Abstract
Extracts of Spondias mombin L. (Anacardiacea) is used in the traditional medicine of Africa and Latin America to treat many inflammatory conditions, with repeated claims of efficacy. However, there are no scientific data yet to support these claims and the mechanism through which the extract may be acting is still unknown. This study was undertaken to investigate the effects of the methanolic extract of the leaf of S. mombin (SM) on inflammation and to uncover some of the possible mechanisms that could explain any observed changes. The anti-inflammatory activity of the extract was investigated in Wistar rats using intraplantar injection of carrageenan as an in vivo model of inflammation. The effect of oral supplementation of the SM extract on tumor necrosis factor (TNF)-α levels after an intraperitoneal lipopolysaccharide (LPS; 1 mg/kg) challenge was investigated in mice. The effect of SM on TNF-α and inducible nitric oxide (iNO) production by LPS-stimulated bone marrow-derived macrophages (BM-MØ) was also investigated in vitro. BM-MØ were preincubated for 2 h with SM (0–100 µg/ml), activated with LPS, and then TNF-α and NO production measured in the cell-free conditioned culture supernatant after 24 h of incubation. The study showed that pre-treatment of rats with the SM extract (at 100, 200, and 400 mg/kg, per os) caused a significant dose-related inhibition of carrageenan-induced paw edema over a 4-h period. In treated mice, LPS-inducible (systemic) TNF-α levels were found to be significantly lower as a result of their receiving the SM extract. In vitro, SM treatment caused a dose-dependent decrease in LPS-inducible TNF-α and NO production by BM-MØ compared to the effects of treatment of the cells with LPS alone. Taken together, the results of these studies suggest that supplementation with SM extract can alleviate inflammatory responses and that this could possibly be via a suppression of the production of pro-inflammatory mediators and cytokines such as TNF-α and iNO.
Introduction
Spondias mombin L. (Anacardiacea) is a plant with versatile folklore uses. It is reputed, among other claims, to be effective in the treatment of inflammatory conditions, wounds, and infections. S. mombin (SM), commonly known as ‘Hog plum’ in English and ‘Ijikara’ by the Ibos of southeastern Nigeria, is a medium-sized tree (that occasionally grows into a large tree) widely present in tropical portions of the Americas, Asia, and Africa. The decoction of the leaves is commonly used to treat laryngitis, sour throat, and tooth decay; this product has also been very popular in traditional birth practices for its peri- and postpartum obstetrics uses (Akubue et al., Citation1983; Nworu et al., Citation2007). In Belize, the juice of the crushed leaves and the powder of dried leaves are used as poultices on wounds and sites of inflammation; in Mexico, the powdered bark is applied directly to wounds. Quite commonly, a tea (made from the flowers and leaves) is taken to relieve stomach aches, biliousness, urethritis, cystitis, as well as to ameliorate inflammation of the eye and/or throat (Ayoka et al., Citation2008). Lastly, the decoction of the astringent bark has been used as an emetic, a remedy for diarrhea, dysentery, and hemorrhoids, and also as a treatment for gonorrhea (Ayoka et al., Citation2008).
The leaves of SM have been reported to contain antiviral ellagitannins and caffeoyl esters, as well as antibacterial and molluscicidal phenolic acids (Corthout et al., Citation1994). The leaves have also been demonstrated to have antihelminthic (Ademola et al., Citation2005) and abortifacient (Offiah and Anyanwu, Citation1989; Nworu et al., Citation2007) properties. In traditional medical practice of southern Nigeria, the freshly boiled aqueous leaf extract of SM is used to treat dizziness, especially after childbirth, while the bark is used to treat mothers after delivery (Onwuka, Citation1992). The more general wound-healing properties of extracts from the leaves of this plant have also been investigated (Villegas et al., Citation1997).
In this study, we assessed the anti-inflammatory properties of a leaf extract (methanolic) of SM and the possible mechanisms of any effect. It was expected that these studies would provide a clearer understanding for the benefits against inflammatory conditions that have been claimed to result from traditional use of the various extracts and decoctions of the plant. Specifically, in the studies here we investigated: the acute anti-inflammatory properties of SM in an experimental rat model; the effect of oral supplementation of SM extract on lipopolysaccharide (LPS)-induced tumor necrosis factor (TNF)-α formation/release in vivo in a mouse model; and, the effect of the extract upon LPS-induced nitric oxide (NO) and TNF-α formation/release by bone marrow-derived macrophages (BM-MØ) in vitro.
Materials and methods
Collection and extraction of plant material
The fresh leaves of SM was collected and authenticated by a plant taxonomist, Mr Alfred O. Ozioko, of the Bioresources Development and Conservation Programme Centre, Nsukka, Nigeria. The leaves were washed, air-dried, and pulverized. A portion of the leaf powder (500 g) was exhaustively extracted by maceration in methanol for 48 h. The extract solution was then filtered through Whatman No. 1 filter paper and concentrated in a rotary evaporator to yield 68.49 g (13.7% recovery) of SM extract. Preliminary phytochemical tests were carried out on this SM using standard procedures (Harborne and Harborne, Citation1998). The level of any endotoxin present was determined using a Limulus Amoebocytes Lysate kit (Endosafe®; Charles River, Sulzfeld, Germany) on a 1 mg SM/ml solution; the results showed that there was no gel clot formed and thus the level of any endotoxin present in the extract was below the kit level of detection (0.03 EU).
Animals
Male Wistar rats (12 week-of-age; weighing 130–180 g) and BALB/c mice (10 week-of-age; weighing 20–26 g) obtained from the laboratory animal facility of the Faculty of Veterinary Medicine, University of Nigeria (Nsukka, Nigeria) and the Centre d’Elevage (Janvier, France) were used in the study. The animals were housed in clean pathogen-free institutional facilities under standard conditions (25 ± 2°C and a 12-h light/dark cycle) and maintained on standard pellets and drinking water ad libitum. The use and care of laboratory animals in the study were in accordance with ethical guidelines as contained in the European Convention for the Protection of Vertebrate Animals used for Experimental and Other Scientific Purposes (EEC Directive 86/609/EEC) of 1986.
Acute toxicity (LD50) test
The acute toxicity (LD50) of the methanol extract of SM extract was estimated in BALB/c mice by the oral route using the method described by Lorke (Citation1983). Briefly, the tests involved two phases. The first phase involved the determination of the toxic range. The mice were placed in three groups (n = 3) and SM (10, 100, and 1,000 mg/kg) suspended in 5% Tween 80 was administered orally. The use of 5% Tween 80 as a vehicle has been shown to be safe and is not expected to interfere with the activities of the extract (based on earlier studies of effects of Tween by Oser and Oser (Citation1956a,Citationb) and Williams et al. (Citation1997)). The treated mice were observed for 24 h for any deaths. The death pattern in the first phase determined the doses used for the second phase according to the Lorke (Citation1983) estimation. In the second phase, four different doses of SM were administered (per os) as predetermined in the earlier phase of the study. The animals were observed for lethality or signs of acute intoxication for the next 24 h. The LD50 was calculated as the geometric mean of the highest nonlethal dose and the least toxic dose.
Acute anti-inflammatory studies by carrageenan-induced paw edema in rats
Carrageenan-induced acute inflammation model (Winter et al., Citation1962) was employed to access the in vivo anti-inflammatory activities of SM. The animals were randomized into five groups (n = 6/group) and fasted for 6 h. Groups 1–3 received 100, 200, and 400 mg/kg per os, respectively, of the extract solubilized in 5% Tween 80; no anesthesia was utilized for the application. Group 4 rats received piroxicam (20 mg/kg, per os); piroxicam is an effective non–steroidal anti-inflammatory drug that has been routinely used as a standard for comparison in these types of studies (CitationMusa et al., 2008; Ayanwuyi et al., Citation2010). Rats in group 5 served as the negative control and were given only the vehicle (5% Tween 80 solution). The total volume of SM solution, piroxicam, or vehicle given to each rat/dosing was 0.5 ml. Animals were deprived of water only during the experiment.
Thirty minutes after drug administration, 0.1 ml of freshly prepared 1% carrageenan in sterile saline (as a phlogistic agent) was injected into the subplanter surface of each rat hind paw (Winter et al., Citation1962). The paw edema produced was then estimated by measuring the linear circumference of each paw before and at 1, 2, 3, and 4 h after induction of inflammation. Paw diameters were measured using a micrometer screw gauge. The mean paw circumference and percent (%) inhibition of edema were then determined to assess the level of inflammation in the extract-treated and control groups. The % inhibition was calculated using the formula:
where Ct is the linear circumference of edema at corresponding time (t) and Co is the linear circumference of the edema for vehicle-treated rats at the same time point.
Effect of SM supplementation on LPS-induced TNF-α release in vivo
BALB/c mice were randomized into four groups (n = 5) and treated for three consecutive days with SM (100 and 200 mg/kg, per os) or vehicle. On the third day, 2 h after the SM treatment, the mice were treated (by intraperitoneal injection) with LPS ((Escherichia coli, serotype 0128:B12); Sigma-Aldrich, Munich, Germany) at 1 mg/kg or phosphate-buffered saline (PBS) in a 100-μl volume. Group treatments were as follows: Group 1 mice were the naive controls that received only the vehicle and were not treated with LPS; Group 2 mice received no SM and were treated with LPS alone; mice in Groups 3 and 4 received daily 100 and 200 mg SM/kg per os treatments, respectively, followed by LPS on Day 3. No anesthesia was utilized for the application; the total volume presented to each mouse/dosing was 0.2 ml. The animals were bled from the retro-orbital plexus 2 h after the LPS (or PBS) treatment and the subsequently isolated sera were stored at −20°C until analyzed. Levels of TNF-α in the sera were determined with a commercial cytokine capture ELISA kit (PeproTech, Hamburg Germany) that had a detection limit of 16 pg/ml.
Generation of BM-MØ
Murine BM-MØ were generated from the BM cells of the tibia and femur of C57Bl/6 donor mice by a modification of previously described protocols (Lin et al., Citation2001; Weischenfeldt and Porse, Citation2008). BM cells were harvested and cultured in R-10 medium containing 10 ng/ml of recombinant murine colony-stimulating factor (rmCSF-1; Immunotools, Friesoythe, Germany) in T-75 cell culture flasks. The cells were incubated at 37°C and 5% CO2 for 24 h to adhere and remove stromal cells and mature BM resident macrophages. Nonadherent cells, which are mainly progenitors, were recovered after 24 h of incubation and further incubated in cell culture flasks to expand and differentiate the cells under the influence of the rmCSF-1.
After 7 days of culture, nonadherent cells were removed and the adherent cells were washed and harvested using a cell scraper. The viability of the generated macrophages was assessed by trypan blue exclusion. The cells generated were plated and used for the in vitro studies of the effects of SM on LPS-induced NO and TNF-α production by macrophages.
Viability of SM-treated BM-MØ assessed using an MTT assay
The viability of the BM-MØ after treatment with SM extract was determined using cellular respiration as an indicator. Cell viability was determined on the basis of mitochondrial-dependent reduction of MTT to formazan (Mosmann, Citation1983). BM-MØ were cultivated in 96-well plates (1 × 104 cells/well) for 24 h. The cells were then treated with various concentrations (0, 5, 25, and 100 µg/ml) of SM in a fixed volume of 100 µl. After 24 h of incubation at 37°C, the medium in each well was discarded; the cells were then incubated with fresh medium containing 5 mg MTT/ml for 4 h. The formazan blue that formed in the cells was then dissolved by addition of 100-µl dimethyl sulfoxide to each well and the optical density of the solution in the well was measured at 550 nm in a multiwell microtiter plate reader (Tecan, Grödig, Austria).
Measurement of NO concentration by Griess reagent
BM-MØ were cultivated in 48-well plates (5 × 105 cells/well) at 37°C in a 5% CO2 incubator for 24 h. Thereafter, the cells were pretreated with various concentrations (0, 5, 25, and 100 µg/ml) of SM. After 2 h of incubation, the cells were then treated with 10 µg/ml of LPS or vehicle (PBS) only. Supernatants were collected after 24 h of incubation and stored at −80°C. Nitrite levels in aliquots of each supernatant were measured in 96-well microtiter plates by mixing 100 µl of cell-free culture supernatant with an equal volume of Griess reagent (Applichem, Darmstadt, Germany) and then incubated at room temperature for 10 min. The Griess reagent contains equal volumes of 0.1% naphthylethylenediamine dihydrochloride and 1% sulphanilamide in 5% phosphoric acid. The NO concentration was determined at 550 nm in a multiwell microtiter plate reader (Tecan) by extrapolation from a standard curve generated using NaNO2 standards that had been included in each measurement plate.
Measurement of TNF-α in culture supernatant
BM-MØ (5 × 105 cells/well) were cultured in 48-well plates for 24 h. The cells were then pretreated with various concentrations (0, 5, 25, and 100 µg/ml) of SM for 2 h. After this period of incubation, the cells were treated with 10 µg LPS/ml or vehicle. After 24-h incubation at 37°C, the cell-free medium in each well was collected and the TNF-α concentration in the material assayed via a commercial cytokine ELISA kit as described earlier.
Statistical analyses
The data are presented as mean (±SEM) and were analyzed by analysis of variance using GraphPad Prism 5 software (GraphPad Software, Inc., San Diego, CA). Generally, differences between test treatments and the control treatment were considered significant at P < 0.05.
Results
Sample analyses
The yield of the methanol extract of SM leaf was 68.5 g (13.7% (wt/w)). In earlier studies, several of the major phytoconstituents present in the leaf extract of S. monbin were quantified. These studies indicated the general presence of saponins, flavonoids, tannins, glycosides, resins, triterpenoids, and steroids; specifically, the leaf extract has been reported to contain 3.82% tannins, 7.60% saponins, 3.00% flavonoids, 6.00% alkaloids, and 1.00% other phenolic compounds (Njoku and Akumefula, Citation2007).
Acute toxicity (LD50) test
At doses up to 5,000 mg/kg per os, the SM did not result in deaths and did not cause overt signs of acute intoxication in the mice.
Acute anti-inflammatory activity
Pre-treatment of the Wistar rats with the methanol extract of SM caused a significant (P < 0.05) and dose-related inhibition of carrageenan-induced inflammation leading to a more rapid resolution of paw edema over a 4-h period of measurement (). In the control rats, paw edema reached a maximum of 2.59 (±0.16) mm in the first hour and then decreased to 1.84 (±0.24) mm by the end of the 4-h time frame. In contrast, rats that had received the 400 mg SM/kg regimen had only a 1.98 (± 0.12) mm edema after 1 h and a 1.06 (±0.16) mm value at 4 h. In general, the intermediate SM treatments (i.e., 100 and 200 mg SM/kg) resulted in dose-related patterns of inhibited edema formation at each of the measured timepoints.
Figure 1. Anti-inflammatory activity of Spondias mombin extract on carrageenan-induced rat paw edema. Rats were randomized in groups (n = 6), fasted for 6 h, and then treated with SM (100, 200, or 400 mg/kg, per os), piroxicam (20 mg/kg, per os), or vehicle. Thirty minutes after the drug administration, 0.1 ml of freshly prepared 1% carrageenan in sterile saline was injected into the subplanter surface of each rat hind paw. The edema produced was estimated by measuring the linear circumference of each paw before and at 1.0, 2.0, 3.0, and 4.0 h after induction of inflammation. SM, Spondias mombin.
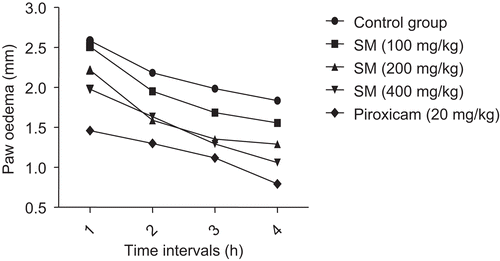
Effect of SM supplementation on LPS-induced TNF-α release in vivo
Compared to untreated mice, the sera of mice treated with LPS for 2 h revealed a very significant induction of TNF-α (P < 0.001). TNF-α production was significantly inhibited in the groups of mice that received oral supplementation of SM (100 and 200 mg/kg, per os) before this LPS treatment (). Mice that were provided the 100 mg SM/kg regimen showed mean LPS-induced serum TNF-α level that was 31.84% lower than that induced in control mice; for the mice treated with 200 mg SM/kg, the levels were on average 67.16% lower than in the LPS-treated controls. The levels of TNF-α noted with the 100 and 200 mg/kg mice groups were also seen to significantly differ from each other.
Figure 2. Effect of SM supplementation on LPS-induced TNF-α release in vivo. Mice were treated daily for three consecutive days with SM (100 or 200 mg/kg, per os). On day 3, 2 h after SM administration, the mice were administered 1 mg LPS/kg (100 µl intraperitoneal injection). Blood was obtained from each mouse retro-orbitally 2 h after the LPS treatment and the serum analyzed for TNF-α via ELISA. Values shown are the mean (±SEM) from n = 5/treatment group. Values that are significantly different from one another are indicated (along with corresponding P value). LPS, lipopolysaccharide; SM, Spondias mombin; TNF-α, tumor necrosis factor-α.
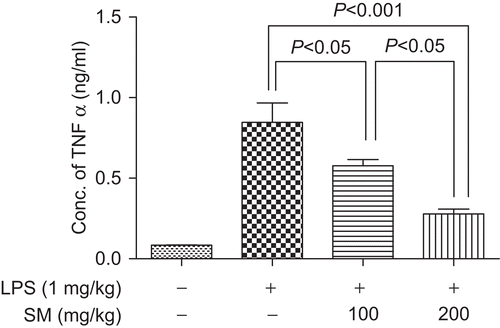
Estimation of viability of SM-treated BM-MØ
The toxicity of SM upon BM-MØ was determined using a modification of the MTT cell viability assay. The assays revealed that BM-MØ viability did not decrease when they were treated with 5–100 µg SM/ml. Instead, it was observed that the SM caused a dose-related increase in the proliferation of the cells (). Specifically, treatment with 5 µg SM/ml led to a 16.01% increase in the OD550 value relative to that of the control cells; treatments with the 25 and 100 µg SM/ml solutions led to values 28.30 and 36.79%, respectively, greater than the controls.
Figure 3. Effect of SM on the viability of bone marrow-derived macrophages (BM-MØ). Cells were cultured with various concentrations of Spondias mombin (SM) for 24 h, and then MTT was added for an additional 4 h. The formazan crystals that formed in the cells were then solubilized with DMSO and the optical density (OD) in the wells was determined at 550 nm as described in the text. Values shown are the mean (±SEM) from n = 3/treatment group. DMSO, dimethyl sulfoxide.
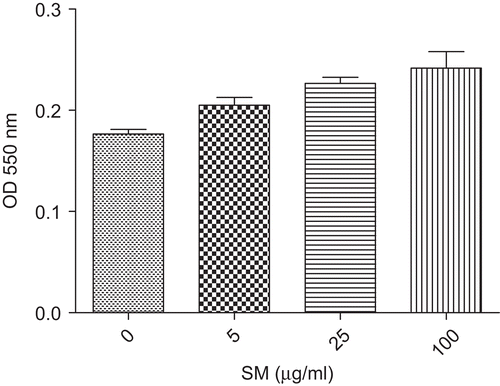
Effect of SM on LPS-induced TNF-α production in vitro
Significant production of TNF-α was observed following treatment of the BM-MØ with 10 µg LPS/ml alone. However, pre-treatment of the cells with SM significantly (P < 0.05) inhibited TNF-α levels in the culture supernatant in a dose-related manner (). Pre-treatment with 5 µg SM/ml resulted in a 22.83% mitigation of the LPS-induced response; treatments with the 25 and 100 µg SM/ml solutions led to inhibitions of 66.68 and 76.35%, respectively.
Figure 4. Effect of SM on LPS-induced TNF-α production in vitro. BM-MØ were pretreated with various concentrations of SM (0, 5, 25, or 100 µg/ml) for 2 h, and then stimulated with 10 µg LPS/ml for 24 h. The supernatant in each culture well was then collected and assayed for TNF-α using cytokine capture ELISA. Values shown are the mean (±SEM) from n = 3/treatment group. *Value is significantly different (P < 0.05) compared with that of the ‘LPS alone’ control treatment. BM-MØ, bone marrow-derived macrophages; LPS, lipopolysaccharide; SM, Spondias mombin; TNF-α, tumor necrosis factor-α.
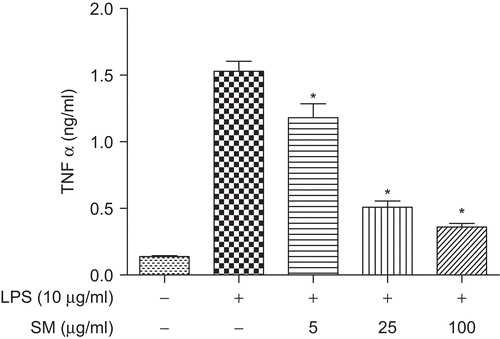
Effect of SM on LPS-induced NO production in vitro
Nitrite that was released from the BM-MØ and then accumulated in the culture medium was measured by the Griess reaction. As illustrated in , the levels of NO in LPS-stimulated control cell cultures were significantly higher than those in normal unstimulated control cells. Akin to what occurred with respect to TNF-α formation/release (see above), LPS-stimulated NO production was inhibited in a concentration-related manner when the BM-MØ were pretreated with 5, 25, and 100 µg SM/ml; these effects were found to be significant (P < 0.05) for the 25 and 100 µg SM/ml treatments. In these studies, pre-treatment with 5 µg SM/ml resulted in a 14.51% mitigation of the LPS-induced response; treatment with 25 and 100 µg SM/ml solutions led to reductions in formation of 33.13 and 39.67%, respectively.
Figure 5. Effect of SM on LPS-induced NO production. BM-MØ were pretreated with various concentrations of SM (0, 5, 25, 100 µg/ml) for 2 h, and then stimulated with 10 µg LPS/ml for 24 h. The supernatant in each culture well was then collected for and assayed for NO using a Griess reagent protocol. Values shown are the mean (±SEM) from n = 3/treatment group. *Value is significantly different (P < 0.05) compared with that of the ‘LPS alone’ control treatment.
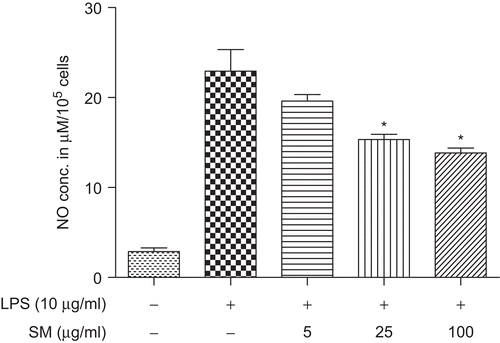
Discussion
The inflammatory response is a complex cascade of steps that include an activation of white blood cells, the release of complement, and the production and release of pro-inflammatory mediators such as cytokines and prostaglandins. The conventional treatments for most inflammatory disorders include therapies that work by reducing or suppressing the release of these pro-inflammatory mediators. Many anti-phlogistic herb-based therapies have also been shown to owe their properties to a suppression of the release of one or more of pro-inflammatory mediators, such as NO, prostaglandins, interleukin-1 (IL-1), IL-6, TNF-α, and/or interferon (Spellman, Citation2006).
Among the many varied uses of SM in traditional medicine is its application in treating inflammatory disorders, infections, and wounds (De Ferreyra, Citation1981; Villegas et al., Citation1997). These earlier observations were the inspiration for these studies of the effects of an SM extract on carrageenan-induced (acute) inflammation in a rat model. The present studies also investigated the effect of oral pre-treatment with the extract on LPS-induced TNF-α release, in vivo. The effect of SM extract on the production and release of inducible nitric oxide (iNO) and TNF-α in the supernatant of LPS-stimulated BM-MØ was also carried out in vitro. LPS is a potent inducer of inflammation in cell lines and systemically in whole animal models. It can promote the secretion of many pro-inflammatory cytokines and mediators. The inflammation model stimulated by LPS is frequently used to evaluate the effect of pharmacological treatment on the inflammatory response (Hong et al., Citation2009; Pearson et al., Citation2010).
In the present studies, the group of Wistar rats pretreated with the methanol extract of SM showed a significant and dose-dependent higher rate of resolution of paw swelling induced by carrageenan over a 4-h period of measurement. Resolution of pain and swelling produced by intraplantar injection of carrageenan is a long-standing and established in vivo model of determining antiphlogistic activities of drugs and putative drug substances (Winter et al., Citation1962). TNF-α is a member of the pro-inflammatory cytokines family and can stimulate the recruitment of neutrophils and monocytes to sites of infection. Increased plasma TNF-α levels during sepsis contribute to host lethality. It has been found that host treatment with TNF-α-neutralizing antibodies yields significant protective effects during an episode of acute sepsis that is usually associated with the release of several pro-inflammatory mediators (Wheeler and Bernard, Citation1999; Raza, Citation2000).
The inhibition of iNO release by activated macrophages by the extract of SM is also an important mechanism in its anti-inflammatory effect. NO is produced by NO synthase (NOS) (Korhonen et al., Citation2005); after exposure to LPS, inducible NOS (iNOS) is induced quantitatively (Duval et al., Citation1996). The role of NO in host defense against microorganisms and tumor cells is well recognized. Nevertheless, excess production of NO is also associated with several diseases, e.g., arthritis, autoimmune diseases, septic shock, as well as in several chronic inflammatory diseases. In these disorders, NO is known to contribute to the inflammation cascade by increasing vascular permeability and extravasations of fluid and proteins at inflammatory sites (Moncada et al., Citation1991; Snyder and Bredt, Citation1992; Guzik et al., Citation2003). As such, the inhibition of high-output NO production has been a therapeutic strategy increasingly used for the treatment of various inflammatory diseases.
With respect to the effects on inducible TNF-α and NO production, the observed suppressive effects were not due to cytotoxicity or systemic toxicity of the extract. Specifically, the acute toxicity studies in mice did not suggest severe untoward effect after oral administration at doses up to 5,000 mg/kg (Lorke, Citation1983). Furthermore, in vitro, the viability of the macrophages was not affected by SM extract at the concentrations used in the study.
The inhibition of inducible TNF-α and NO production seen here to have been caused by the extract of SM provides clues to possible mechanism(s) that could be explain the reported efficacy of the extract in its traditional use against disorders that are characterized by (or contain a significant component of) inflammation. Select phytoconstituents, such as phenols, triterpenes, tannins, anthraquinones, and flavonoids that are present in SM might be responsible for these anti-inflammatory properties. Many of these same constituents have been shown to display other ‘beneficial’ health-related activities, e.g., anti-free radical, antioxidant, and anti-aging (Pauly and Fleury, Citation2002). Several of the flavonoids and other phenolic compounds that were found to be present in the extract of SM have been shown to possess anti-inflammatory activities; one of the mechanisms underlying these immunomodulatory effects appears to be an inhibition of the generation of pro-inflammatory arachidonic acid derivatives and cytokines (Williams et al., Citation1995).
At this time, the specific mechanisms (i.e., at cellular and/or molecular level) utilized by these various SM extract constituents to cause the specific effects observed in the current studies still await delineation.
Conclusion
In this study, the methanolic extract of SM displayed anti-inflammatory properties against carrageenan-induced paw edema in rats, inhibited LPS-induced release of pro-inflammatory TNF-α in vivo, and inhibited iNO and TNF-α formation in vitro among cultures of BM-MØ. These results lend credence to the ethno-medicinal uses of the SM against inflammatory disorders and suggest the involvement of a suppression of pro-inflammatory mediators (such as NO and TNF-α) as a possible mechanism underlying these effects.
Acknowledgement
The authors are grateful to the Alexander von Humboldt Foundation for the postdoctoral fellowship sponsorship to C.S.N., which made the completion of this work possible.
Declaration of interest
The authors report no conflicts of interest. The authors alone are responsible for the content and writing of the paper.
References
- Ademola, I. O., Fagbemi, B. O. and Idowu, S. O. 2005. Anthelmintic activity of extracts of Spondias mombin against gastrointestinal nematodes of sheep: Studies in vitro and in vivo. Trop. Anim. Health Prod. 37:223–235.
- Akubue, P. I., Mittal, G. C. and Aguwa, C. N. 1983. Preliminary pharmacological study of some Nigerian medicinal plants. 1. J. Ethnopharmacol. 8:53–63.
- Ayanwuyi, L. O., Yaro, A. H. and Abodunde, O. M. 2010. Analgesic and anti-inflammatory effects of the methanol stem bark extract of Prosopis africana. Pharm. Biol. 48:296–299.
- Ayoka, A. O., Akomolafe, R. O., Akinsomisoye, O. S., and Ukponmwan, O. E. 2008. Medicinal and economic value of Spondias mombin. Afr. J. Biomed. Res. 11:129–136.
- Corthout, J., Pieters, L., Claeys, M., Geerts, S., Vanden Berghe, D. and Vlietinck, A. 1994. Antibacterial and molluscicidal phenolic acids from Spondias mombin. Planta Med. 60:460–463.
- De Ferreyra, E. C. 1981. Plants that can cure wounds in man and animals. Boletin de Lima 1–12.
- Duval, D. L., Miller, D. R., Collier, J. and Billings, R. E. 1996. Characterization of hepatic nitric oxide synthase: Identification as the cytokine-inducible form primarily regulated by oxidants. Mol. Pharmacol. 50:277–284.
- Guzik, T. J., Korbut, R. and Adamek-Guzik, T. 2003. Nitric oxide and superoxide in inflammation and immune regulation. J. Physiol. Pharmacol. 54:469–487.
- Harborne, J. B. and Harborne, A. J. (Eds.) 1998. Phytochemical Methods: A Guide to Modern Techniques of Plant Analysis. London: Kluwer Academic Publishers.
- Hong, Y. H., Chao, W. W., Chen, M. L. and Lin, B. F. 2009. Ethyl acetate extracts of alfalfa (Medicago sativa L.) sprouts inhibit lipopolysaccharide-induced inflammation in vitro and in vivo. J. Biomed. Sci. 16:64.
- Korhonen, R., Lahti, A., Kankaanranta, H. and Moilanen, E. 2005. Nitric oxide production and signaling in inflammation. Curr. Drug Targets. Inflamm. Allergy. 4:471–479.
- Lin, H., Chen, C. and Chen, B. D. 2001. Resistance of bone marrow-derived macrophages to apoptosis is associated with the expression of X-linked inhibitor of apoptosis protein in primary cultures of bone marrow cells. Biochem. J. 353:299–306.
- Lorke, D. 1983. A new approach to practical acute toxicity testing. Arch. Toxicol. 54:275–287.
- Moncada, S., Palmer, R. M. and Higgs, E. A. 1991. Nitric oxide: Physiology, pathophysiology, and pharmacology. Pharmacol. Rev. 43:109–142.
- Mosmann, T. 1983. Rapid colorimetric assay for cellular growth and survival: Application to proliferation and cytotoxicity assays. J. Immunol. Methods 65:55–63.
- Musa, Y. M., Haruna, A. K., Ilyas, M., Yaro, A. H., Ahmadu, A. A. and Usman, H. 2007. Phytochemical, analgesic and anti-inflammatory effects of the ethylacetate extract of the leaves of Pseudocedrella kotschyii. Afr J Tradit Complement Altern Med. 2008; 5(1):92–96.
- Njoku, P. C. and Akumefula, M. I. 2007. Phytochemical and nutrient evaluation of Spondias mombin leaves. Pakistan J. Nutr. 6:613–615.
- Nworu, C. S., Akah, P. A., Okoli, C. O., and Okoye, T. C. 2007. Oxytocic activities of leaf extract of Spondias mombin (Anacardiaceae). Pharm. Biol. 45:366–371.
- Offiah, V. N. and Anyanwu, I. I. 1989. Abortifacient activity of an aqueous extract of Spondias mombin leaves. J. Ethnopharmacol. 26:317–320.
- Onwuka, C. F. 1992. Hydrocyanic acid contents of tropical browse and their influence on performance of goats. Food Chem. Essex 45:5–10.
- Oser, B. L. and Oser, M. 1956a. Nutritional studies on rats on diets containing high levels of partial ester emulsifiers. I. General plan and procedures; growth and food utilization. J. Nutr. 60:367–390.
- Oser, B. L. and Oser, M. 1956b. Nutritional studies on rats of diets containing high levels of partial ester emulsifiers. II. Reproduction and lactation. J. Nutr. 60:489–505.
- Pauly, G. and Fleury, M. 2002. Cosmetic containing plant extracts. Official Gazette of US Patents and Trademark Office Patents 1259(3).
- Pearson, W., Fletcher, R. S., Kott, L. S. and Hurtig, M. B. 2010. Protection against LPS-induced cartilage inflammation and degradation provided by a biological extract of Mentha spicata. BMC Complement. Altern. Med. 10:19.
- Raza, A. 2000. Anti-TNF therapies in rheumatoid arthritis, Crohn’s disease, sepsis, and myelodysplastic syndromes. Microsc. Res. Tech. 50:229–235.
- Snyder, S. H. and Bredt, D. S. 1992. Biological roles of nitric oxide. Sci. Am. 266:68–71, 74.
- Spellman, K. 2006. Modulation of cytokine expression by traditional medicines: A review of herbal immunomodulators. Altern. Med. Rev. 11:128–150.
- Villegas, L. F., Fernández, I. D., Maldonado, H., Torres, R., Zavaleta, A., Vaisberg, A. J. and Hammond, G. B. 1997. Evaluation of the wound-healing activity of selected traditional medicinal plants from Perú. J. Ethnopharmacol. 55:193–200.
- Weischenfeldt, J. and Porse, B. 2008. Bone marrow-derived macrophages (BMM): Isolation and applications. Cold Spring Harbor Protocols. <http://cshprotocols.cshlp.org/cgi/content/full/2008/13/pdb.prot5080>.
- Wheeler, A. P. and Bernard, G. R. 1999. Treating patients with severe sepsis. New Engl. J. Med. 340:207–214.
- Williams, C. A., Hoult, J. R., Harborne, J. B., Greenham, J., and Eagles, J. 1995. A biologically active lipophilic flavonol from Tanacetum parthenium. Phytochemistry 38:267–270.
- Williams, J., Odum, J., Lewis, R. W. and Brady, A. M. 1997. The oral administration of polysorbate 80 to the immature female rat does not increase uterine weight. Toxicol. Lett. 91:19–24.
- Winter, C. A., Risley, E. A. and Nuss, G. W. 1962. Carrageenin-induced edema in hind paw of the rat as an assay for anti-iflammatory drugs. Proc. Soc. Exp. Biol. Med. 111:544–547.