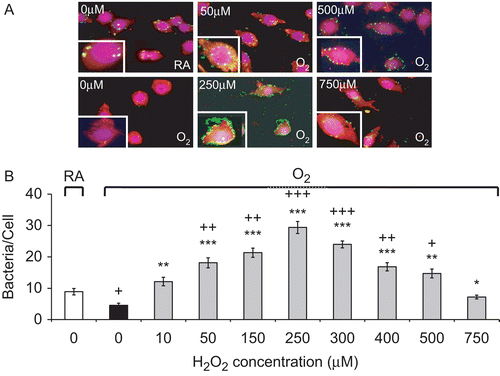
H2O2 reduces hyperoxia-induced actin oxidation
Our previous studies showed that hyperoxia-reduced macrophage phagocytosis was associated with reduced formation of filopodia and lamellipodia and elevated stress fiber formation, which can be attenuated by antioxidant treatments (Morrow et al., Citation2007). To determine the mechanisms underlying the rescuing effect of H2O2 on hyperoxia-induced macrophage dysfunction, the organization of F-actin cytoskeleton was examined. RAW 264.7 cells were treated with different concentrations of H2O2 (from 10 to 750 µM) for 1 h following exposure to hyperoxia for 24 h, and subsequently incubated with PA for 1 h. H2O2 at levels ranging from 10 to 250 µM stimulated filopodia and lamellipodia formation and attenuated cytoskeleton disorganization in the hyperoxic macrophages (cell microscopy pictures available from Authors by request). In contrast, macrophages treated with high concentrations of H2O2 (≥750 µM) did not exhibit improved cellular morphology in hyperoxia. These results indicate that moderate concentrations of H2O2 can restore normal formation of filopodia and lamellipodia in hyperoxic macrophages.
Studies from our and other laboratories have shown that actin oxidation plays a critical role in the compromised macrophage phagocytosis under hyperoxic conditions (O’Reilly et al., Citation2003; Morrow et al., Citation2007). To address whether H2O2 affects macrophage function via altering actin oxidation, the extent of carbonyl formation was assessed in immunoprecipitated total actin protein isolated from RAW cells as described previously by Morrow et al. (Citation2007). The data presented in show that treatment with 50 and 250 µM H2O2 significantly reduced the amount of oxidized actin in hyperoxic macrophages, suggesting that H2O2 exhibits its effects on macrophage phagocytosis in hyperoxia by reducing actin oxidation.
Figure 5. H2O2 reduces hyperoxia-induced actin oxidation. Actin was immunoprecipitated from RAW cells, that were cultured in 95% O2 for 24 h and then treated with different concentrations of H2O2 for 1 h. Cell lysates were incubated with 2,4-DNPH in TFA to convert carbonyl groups to their 2,4-DNP–hydrazone derivatives. The resulting proteins were resolved on SDS-PAGE and Western blotting analysis was performed using anti-DNP primary and then HRP-conjugated secondary antibodies. (A) Blots represented oxidized actin. (B) Blots represent total amounts of actin. (C) Quantification of blots represented in panel A. Data are expressed in arbitrary units. Each value represents mean ± SE of three independent experiments. Value is significantly (*p ≤ 0.05, ***p ≤ 0.001) different from that of hyperoxic controls.
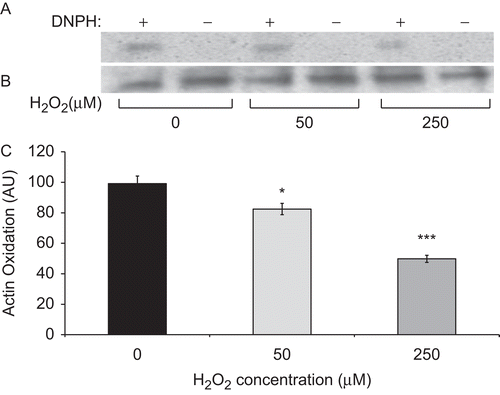
Discussion
In this study, we have shown that H2O2 enhanced phagocytic activity in a dose-dependent manner in cultured macrophages. In addition, a brief treatment with H2O2 reversed the hyperoxia-induced suppression of the ability of macrophages to phagocytose both PA and inert latex beads. Accompanied with the enhanced phagocytic activity, macrophages treated with H2O2 had reduced stress fiber formation and actin oxidation that was induced by prolonged exposure to hyperoxia (Morrow et al., Citation2007).
H2O2, a form of ROS generated under hyperoxic conditions, has the potential to damage proteins, lipids, and nucleic acids (Bienert et al., Citation2006). Therefore, many studies have focused on the adverse effects of H2O2 on altering macromolecule functions that can subsequently cause cellular damage (Schröder and Eaton, Citation2008). However, emerging evidence has illustrated the essential roles that H2O2 plays in a variety of physiological functions (Bienert et al., Citation2006; Forman, Citation2007). In this study, we demonstrated that macrophage function in phagocytosis could be enhanced following a brief exposure to moderate concentrations of H2O2 under both normoxic and hyperoxic conditions. These results suggest that it is unlikely that H2O2 contributes to hyperoxia-suppressed phagocytic activity unless the local concentration of H2O2 exceeded 500 µM. Our finding is consistent with an early report showing that catalase did not exhibit a significant effect on macrophage phagocytosis in hyperoxia, although the effect of hyperoxia on bacterial adherence to mononuclear cells was ameliorated by catalase (Arita et al., Citation2007).
Actin cytoskeleton organization plays an essential role in phagocytosis. Phagocytosis requires well-coordinated cytoskeletal rearrangements driven by actin polymerization (Kuiper et al., Citation2008). Actin polymerization is critical in the movement of cells toward the particles and for their ingestion (May and Machesky, Citation2001). Haberzettl et al. (Citation2007) showed that inhibition of actin polymerization caused a significant reduction of particle uptake in interstitial macrophages as well as rat AMs. Previous studies including ours indicate that hyperoxia impairs the ability of macrophages to phagocytose bacteria by interfering with the directional actin polymerization/remodeling that plays an essential role in the internalization of bacteria (O’Reilly et al., Citation2003; Morrow et al., Citation2007; Acosta-Iborra et al., Citation2009). The dysfunction of macrophages in hyperoxia is associated with increased formation of stress fibers and loss of cortical actin (O’Reilly et al., Citation2003; Morrow et al., Citation2007; Acosta-Iborra et al., Citation2009). In this report, our results indicate that moderate concentrations of H2O2 can restore the morphological changes of actin cytoskeleton organization under hyperoxic conditions. The primary morphological changes observed in hyperoxic macrophages were attenuated by H2O2: few stress fibers were formed and the reduced formation of filopodia and lamellipodia was restored. This observation was also made by others showing that H2O2 can induce formation of F-actin cytoskeleton without increasing actin nucleation activity (Omann et al., Citation1994).
Oxidation of actin has been demonstrated in numerous cell types exposed to ROS (Boota et al., Citation1996, Ferro et al., Citation1997; Banan et al., Citation2001). Under hyperoxic condition, oxidation of actin was also detected by the increased amount of carbonyl formation in cultured macrophages (O’Reilly et al., Citation2003; Morrow et al., Citation2007). Oxidative modifications of the actin polypeptide chain can lead to alteration of the functions of proteins, including cross-linked proteins, spectrin, and fodrin which can cause impairment of interaction with other cytoskeletal proteins (Dalle-Donne et al., Citation1995, Citation1999, Citation2003; Milzani et al., Citation2000). Indeed, actin carbonylation has been shown to cause severe functional impairment associated with filament disruption (Dalle-Donne et al., Citation2001). Although H2O2 has been shown to alter actin interactions via oxidation of the thiol groups (Crowell et al., Citation1995), studies in this report indicate that moderate concentrations of H2O2 can reduce actin carbonylation in hyperoxic macrophages (). Even though H2O2 can cause oxidative damage, low doses of H2O2 have been shown to be involved in enhanced activities of antioxidant enzymes (Bose Girigoswami et al., Citation2005). We have previously demonstrated that antioxidants, including superoxide dismutase, can rescue hyperoxia-suppressed macrophage functions (Morrow et al., Citation2007). Therefore, the results shown in , i.e., demonstrating the reduced levels of actin oxidation in hyperoxic macrophages treated with moderate levels of H2O2, support the notion that moderate concentrations of H2O2 may induce levels of antioxidants under hyperoxic conditions. Inhibition of actin oxidation by moderate concentrations of H2O2 may play an important role in attenuating hyperoxia-induced excessive stress fiber formation and in restoring normal actin cytoskeleton organization.
In summary, our study demonstrates that moderate concentrations of H2O2 can significantly enhance macrophage phagocytic activity. In addition, H2O2 can restore hyperoxia-suppressed phagocytosis of bacteria through the attenuation of actin oxidation and maintenance of actin cytoskeleton organization. These findings suggest that H2O2 is beneficial to host immunity not only due to its direct bactericidal effects, but also its ability to improve macrophage functions.
Acknowledgements
We thank Dr. Charles R. Ashby Jr, Maitreyi Muralidhar, and Ashwini Gore for insightful discussion and invaluable suggestions on this project/manuscript. This work was supported by grants from the American Lung Association of New York State (LLM), National Heart and Blood Institute (HL093708, LLM), St. John’s University College of Pharmacy, and Ministry of Education and Training, Vietnamese Government. Dr. Maria Entezari (Tahereh Tahera) is currently at LaGuardia Community College, City University of New York.
Declaration of interest
The authors report no declarations of interest. The authors are alone responsible for the content and writing of the paper.
References
- Acosta-Iborra, B., Elorza, A., Olazabal, I. M., Martín-Cofreces, N. B., Martin-Puig, S., Miró, M., Calzada, M. J., Aragonés, J., Sánchez-Madrid, F. and Landázuri, M. O. 2009. Macrophage oxygen sensing modulates antigen presentation and phagocytic functions involving IFN-g production through the HIF-1a transcription factor. J. Immunol. 182:3155–3164.
- Arita, Y., Kazzaz, J. A., Joseph, A., Koo, H. C., Li, Y. and Davis, J. M. 2007. Antioxidants improve antibacterial function in hyperoxia-exposed macrophages. Free Radic. Biol. Med. 42:1517–1523.
- Baleeiro, C. E., Christensen, P. J., Morris, S. B., Mendez, M. P., Wilcoxen, S. E.and Paine, R. 2006. GM-CSF and the impaired pulmonary innate immune response following hyperoxic stress. Am. J. Physiol. Lung Cell Mol. Physiol. 291:L1246–L1255.
- Baleeiro, C. E., Wilcoxen, S. E., Morris, S. B., Standiford, T. J.and Paine, R., 3rd. 2003. Sub–lethal hyperoxia impairs pulmonary innate immunity. J. Immunol. 171:955–963.
- Banan, A., Fields, J. Z., Zhang, Y.and Keshavarzian, A. 2001. iNOS upregulation mediates oxidant-induced disruption of F-actin and barrier of intestinal monolayers. Am. J. Physiol. Gastrointest. Liver Physiol. 280:G1234–G1246.
- Bejarano, I., Terrón, M. P., Paredes, S. D., Barriga, C., Rodríguez, A. B.and Pariente, J. A. 2007. Hydrogen peroxide increases the phagocytic function of human neutrophils by calcium mobilization. Mol. Cell. Biochem. 296:77–84.
- Bienert, G. P., Schjoerring, J. K.and Jahn, T. P. 2006. Membrane transport of hydrogen peroxide. Biochim. Biophys. Acta 1758:994–1003.
- Boota, A., Zar, H., Kim, Y. M., Johnson, B., Pitt, B.and Davies, P. 1996. IL-1b stimulates superoxide and delayed peroxynitrite production by pulmonary vascular smooth muscle cells. Am. J. Physiol. 271:L932–L938.
- Bose Girigoswami, K., Bhaumik, G.and Ghosh, R. 2005. Induced resistance in cells exposed to repeated low doses of H2O2 involves enhanced activity of antioxidant enzymes. Cell Biol. Int. 29:761–767.
- Ciencewicki, J., Trivedi, S.and Kleeberger, S. R. 2008. Oxidants and the pathogenesis of lung diseases. J. Allergy Clin. Immunol. 122:456–68; quiz 469.
- Crowell, R. E., Hallin, G., Heaphy, E.and Mold, C. 1995. Hyperoxic suppression of Fc-gamma receptor-mediated phagocytosis by isolated murine pulmonary macrophages. Am. J. Respir. Cell Mol. Biol. 12:190–195.
- Dalle-Donne, I., Milzani, A.and Colombo, R. 1995. H2O2-treated actin: assembly and polymer interactions with cross-linking proteins. Biophys. J. 69:2710–2719.
- Dalle-Donne, I., Milzani, A.and Colombo, R. 1999. The tert-butyl hydroperoxide-induced oxidation of actin Cys-374 is coupled with structural changes in distant regions of the protein. Biochemistry 38:12471–12480.
- Dalle-Donne, I., Rossi, R., Giustarini, D., Gagliano, N., Lusini, L., Milzani, A., Di Simplicio, P.and Colombo, R. 2001. Actin carbonylation: From a simple marker of protein oxidation to relevant signs of severe functional impairment. Free Radic. Biol. Med. 31:1075–1083.
- Dalle-Donne, I., Rossi, R., Giustarini, D., Milzani, A.and Colombo, R. 2003. Protein carbonyl groups as biomarkers of oxidative stress. Clin. Chim. Acta 329:23–38.
- Ferro, T. J., Gertzberg, N., Selden, L., Neumann, P. and Johnson, A. 1997. Endothelial barrier dysfunction and p42 oxidation induced by TNF-a are mediated by nitric oxide. Am. J. Physiol. 272:L979–L988.
- Forman, H. J. 2007. Use and abuse of exogenous H2O2 in studies of signal transduction. Free Radic. Biol. Med. 42:926–932.
- Franek, W. R., Chowdary, Y. C., Lin, X., Hu, M., Miller, E. J., Kazzaz, J. A., Razzano, P., Romashko, J. 3rd, Davis, J. M., Narula, P., Horowitz, S., Scott, W.and Mantell, L. L. 2002. Suppression of nuclear factor-k B activity by nitric oxide and hyperoxia in oxygen-resistant cells. J. Biol. Chem. 277:42694–42700.
- Franek, W. R., Morrow, D. M., Zhu, H., Vancurova, I., Miskolci, V., Darley-Usmar, K., Simms, H. H.and Mantell, L. L. 2004. NF-kB protects lung epithelium against hyperoxia-induced nonapoptotic cell death-oncosis. Free Radic. Biol. Med. 37:1670–1679.
- Haberzettl, P., Duffin, R., Krämer, U., Höhr, D., Schins, R. P., Borm, P. J.and Albrecht, C. 2007. Actin plays a crucial role in the phagocytosis and biological response to respirable quartz particles in macrophages. Arch. Toxicol. 81:459–470.
- Kikuchi, Y., Tateda, K., Fuse, E. T., Matsumoto, T., Gotoh, N., Fukushima, J., Takizawa, H., Nagase, T., Standiford, T. J.and Yamaguchi, K. 2009. Hyperoxia exaggerates bacterial dissemination and lethality in Pseudomonas aeruginosa pneumonia. Pulm. Pharmacol. Ther. 22:333–339.
- Kollef, M. H., Shorr, A., Tabak, Y. P., Gupta, V., Liu, L. Z.and Johannes, R. S. 2005. Epidemiology and outcomes of health-care-associated pneumonia: Results from a large US database of culture-positive pneumonia. Chest. 128:3854–3862.
- Kuiper, J. W., Pluk, H., Oerlemans, F., van Leeuwen, F. N., de Lange, F., Fransen, J.and Wieringa, B. 2008. Creatine kinase-mediated ATP supply fuels actin-based events in phagocytosis. PLoS Biol. 6:e51.
- Lamb, C.and Dixon, R. A. 1997. The oxidative burst in plant disease resistance. Annu. Rev. Plant Physiol. Plant Mol. Biol. 48:251–275.
- Leblebicioglu, H., Rosenthal, V. D., Arikan, O. A., Ozgültekin, A., Yalcin, A. N., Koksal, I., Usluer, G., Sardan, Y. C.and Ulusoy, S.; Turkish Branch of INICC. 2007. Device-associated hospital-acquired infection rates in Turkish intensive care units. Findings of the International Nosocomial Infection Control Consortium (INICC). J. Hosp. Infect. 65:251–257.
- Make, B. J., Hill, N. S., Goldberg, A. I., Bach, J. R., Criner, G. J., Dunne, P. E., Gilmartin, M. E., Heffner, J. E., Kacmarek, R., Keens, T. G., McInturff, S., O’Donohue, W. J. Jr Oppenheimer, E. A., and Robert, D. 1998. Mechanical ventilation beyond the intensive care unit. Report of a consensus conference of the American College of Chest Physicians. Chest 113:289S–344S.
- Marquette, C. H., Copin, M. C., Wallet, F., Neviere, R., Saulnier, F., Mathieu, D., Durocher, A., Ramon, P.and Tonnel, A. B. 1995. Diagnostic tests for pneumonia in ventilated patients: Prospective evaluation of diagnostic accuracy using histology as a diagnostic gold standard. Am. J. Respir. Crit. Care Med. 151:1878–1888.
- May, R. C.and Machesky, L. M. 2001. Phagocytosis and the actin cytoskeleton. J. Cell. Sci. 114:1061–1077.
- Milzani, A., Rossi, R., Di Simplicio, P., Giustarini, D., Colombo, R.and DalleDonne, I. 2000. The oxidation produced by hydrogen peroxide on Ca-ATP-G-actin. Protein Sci. 9:1774–1782.
- Morrow, D. M., Entezari-Zaher, T., Romashko, J. 3rd Azghani, A. O., Javdan, M., Ulloa, L., Miller, E. J.and Mantell, L. L. 2007. Antioxidants preserve macrophage phagocytosis of Pseudomonas aeruginosa during hyperoxia. Free Radic. Biol. Med. 42:1338–1349.
- Nicasio, A. M., Eagye, K. J., Kuti, E. L., Nicolau, D. P.and Kuti, J. L. 2010. Length of stay and hospital costs associated with a pharmacodynamic-based clinical pathway for empiric antibiotic choice for ventilator-associated pneumonia. Pharmacotherapy. 30:453–462.
- Omann, G. M., Harter, J. M., Burger, J. M.and Hinshaw, D. B. 1994. H2O2-induced increases in cellular F-actin occur without increases in actin nucleation activity. Arch. Biochem. Biophys. 308:407–412.
- Oosting, R. S., van Bree, L., van Iwaarden, J. F., van Golde, L. M.and Verhoef, J. 1990. Impairment of phagocytic functions of alveolar macrophages by hydrogen peroxide. Am. J. Physiol. 259:L87–L94.
- O’Reilly, P. J., Hickman-Davis, J. M., Davis, I. C.and Matalon, S. 2003. Hyperoxia impairs antibacterial function of macrophages through effects on actin. Am. J. Respir. Cell Mol. Biol. 28:443–450.
- Raffin, T. A., Simon, L. M., Braun, D., Theodore, J.and Robin, E. D. 1980. Impairment of phagocytosis by moderate hyperoxia (40 to 60% oxygen) in lung macrophages. Lab. Invest. 42:622–626.
- Ramirez Barba, E. J., Rosenthal, V. D., Higuera, F., Oropeza, M. S., Hernández, H. T., López, M. S., Lona, E. L., Duarte, P., Ruiz, J., Hernandez, R. R., Chavez, A., Cerrato, I. P., Ramirez, G. E.and Safdar, N. 2006. Device-associated nosocomial infection rates in intensive care units in four Mexican public hospitals. Am. J. Infect. Control. 34:244–247.
- Reddy, N. M., Suryanarayana, V., Kalvakolanu, D. V., Yamamoto, M., Kensler,T. W., Hassoun, P. M., Kleeberger, S. R.and Reddy, S. P. 2009. Innate immunity against bacterial infection following hyperoxia exposure is impaired in NRF2-deficient mice. J. Immunol. 183:4601–4608.
- Richards, M. J., Edwards, J. R., Culver, D. H.and Gaynes, R. P. 1999. Nosocomial infections in medical intensive care units in the United States. National Nosocomial Infections Surveillance System. Crit. Care Med. 27:887–892.
- Rister, M. 1982. Effects of hyperoxia on phagocytosis. Blut 45:157–166.
- Schröder, E.and Eaton, P. 2008. Hydrogen peroxide as an endogenous mediator and exogenous tool in cardiovascular research: Issues and considerations. Curr. Opin. Pharmacol. 8:153–159.
- Suttorp, N.and Simon, L. M. 1983. Decreased bactericidal function and impaired respiratory burst in lung macrophages after sustained in vitro hyperoxia. Am. Rev. Respir. Dis. 128:486–490.
- Svobodová, A., Walterová, D.and Psotová, J. 2006. Influence of silymarin and its flavonolignans on H2O2-induced oxidative stress in human keratinocytes and mouse fibroblasts. Burns 32:973–979.
- Turrens, J. F., Freeman, B. A.and Crapo, J. D. 1982. Hyperoxia increases H2O2 release by lung mitochondria and microsomes. Arch. Biochem. Biophys. 217:411–421.
- Zhu, S., Basiouny, K. F., Crow, J. P.and Matalon, S. 2000. Carbon dioxide enhances nitration of surfactant protein A by activated alveolar macrophages. Am. J. Physiol. Lung Cell Mol. Physiol. 278:L1025–L1031.