Abstract
Immunosuppressive environmental chemicals may increase the potency of allergens and thereby play a role in the development of allergic diseases. This study’s primary objective was to examine the mechanisms behind the relationship between allergic diseases and the immunosuppression induced by some environmental chemicals. We focused on the modulation of allergic potential in vitro and in mice by the organophosphorus pesticide O,O-diethyl-O-4-nitrophenyl-thiophosphate (parathion) and the organochlorine pesticide 1,1,1-trichloro-2,2-bis(4-methoxy-phenyl)ethane (methoxychlor), with respect to the TH1-type allergen 2,4-dinitrochlorobenzene (DNCB) and the TH2-type allergen trimellitic anhydride (TMA). Mice (4-week-old) were orally administered parathion or methoxychlor. Four weeks after the final dosing, the mice were sensitized to DNCB or TMA, and T-lymphocyte proliferation measured in their (using a local lymph node assay [LLNA]). In addition, we analyzed T-lymphocytes via surface antigen expression and local cytokine production in auricular lymph nodes after treatment with 0.1% DNCB or 0.3% TMA. The estimated concentration of DNCB and TMA to yield a stimulation index (SI) of cell proliferation of three decreased markedly in parathion- and methoxychlor-pre-treated mice. Pesticide pre-treatment induced marked increases in the number of helper and cytotoxic T-cells, levels of TH1 and TH2 cytokines, and gene expression in lymph node cells. According to our results, TH1- and TH2-type allergies are aggravated by prior exposure to immunosuppressive environmental chemicals.
Abbreviations: | ||
ANOVA, | = | analysis of variance; |
DNCB, | = | 2,4-dinitrochlorobenzene; |
FITC, | = | fluorescein isothiocyanate; |
[3H]-TdR, | = | [3H]-methyl thymidine; |
IFNγ, | = | interferon-γ; |
IL, | = | interleukin; |
LLNA, | = | local lymph node assay; |
LN, | = | lymph node; |
MHC, | = | major histocompatibility complex; |
OC, | = | organochlorine; |
OP, | = | organophosphorus; |
PBS, | = | phosphate-buffered saline; |
PE, | = | phycoerythrin; |
PS, | = | phosphatidylserine; |
SI, | = | stimulation index; |
TMA, | = | trimellitic anhydride; |
TNFα, | = | tumor necrosis factor-alpha; |
TH, | = | T-helper |
Introduction
Several epidemiologic studies have suggested that the prevalence of allergic disease has increased rapidly, mainly among children and adolescents, over the past several decades (Beasley et al., Citation2003; Yanagisawa et al., Citation2008). The causes of allergy include genetic factors (e.g., host susceptibility) and environmental factors (e.g., allergen load, environmental pollutants; Burney et al., Citation1990; Peat and Li, Citation1999; Yanagisawa et al., Citation2008). Environmental chemicals may increase the potency of allergens and thereby play a role in the development of allergic diseases (Casillas et al., Citation1999; Yanagisawa et al., Citation2008).
Current evidence suggests that the dysregulation of immune function caused by several types of environmental chemicals lead to a variety of immune disorders, including immunodeficiency, allergies, and autoimmunity (Sobel et al., Citation2005; Zhang et al., Citation2005; Narita et al., Citation2007; Wang et al., Citation2007; Ward et al., Citation2009; Xu et al., Citation2010). Therefore, in a previous study, we focused on the organophosphorus pesticide parathion and organochlorine pesticide methoxychlor which were both known to play roles in the suppression of immune functions (Wiltrout et al., Citation1978; Casale et al., Citation1983, Citation1984; Chapin et al., Citation1997; Staples et al., Citation1998; Takeuchi et al., Citation2002; Fukuyama et al., Citation2010b and Citation2010c), and demonstrated that prior oral exposure to methoxychlor and parathion aggravated T-lymphocyte–mediated allergic reactions, including results of a local lymph node assay (LLNA), a thymidine uptake test used to screen chemicals for their potential to cause dermal hypersensitivity (Fukuyama et al., Citation2010d).
Environmental chemicals associated with immune disorders are thought to belong to at least one of three general categories of mechanisms (Rao and Richardson, Citation1999; Sobel et al., Citation2005): (i) altering the self-antigen such that it appears foreign to the immune system; (ii) preventing the central tolerance of autoreactive T- or B-lymphocytes; and, (iii) altering gene expression. Hormones such as estrogens and environmental chemicals that behave like estrogens are thought to belong to this last category (Grimaldi et al., Citation2002), and several studies linking environmental chemicals with immune disorders have noted the chemicals’ estrogenic character (Sobel et al., Citation2005; Ward et al., Citation2009; Xu et al., Citation2010). Our goal was to distinguish between these categories of mechanism. In our previous study, because four weeks of recovery time was left between oral exposure to environmental chemicals (parathion or methoxychlor) and allergen sensitization, parathion and methoxychlor could not act as the self-antigen. In terms of relativity of estrogenic character, we elicited positive reactions using parathion, which is not a hormone disruptor.
Based on the parathion and methoxychlor studies described above, we hypothesize that the breakdown of the central tolerance of autoreactive T- or B-lymphocytes is strongly related to abnormal hypersensitivity. In this paper, we attempt to clarify the mechanisms of the relationship between allergy and the breakdown of central tolerance by using uptake of [3H]-methyl thymidine ([3H]-TdR) (via the LLNA), numbers of CD4+ and CD8+ T-lymphocytes, local TH1- and TH2-type cytokine production, gene expression in lymph nodes, and the environmental chemicals methoxychlor, parathion, the TH1-type sensitizer 2,4-dinitrochlorobenzene (DNCB), and the TH2-type sensitizer trimellitic anhydride (TMA). Exposure to TMA is recognized as a leading cause of occupational asthma worldwide. Several reports (Arts et al., Citation2003; Plitnick et al., Citation2003; Vanoirbeek et al, Citation2003; Ban et al, Citation2006; Fukuyama et al., Citation2008a and Citation2008b, Citation2009, Citation2010a) have shown that dermal TMA exposure increases a TH2 cytokine secretion pattern in mice. In contrast, identical dermal exposure to DNCB, which causes delayed-type hypersensitivity reactions but lacks the potential to cause respiratory hypersensitivity, elicits the TH1-type cytokine secretion profile (Larsen et al., Citation2001; Dearman et al., Citation2005; Matheson et al., Citation2005a and Citation2005b; Vanoirbeek et al., 2006; Fukuyama et al., Citation2008a and Citation2008b, Citation2009, Citation2010a). These low-molecular weight chemicals are well-known human allergens (Larsen et al., Citation2001; Matheson et al., Citation2005a and Citation2005b) that have been used extensively in experimental research on chemical hypersensitivity.
Materials and methods
Chemicals
Standard methoxychlor (C16H15Cl3O2, > 97% pure), standard parathion (C10H14NO5PS, 99.5% pure), acetone, and olive oil were purchased from Wako Pure Chemical Industries, Ltd. (Osaka, Japan). TMA and DNCB were purchased from Tokyo Kasei Kogyo Co., Ltd. (Tokyo, Japan). Corn oil was purchased from Hayashi Chemicals (Tokyo, Japan). The caspase-3 inhibitors Z-VAD-FMK and Ac-DEVD-CHO were purchased from Promega (Tokyo, Japan). For the in vitro studies, parathion and methoxychlor were dissolved and then diluted in DMSO or acetone, respectively, to 0.5% (w/v). We selected for study three concentrations of these pesticides based upon the results of cell viability analyses (data not shown); the exact concentrations chosen were based on each agent’s ability to substantially inhibit cell viability, yet not to induce 100% lethality in either of the two cell types. For in vivo oral administration, methoxychlor and parathion were diluted in corn oil. TMA and DNCB were dissolved in a 4:1 acetone:olive oil mixture. The maximum doses used in this study were selected to avoid systemic toxicity or excessive local sensitization (particularly in the preliminary test; data not shown). The doses used are shown in .
Table 1. Chemical doses used.
Cell culture
The human acute T-cell leukemia cell line (Jurkat E6.1) and the mice lymphoma cell line (EL4) were obtained from DS Pharma Biomedical Co., Ltd. (Tokyo, Japan). Jurkat E6.1 cells were cultured in 260-mL EasYFlasks (Nalge Nunc International K.K., Tokyo, Japan) in 25 mL of RPMI 1640 (Gibco, Tokyo, Japan) supplemented with 10% heat-inactivated fetal calf serum (FCS; Gibco) at 37°C in a 5% CO2 atmosphere. EL4 cells were cultured in the same 260-mL EasYFlasks (Nalge Nunc International K.K.) in 25 mL of Dulbecco’s modified Eagle medium supplemented with 10% FCS (DS Pharma Biomedical Co., Ltd.) at 37°C in a 5% CO2 atmosphere. The medium was changed two to three times per week for both cell lines.
Animals
Female BALB/c mice (3-week-old) were purchased from Charles River Japan Laboratories (Atsugi, Kanagawa, Japan) and housed individually under controlled lighting (lights on from 0700 to 1900 h), temperature (22 ± 3°C), humidity (55 ± 15%), and ventilation (at least 10 complete fresh-air changes hourly). Food (Certified Pellet Diet MF; Oriental Yeast Co., Tokyo, Japan) and water were available ad libitum. The current study was conducted in accordance with the Code of Ethics for Animal Experimentation of the Institute of Environmental Toxicology.
In vitro study (confirmation of parathion- and methoxychlor-induced apoptosis)
The Jurkat E6.1 and EL4 cells were plated into two sets of plates (one set for each test agent) at densities of 5 × 105 cells/mL, respectively. Duplicate plates per dose per agent were treated with the following doses: parathion (3 × 10−8, 1 × 10−7, or 3 × 10−7 M), or methoxychlor (1 × 10−8, 3 × 10−8, or 1 × 10−7 M). Immediately after initiation of the chemical treatment, parallel sets of plates of agent-treated cells were also treated with the caspase-3 inhibitors Z-VAD-FMK (2 × 10−5 M) or Ac-DEVD-CHO (10−5 M). Control cell cultures were untreated (i.e., received vehicle-bearing medium only). In all cases, the plates were then incubated for 4 h at 37°C under 5% CO2. At the end of exposure, the culture medium (containing all the non-adherent cells that were originally seeded, live as well as dead) from each given plate was transferred to a 5-mL tube and then used for analysis of Annexin-V and caspase-3 expression, and amount of DNA fragmentation. All experiments were conducted twice, thereby yielding quadruplicate data for each variable (agent dose with or without caspase-3 inhibitors) were analyzed.
Apoptosis assay
To assess apoptosis, we measured the proportion of Annexin-V+ and caspase-3+ cells, and the extent of DNA fragmentation in both cell lines. It has been reported that Annexin-V+ staining can detect apoptosis at an early stage, visualizing alterations of the cell membrane resulting from movement of phosphatidylserine residues to the external leaflet of the plasma membrane (Morris et al., Citation1984; Vermes et al., Citation1995). A later-stage marker of apoptosis is DNA fragmentation. Apoptosis has been characterized biochemically by the production of internucleosomal DNA fragments of 180–200 bp, resulting from the activation of an endonuclease. Caspase activation is a required step in the apoptotic pathway and caspase-3 was the first caspase linked to apoptosis (Gurtu et al., Citation1997).
To perform flow cytometric analysis of apoptotic cell, cells were stained with fluorescein isothiocyanate (FITC)-conjugated Annexin-V, FITC-conjugated caspase-3, and propidium iodide. To assay the cells, we used an Annexin-V:FITC Apoptosis Detection Kit I and FITC Active Caspase-3 Apoptosis Kit (BD Pharmingen, San Diego, CA) in accordance with the manufacturer’s protocol. For each sample, 10,000 events were recorded and analyzed.
The FastPure DNA Kit (Takara Bio, Inc., Shiga, Japan) was used to extract DNA from aliquots of 3 × 105 cells in accordance with the manufacturer’s protocol. To measure DNA fragmentation, DNA samples were electrophoretically separated over 2% agarose gels (Reliant® Gel System; Lonza, Verviers, Belgium) in 90 mM Tris-boric acid-EDTA solution (Nippon Gene, Tokyo, Japan) at 8.5 V/cm for 2 h. After electrophoresis, the gels were stained with SYBR® Green I (Takara Bio, Inc.), and the nucleic acids were visualized with a fluoroimager (Ettan DIGE; GE Healthcare Bio-Sciences Corp., Tokyo, Japan).
In vivo study (LLNA)
After a 1-week acclimatization period, BALB/c mice (4-week-old) were allocated randomly to dose and control groups for each chemical (methoxychlor; 0, 30, or 300 mg kg−1 day−1, or parathion; 0, 0.15, or 1.5 mg kg−1 day−1, n = 20 per dose). On Days 1–5, each mouse received orally an aliquot of the test solution or solvent alone (corn oil). About 4 week after the final oral administration (Day 31), the LLNA was performed as described by Kimber and Weisenberger (Citation1989), with minor modifications. Mice were allocated randomly to dose and control groups for each chemical (TMA; 0, 0.1, 0.3, and 1.0%, or DNCB; 0, 0.03, 0.1, and 0.3%, n = 5 per dose). A 25-µL aliquot of test solution or solvent (4:1 acetone:olive oil) only was applied daily to the dorsum of each ear of each mouse for three consecutive days (Days 31–33).
On Day 36, [3H]-TdR (20 µCi/animal; Perkin Elmer Japan, Tokyo, Japan) was injected via the tail vein into all test and control mice. At 5 h after the injection, the mice were euthanized with pentobarbital sodium (75 mg/kg, IP), and the auricular lymph nodes on both sides of each mouse were removed and pooled (by mouse) in phosphate-buffered saline (PBS, pH 7.4; Gibco). Single-cell suspensions of lymph nodes in 5 mL of PBS were prepared by passage through sterile 70-µm nylon cell strainers (Falcon, Tokyo, Japan). The lymph node cell suspension was washed twice with an excess of PBS, and the cell pellet was incubated in 3 mL of 5% trichloroacetic acid (Wako) at 4°C for ~18 h. Each cell pellet was re-suspended in 1 mL of trichloroacetic acid and transferred to 9 mL of scintillation fluid (AtomLight, Perkin Elmer Japan). Incorporation of [3H]-TdR was measured with a β-scintillation counter (LC-6100; Aloka, Tokyo, Japan) as disintegrations per minute for each mouse.
Stimulation indexes (SIs) and EC3 values were calculated from [3H]-TdR incorporation data. The SI was calculated by dividing the mean [3H]-TdR incorporation value for each treatment group by that of the control group. The EC3 value is an estimate of the amount of test solution required to induce an SI of 3 (Basketter et al., Citation1999). In the standard LLNA, the criterion for a positive response is an SI of 3 or greater (Dearman et al., Citation1999).
In vivo study (preparation for detailed analysis of hypersensitivity)
The oral administration and dermal sensitization protocol and procedure for the detailed analysis were the same as those for the LLNA. After a 1-week acclimatization period, BALB/c mice (4-week-old) were allocated randomly to dose and control groups for pre-treatment with methoxychlor or parathion (methoxychlor; 0, 30, or 300 mg kg−1 day−1, or parathion; 0, 0.15, or 1.5 mg kg−1 day−1, n = 18 per dose). About 4 weeks after the last oral pre-treatment (Day 31), mice were allocated randomly to dose and control groups for the test chemicals DNCB or TMA (TMA; 0 and 0.3%, or DNCB; 0 and 0.1%, n = 6 per dose). To confirm the TH1 and TH2 allergenicity of the test chemicals, we used a sensitization dose at approximately the same levels of the EC3 values (see ). On Day 36, the mice were euthanized with pentobarbital sodium (75 mg/kg), and the lymph nodes on both sides of each mouse were removed and pooled (by mouse) in PBS. For cell counts, flow cytometry, RNA analysis and cell culture, single-cell suspensions of lymph nodes in 1 mL RPMI 1640 supplemented with 5% FCS were prepared by passage through sterile 70-µm nylon cell strainers. The number of lymph node cells was determined with a Coulter Z2 counter.
To perform flow cytometric analyses, lymphocytes were stained with FITC-conjugated hamster anti-mouse CD3 (clone 145-2C11), phycoerythrin (PE)-conjugated rat anti-mouse CD4 (clone RM4-5), and/or PE-Cy5-conjugated rat anti-mouse CD8 (clone 53-6.7). All antibodies for flow cytometric analyses were purchased from BD Pharmingen. To avoid non-specific binding, 1 × 106 cells were pre-incubated with 20% normal goat serum (Sigma, St. Louis, MO) for 10 min at 4°C; this was followed by incubation with the various FITC-, PE-, and PE-Cy5-conjugated monoclonal antibodies for 30 min at 4°C in the dark. The cells were then washed twice with FCS-PBS, re-suspended at 1 × 106 cells/tube in 1 mL of PBS, and then analyzed in a FACSCaliber flow cytometer (BD Pharmingen) using the Cell Quest program (BD Pharmingen). For each sample, 10,000 events were recorded and analyzed for the expression of antigens.
Cytokine production
To stimulate T-cell-receptor signaling, we cultured single-cell suspensions of LN (1 × 106 cells/well) with anti-CD3 (2 µg/mL) and/or anti-CD28 (2 µg/mL) (BD Pharmingen) T-lymphocyte antibodies for 24 or 72 h in 24-well plates at 37°C in a 5% CO2 atmosphere. Tumor necrosis factor-α (TNFα), interferon-γ (IFNγ), and interleukins (ILs)-4, -5, -13, and -17 in the supernatants were assayed by BD Cytometric Bead Array (BD Pharmingen) in accordance with the manufacturer’s protocol. Briefly, the supernatants (50 µl) and cytokine beads (50 µl) were each added to a 5 mL tube and the tubes incubated for 1 h at room temperature. PE-conjugated anti-mouse cytokine antibody (50 µl) was added and each tube was incubated a further 1 h at room temperature. The cells were then washed with wash buffer, re-suspended in 300 µl of wash buffer, and then analyzed in a FACSCaliber flow cytometer using the Cell Quest program. For each sample, 300 events were recorded and analyzed for the expression of cytokines.
TNFα, IFNγ, and IL-17 in the supernatants were quantified after culture for 24 h in the presence of anti-CD3 antibody. IL-13 in the supernatants was quantified after culture for 72 h in the presence of anti-CD3. IL-4 in the supernatants was quantified after culture for 24 h in the presence of anti-CD3 and anti-CD28. IL-5 in the supernatants was quantified after culture for 72 h in the presence of anti-CD3 and anti-CD28.
Gene expression
Total RNA in the cell pellet (1 × 105 cells/mouse) was extracted and reverse transcribed into complementary DNA (cDNA) by using Taqman® Fast Cells-to-CT™ Kit (Applied Biosystems, Tokyo, Japan) according to the manufacturer’s protocol. Polymerase chain reaction (PCR) primers for the genes encoding the following proteins were purchased from Takara Bio, Inc.: T-box expressed in T-cells (T-bet), retinoic acid-related orphan receptor-γt (ROR-γt), GATA binding protein 3 (GATA-3), and glyceraldehyde-3-phosphate dehydrogenase (GAPDH). The corresponding cDNAs were amplified by quantitative real-time PCR by using the Thermal Cycler Dice system (Takara Bio, Inc.). The data acquired for each sample were normalized to expression levels recorded for the housekeeping gene GAPDH.
Statistical analysis
The data were transformed logarithmically to equalize the variance, and analysis of variance (ANOVA) was used to evaluate the results. For any statistically significant ANOVA outcomes, the differences between groups were assessed by a Dunnett’s multiple comparison test. A value of p < 0.05 was considered to indicate statistical significance.
Results
In vitro study (confirmation of parathion- and methoxychlor-induced apoptosis)
We performed in vitro analysis to evaluate the extent of apoptosis resulting from parathion or methoxychlor treatment (). In both Jurkat E6.1 and EL4 cells, the proportion of Annexin-V+ cells increased significantly (p < 0.01) relative to the controls in the parathion (0.3 × 10−7 and 1 × 10−7 M) and methoxychlor (1 × 10−7 and 3 × 10−7 M) groups. The proportion of caspase-3+ cells increased significantly (p < 0.01) relative to the controls in the parathion (1 × 10−7 M) and methoxychlor (3 × 10−7 M) groups. Because it has been observed that oligonucleosomal cleavage accompanies apoptosis in most systems, we assessed the degradation patterns of nuclear DNA during chemically induced cell deaths by using agarose gel electrophoresis. In the parathion (1 × 10−7 M), and methoxychlor (1 × 10−7 and 3 × 10−7 M) treatment groups in both Jurkat E6.1 and EL4 cells, the fraction of DNA 300–500 bp in length in ladder form increased. In contrast, inhibitors of caspase-3 (Z-VAD-FMK and Ac-DEVD-CHO) substantially protected both Jurkat E6.1 and EL4 cells from cell death associated with treatment with parathion or methoxychlor.
Figure 1. Annexin-V (A: Jurkat T-cells; D: EL4 cells) and caspase-3 (B: Jurkat T-cells; E: EL4 cells) expression, and amount of DNA fragmentation (C: Jurkat T-cells; F: EL4 cells) in cells treated with parathion and methoxychlor. Annexin-V and caspase-3 expression values are expressed as mean (%) ± SD (n = 4 per group). Statistical significance indicated by asterisks: *p < 0.05 and **p < 0.01 compared with the control value (Dunnett’s multiple comparison test); Φp < .05 and ΦΦp < 0.01 compared with the high-dose treatment group (Dunnett’s multiple comparison test). For DNA fragmentation, molecular weight markers are shown on the left in C and F.
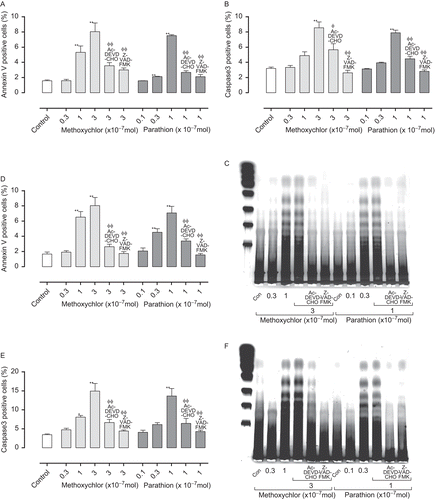
In vivo study (LLNA)
To evaluate the extent to which prior oral exposure to parathion and methoxychlor increase sensitivity to TMA and DNCB, we measured incorporation of [3H]-TdR—an indicator of cell proliferation—in auricular lymph nodes (LLNA, ). In the TMA group pre-treated with parathion, pre-treatment with solvent only and parathion (0.15 mg/kg) induced a positive [3H]-TdR incorporation response (defined as SI ≥ 3) in the 1% TMA sensitization group. Pre-treatment with 1.5 mg/kg parathion was associated with a positive response in the 0.1%, 0.3%, and 1% sensitization groups. EC3 values of the TMA group pre-treated with 0, 0.15, and 1.5 mg parathion/kg were 0.52%, 0.18%, and 0.04%, respectively.
Figure 2. Incorporation of [3H]-TdR in auricular lymph node in (A) TMA pre-treated with parathion, (B) DNCB pre-treated with parathion, (C) TMA pre-treated with methoxychlor, and (D) DNCB pre-treated with methoxychlor, in local lymph node assay. Incorporation of [3H]-TdR values are expressed as mean (dpm) ± SD (n = 5 per group). Statistical significance indicated by asterisks: *p < 0.05, **p < 0.01 compared with the vehicle control group (Dunnett’s multiple comparison test). DNCB, 2,4-dinitrochlorobenzene; TMA, trimellitic anhydride. [3H]-TdR, [3H]-methyl thymidine
![Figure 2. Incorporation of [3H]-TdR in auricular lymph node in (A) TMA pre-treated with parathion, (B) DNCB pre-treated with parathion, (C) TMA pre-treated with methoxychlor, and (D) DNCB pre-treated with methoxychlor, in local lymph node assay. Incorporation of [3H]-TdR values are expressed as mean (dpm) ± SD (n = 5 per group). Statistical significance indicated by asterisks: *p < 0.05, **p < 0.01 compared with the vehicle control group (Dunnett’s multiple comparison test). DNCB, 2,4-dinitrochlorobenzene; TMA, trimellitic anhydride. [3H]-TdR, [3H]-methyl thymidine](/cms/asset/9ed0441c-5716-47ad-a22e-9285fc6c17cf/iimt_a_566231_f0002_b.gif)
In the DNCB group pre-treated with parathion, pre-treatment with solvent only induced a positive response in the 0.1% and 0.3% sensitization groups. Parathion pre-treatment (0.15 and 1.5 mg parathion/kg) was associated with a positive response in the 0.03%, 0.1%, and 0.3% sensitization groups. EC3 values of the DNCB group pre-treated with 0, 0.15, and 1.5 mg parathion/kg were 0.07%, 0.03%, and 0.02%, respectively.
In the TMA group pre-treated with methoxychlor, pre-treatment with solvent only and 30 mg methoxychlor/kg was associated with a positive response in the 1% sensitization groups. Pre-treatment with 300 mg methoxychlor/ kg was associated with a positive response in the 0.1%, 0.3%, and 1% sensitization groups. EC3 values of the TMA group pre-treated with 0, 0.15, or 1.5 mg parathion/ kg were 0.42%, 0.30%, and 0.04%, respectively.
In the DNCB group pre-treated with methoxychlor, pre-treatment with solvent only induced a positive response in the 0.1% and 0.3% sensitization groups. Methoxychlor pre-treatment (30 and 300 mg/kg) was associated with a positive response in the 0.03%, 0.1%, and 0.3% sensitization groups. EC3 values of the DNCB group pre-treated with 0, 30, or 300 mg parathion/kg were 0.10%, 0.02%, and 0.02%, respectively.
In vivo study (preparation for detailed analysis of hypersensitivity)
We performed detailed analysis of hypersensitivity, including the helper (CD4+CD8−) and cytotoxic (CD4−CD8+) T-lymphocyte counts (), TH1 (IFNγ, TNFα, and IL-17; ) and TH2 (IL-4, IL-5 and IL-13; ) cytokine production, and the gene expression (T-bet, ROR-γt and GATA-3; ) in lymph nodes of mice treated with 0.3% TMA and 0.1% DNCB. The total number of lymphocytes were increased in parallel with the number of T-lymphocyte counts (data not shown). Groups pre-treated with parathion or methoxychlor, acetone and olive oil vehicle alone (without TMA or DNBC) produced no increase in any of the parameters studied. In the TMA group pre-treated with 1.5 mg parathion/kg, cytotoxic T-lymphocyte counts, IFNγ and TNFα production, TH2 cytokine production, and GATA-3 gene expression were significantly increased relative to control group levels (i.e., pre-treated with 0 mg parathion/kg). Helper and cytotoxic T-lymphocyte counts, IFNγ, TNFα, and IL-4 production in the 0.15-mg parathion/kg pre-treatment group were significantly increased relative to control group levels (i.e., pre-treated with 0 mg parathion/kg).
Figure 3. Helper (CD4+CD8−) (A: parathion; B: methoxychlor) and cytotoxic (CD4−CD8+) (C: Parathion; D: Methoxychlor) T-lymphocyte counts in auricular lymph nodes of mice treated with test solution. Helper- and cytotoxic T-lymphocyte counts are expressed as mean (× 105 cells) ± SD (n = 6 per group). Statistical significance indicated by asterisks: *p < 0.05, **p < 0.01 compared with the vehicle control group (Dunnett’s multiple comparison test). AOO, acetone + olive oil, DNCB, 2,4-dinitrochlorobenzene; TMA, trimellitic anhydride.
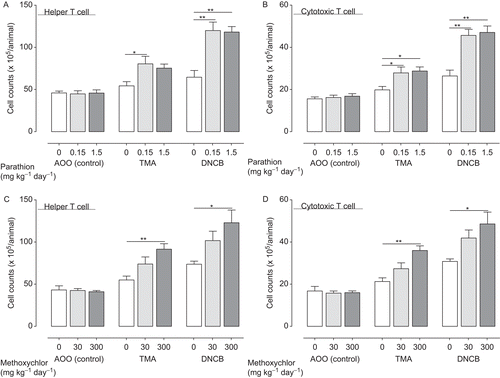
Figure 4. TH1 cytokine production (IFNγ (A: parathion; D: methoxychlor), TNFα (B: Parathion; E: Methoxychlor), IL-17 (C: parathion; F: methoxychlor)) in auricular lymph nodes of mice treated with test solution. TH1 cytokine production values are expressed as mean (pg/ mL) ± SD (n = 6 per group). Statistical significance is indicated by asterisks: *p < 0.05, **p < 0.01 compared with the vehicle control group (Dunnett’s multiple comparison test). AOO, acetone + olive oil; IFNγ, interferon-γ; IL, interleukin; TMA, trimellitic anhydride; DNCB, 2,4-dinitrochlorobenzene; TNFα, tumor necrosis factor-α.
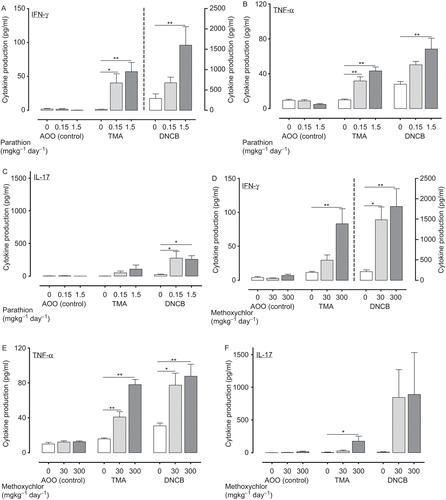
Figure 5. TH2 cytokine production (IL-4 (A: parathion; D: methoxychlor), IL-5 (B: parathion; E: methoxychlor), IL-13 (C: parathion; F: methoxychlor)) in auricular lymph nodes of mice treated with test solution. TH2 cytokine production values are expressed as mean (pg/mL) ± SD (n = 6 per group). Statistical significance is indicated by asterisks: *p < 0.05, **p < 0.01 compared with the vehicle control group (Dunnett’s multiple comparison test). AOO, acetone + olive oil; DNCB, 2,4-dinitrochlorobenzene; IL, interleukin; TMA, trimellitic anhydride.
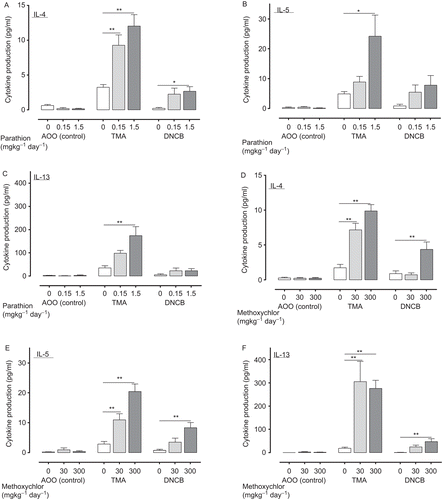
Figure 6. Expression of genes (T-bet (A: parathion; D: methoxychlor), GATA-3 (B: parathion; E: methoxychlor), ROR-γt (C: parathion; F: methoxychlor)) in auricular lymph nodes of mice treated with test solution. Gene expression values are represented the increase in expression compared to the value for the expression of GAPDH, and are expressed as mean ± SD (n = 6 per group). Statistical significance indicated by asterisks: *p < 0.05, **p < 0.01 compared with the vehicle control group (Dunnett’s multiple comparison test). PCR bands in photographs of the gels were visualized with a fluoroimager (Ettan DIGE) with 32, 35, and 35 amplification cycles for T-bet, ROR-γt and GATA-3, respectively. AOO, acetone + olive oil; DNCB, 2,4-dinitrochlorobenzene; GAPDH, glyceraldehyde-3-phosphate dehydrogenase; GATA 3, GATA binding protein 3; PCR, polymerase chain reaction; ROR-γt, retinoic acid-related orphan receptor-γt; T-bet, T-box expressed in T-cells; TMA, trimellitic anhydride.
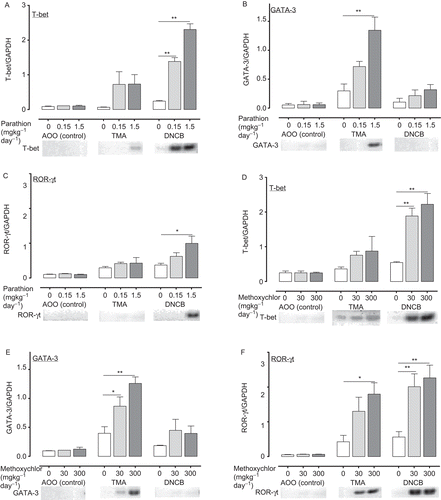
In the DNCB group pre-treated with 1.5 mg parathion/kg, helper and cytotoxic T-lymphocyte counts, TH1 cytokine production, IL-4 production, and both T-bet and ROR-γt gene expression were significantly increased relative to control group levels (i.e., pre-treated with 0 mg parathion/kg). Helper and cytotoxic T-lymphocyte counts, IL-17 production, and T-bet gene expression were significantly increased relative to control group levels (i.e., pre-treated with 0 mg parathion/kg).
In the TMA groups pre-treated with 300 mg methoxychlor/kg, helper and cytotoxic T-lymphocyte counts, TH1 and TH2 cytokine production, and T-bet, GATA-3, and ROR-γt gene expression were significantly increased relative to control group levels (i.e., pre-treated with 0 mg methoxychlor/kg); in the 30 mg methoxychlor/kg pre-treatment group, TNFα and TH2 cytokine production, and GATA-3 gene expression were significantly increased relative to control group levels (i.e., pre-treated with 0 mg methoxychlor/kg).
In the DNCB groups pre-treated with 300 mg methoxychlor/kg, helper and cytotoxic T-lymphocyte counts, IFNγ, TNFα, and TH2 cytokine production, and both T-bet and ROR-γt gene expression were significantly increased relative to control group levels (i.e., pre-treated with 0 mg methoxychlor/kg); in the 30 mg methoxychlor/ kg pre-treatment group, IFNγ and TNFα production, and both T-bet and ROR-γt gene expression were significantly increased relative to control group levels (i.e., pre-treated with 0 mg methoxychlor/kg).
Discussion
In this study, we focused on the disorder of hypersensitivity induced by two immunosuppressive environmental chemicals (parathion and methoxychlor) and attempted to clarify the mechanisms that produce these disorders. We studied via several types of detection methods including LLNA, numbers of CD4+ and CD8+ T-lymphocytes, local TH1- and TH2-type cytokine production, gene expression in lymph nodes, and the effects of these chemicals on reactions to TH1- and TH2-type allergens (i.e., DNCB and TMA). This study shows that prior oral exposure of mice to the immunosuppressive environmental chemicals parathion and methoxychlor aggravates TH1- and TH2-type allergy in mice. The aggravating effects are paralleled by those seen in uptake of [3H]-TdR (LLNA), numbers of CD4+ and CD8+ T-lymphocytes, local TH1- and TH2-type cytokine production, and expression of gene expression in lymph nodes.
In vitro study confirmed the immunosuppressive effects of parathion and methoxychlor by detecting apoptosis in a human leukemia cell line (Jurkat E6.1) and a mouse lymphoma cell line (EL4). In this study, we observed a significant increase in DNA fragmentation and in the proportion of Annexin-V+ and caspase-3+ cells resulting from treatment with parathion and methoxychlor (see ). In addition, in both Jurkat E6.1 and EL4 cells, co-treatment with the caspase-3 inhibitors Z-VAD-FMK or Ac-DEVD-CHO inhibited the apoptosis induced by parathion and methoxychlor (see ). These findings suggest that each of these environmental chemicals induced T-lymphocyte apoptosis in vitro via the caspase-3 pathway.
Thus, in the in vivo study, we focused on the immune disorder of allergic responses to investigate further whether parathion and methoxychlor induce immune dysregulation. Using the LLNA, we studied the response of mice to the TH1-type allergen DNCB and the TH2-type allergen TMA. The LLNA assesses, in mice, the potential of topical application of a chemical to the skin to cause a primary T-lymphocyte proliferative response (Dearman et al., Citation1999). In the LLNA, contact allergens can be categorized on the basis of relative skin sensitization potency in the form of EC3 values derived from the SI (Kimber et al., Citation2003).
In the present study, marked decreases in EC3 values of TMA and DNCB were observed in parathion- and methoxychlor-pre-treated groups (see ). On the basis of the EC3 values, we categorize both TMA and DNCB as strong allergens after pre-treatment with vehicle and as extreme allergens after pre-treatment with high-dose parathion or methoxychlor. These findings suggest that the failure or breakdown of immune regulation caused by parathion and methoxychlor aggravates the acquired allergy to DNCB and TMA.
Then, to clarify further the mechanisms of increased allergic potential by prior exposure to parathion and methoxychlor, we performed detailed analysis including cell counts of helper and cytotoxic T-lymphocytes, T-lymphocyte–mediated cytokines, and gene expression. In the development of an immune response, activated local B-lymphocytes can act as antigen-presenting cells for helper- and cytotoxic T-lymphocytes (Goutet et al., Citation2005). The helper T-lymphocytes that recognize major histocompatibility complex (MHC) Class II molecules are of two functional types, TH1 and TH2 (Santana and Rosenstein, Citation2003). TH1-lymphocytes are specialized to activate macrophages that are promoted by, or have ingested, pathogens. TH2-lymphocytes are specialized for B-lymphocyte activation (Santana and Rosenstein, Citation2003).
To evaluate the state of activation of TH1- and TH2-lymphocytes following DNCB and TMA treatment, we assessed in lymph nodes the membrane expression of CD4 and CD8 molecules that are important for antigen presentation and regulation of the immune response (Murray, Citation1998). In our study, we observed statistical increases in the helper and cytotoxic T-lymphocyte populations in parathion- and methoxychlor-treated groups (see ). According to our results, since the MHC molecules that are recognized by helper- and cytotoxic T-lymphocytes, up-regulation of helper- and cytotoxic T-lymphocytes would produce several misleading types of allergens to antigen-presenting T-lymphocytes.
There is a body of evidence from human and animal studies to suggest the subdivision of the T-helper lymphocytes (TH1 and TH2) (Mosmann and Coffman, Citation1989). The TH1-lymphocytes produce IFNγ and TNFα, which direct the immune response toward the TH1-type response, including a cell-mediated immune response, whereas TH2-lymphocytes produce IL-4, IL-5, and IL-13, which are associated with IgE production (Kaplan et al., Citation1996; Shim et al., Citation2001). In our study, TMA induced a substantial increase in levels of TH2 cytokines (e.g. IL-4, IL-5, and IL-13) produced by ex vivo re-stimulated lymph node cells (see ). In contrast, the TH1 cytokine secretion profile was induced by identical treatment with the TH1-type allergen DNCB, with the production of high levels of IFNγ and TNFα (see ); these increases were associated with an increase in helper and cytotoxic T-lymphocyte populations in lymph nodes.
Recent clinical analysis suggests that in addition to TH1 factors, IL-17 is increased in contact hypersensitivity reactions (Joshi et al., Citation2009). In 2000, Infante-Duarte and colleagues first demonstrated that IL-17-producing T-lymphocytes were a TH population distinct from TH1- and TH2-lymphocytes in both mice and humans. Subsequently, the importance of IL-17-producing T-lymphocytes rather than TH1-lymphocytes in the development of contact hypersensitivity was demonstrated in IL-17-deficient mice (Nakae et al., Citation2002; Oboki et al., Citation2008). In this study, we observed that prior exposure to parathion or methoxychlor induced significant increases in IL-17 production (see and ). These data suggest that IL-17 is of clinical importance to the development and severity of several types of allergic potential by environmental chemicals.
Recently, a number of non-cytokine markers of TH1, TH2, and TH17 responses have been described, including the transcription factor T-bet, GATA-3 and ROR-γt, respectively. T-bet is known as a TH1 lineage commitment transcription factor as a result of its transactivation of the TH1 cytokine IFNγ (Szabo et al., Citation2000). GATA-3 has been described as a “master switch” in the development of TH2-lymphocytes; it activates expression of key TH2 genes, including the prototypic cytokines IL-4, IL-5, and IL-13 (Ritz et al., Citation2004). Ivanov et al. (Citation2006) indentified ROR-γt as a key transcription factor for TH17-lymphocyte differentiation. Thus, to evaluate the state of activation of TH1-, TH2-, and TH17-lymphocytes following treatment with several chemicals, we assessed the gene expression of T-bet, GATA-3, and ROR-γt, respectively, in the lymph node cells. In conclusion, we found that parathion and methoxychlor induced significant increases in the expression of T-bet, GATA-3, and ROR-γt (see ). These increases were associated with an increase in helper- and cytotoxic T-lymphocyte populations, and TH1 and TH2 cytokine production in the lymph nodes.
Overall, these results demonstrate that immunosuppressive environmental chemicals can disrupt immune function and increase the risk of developing markers for several types of allergy. Further confirmation of these results should expand to include various symptoms in parallel with changes in cellular function as well as immunologic or histologic markers.
The relationship between allergies and the breakdown of immune regulation induced by exposure to environmental chemicals is not fully understood. Our report suggests directions for further investigation into mechanisms of allergic diseases caused by environmental chemicals.
Acknowledgments
We thank Drs. Y. Shutoh, H. Fujie, A. Haishima, and Y. Komatsu of the Institute of Environmental Toxicology (Ibaraki, Japan) for their technical assistance.
Declaration of interest
The authors have no declarations of interest to disclose. The authors are alone responsible for the content and writing of the paper.
References
- Arts, J. H., Bloksma, N., Leusink-Muis, T., and Kuper, C. F. 2003. Respiratory allergy and pulmonary irritation to trimellitic anhydride in Brown Norway rats. Toxicol Appl Pharmacol. 187:38–49.
- Ban, M., Morel, G., Langonné, I., Huguet, N., Pépin, E., and Binet, S. 2006. TDI can induce respiratory allergy with Th2-dominated response in mice. Toxicology. 218:39–47.
- Basketter, D. A., Lea, L. J., Dickens, A., Briggs, D., Pate, I., Dearman, R. J., and Kimber, I. 1999. A comparison of statistical approaches to the derivation of EC3 values from local lymph node assay dose responses. J Appl Toxicol. 19:261–266.
- Beasley, R., Ellwood, P., and Asher, I. 2003. International patterns of the prevalence of pediatric asthma the ISAAC program. Pediatr Clin North Am. 50:539–553.
- Burney, P. G., Chinn, S., and Rona, R. J. 1990. Has the prevalence of asthma increased in children? Evidence from the national study of health and growth 1973-86. BMJ. 300:1306–1310.
- Casale, G. P.,Cohen, S. D. and DiCapua, R. A. 1983. The effects of organophosphate-induced cholinergic stimulation on the antibody response to sheep erythrocytes in inbred mice. Toxicol Appl Pharmacol. 68:198–205.
- Casale, G. P., Cohen, S. D. and DiCapua, R. A. 1984. Parathion-induced suppression of humoral immunity in inbred mice. Toxicol Lett. 23:239–247.
- Casillas, A. M., Hiura, T., Li, N. and Nel, A. E. 1999. Enhancement of allergic inflammation by diesel exhaust particles: Permissive role of reactive oxygen species. Ann Allergy Asthma Immunol. 83:624–629.
- Chapin, R. E., Harris, M. W., Davis, B. J., Ward, S. M., Wilson, R. E., Mauney, M. A., Lockhart, A. C., Smialowicz, R. J., Moser, V. C., Burka, L. T. and Collins, B. J. 1997. The effects of perinatal/juvenile methoxychlor exposure on adult rat nervous, immune, and reproductive system function. Fundam Appl Toxicol. 40:138–157.
- Dearman, R. J., Basketter, D. A. and Kimber, I. 1999. Local lymph node assay: Use in hazard and risk assessment. J Appl Toxicol. 19:299–306.
- Dearman, R. J., Humphreys, N., Skinner, R. A. and Kimber, I. 2005. Allergen-induced cytokine phenotypes in mice: Role of CD4 and CD8 T-cell populations. Clin Exp Allergy. 35:498–505.
- Fukuyama, T., Tajima, Y., Ueda, H., Hayashi, K., Shutoh, Y., Harada, T. and Kosaka, T. 2010a. A method for measuring mouse respiratory allergic reaction to low-dose chemical exposure to allergens: An environmental chemical of uncertain allergenicity, a typical contact allergen and a non-sensitizing irritant. Toxicol Lett. 195:35–43.
- Fukuyama, T., Tajima, Y., Ueda, H., Hayashi, K., Shutoh, Y., Harada, T. and Kosaka, T. 2010b. Apoptosis in immunocytes induced by several types of pesticides. J Immunotoxicol. 7:39–56.
- Fukuyama, T., Kosaka, T., Tajima, Y., Hayashi, K., Shutoh, Y., and Harada, T. 2010c. Detection of thymocytes apoptosis in mice induced by the organochlorine pesticide methoxychlor. Immunopharmacol Immunotoxicol. (In press).
- Fukuyama, T., Kosaka, T., Tajima, Y., Ueda, H., Hayashi, K., Shutoh, Y. and Harada, T. 2010d. Prior exposure to organophosphorus and organochlorine pesticides increases the allergic potential of environmental chemical allergens in a local lymph node assay. Toxicol Lett. 199:347–356.
- Fukuyama, T., Tajima, Y., Ueda, H., Hayashi, K., Shutoh, Y., Harada, T. and Kosaka, T. 2009. Allergic reaction induced by dermal and/or respiratory exposure to low-dose phenoxyacetic acid, organophosphorus, and carbamate pesticides. Toxicology. 261:152–161.
- Fukuyama, T., Ueda, H., Hayashi, K., Tajima, Y., Shuto, Y., Saito, T. R., Harada, T. and Kosaka, T. 2008a. Detection of low-level environmental chemical allergy by a long-term sensitization method. Toxicol Lett. 180:1–8.
- Fukuyama, T., Ueda, H., Hayashi, K., Tajima, Y., Shuto, Y., Saito, T. R., Harada, T. and Kosaka, T. 2008b. Use of long-term dermal sensitization followed by intratracheal challenge method to identify low-dose chemical-induced respiratory allergic responses in mice. Toxicol Lett. 181:163–170.
- Goutet, M., Pépin, E., Langonné, I., Huguet, N. and Ban, M. 2005. Identification of contact and respiratory sensitizers using flow cytometry. Toxicol Appl Pharmacol. 205:259–270.
- Grimaldi, C. M., Cleary, J., Dagtas, A. S., Moussai, D. and Diamond, B. 2002. Estrogen alters thresholds for B-cell apoptosis and activation. J Clin Invest. 109:1625–1633.
- Gurtu, V., Kain, S. R. and Zhang, G. 1997. Fluorometric and colorimetric detection of caspase activity associated with apoptosis. Anal Biochem. 251:98–102.
- Infante-Duarte, C., Horton, H. F., Byrne, M. C. and Kamradt, T. 2000. Microbial lipopeptides induce the production of IL-17 in Th cells. J Immunol. 165:6107–6115.
- Ivanov, I. I., McKenzie, B. S., Zhou, L., Tadokoro, C. E., Lepelley, A., Lafaille, J. J., Cua, D. J. and Littman, D. R. 2006. The orphan nuclear receptor RORγt directs the differentiation program of proinflammatory IL-17+ T-helper cells. Cell. 126:1121–1133.
- Joshi, A. D., Fong, D. J., Oak, S. R., Trujillo, G., Flaherty, K. R., Martinez, F. J. and Hogaboam, C. M. 2009. Interleukin-17-mediated immunopathogenesis in experimental hypersensitivity pneumonitis. Am J Respir Crit Care Med. 179:705–716.
- Kaplan, M. H., Schindler, U., Smiley, S. T. and Grusby, M. J. 1996. Stat6 is required for mediating responses to IL-4 and for development of Th2 cells. Immunity. 4:313–319.
- Kimber, I., Basketter, D. A., Butler, M., Gamer, A., Garrigue, J. L., Gerberick, G. F., Newsome, C., Steiling, W. and Vohr, H. W. 2003. Classification of contact allergens according to potency: Proposals. Food Chem Toxicol. 41:1799–1809.
- Kimber, I. and Weisenberger, C. 1989. A murine local lymph node assay for the identification of contact allergens. Assay development and results of an initial validation study. Arch Toxicol. 63:274–282.
- Larsen, C. P., Regal, R. R. and Regal, J. F. 2001. Trimellitic anhydride-induced allergic response in the guinea pig lung involves antibody-dependent and -independent complement system activation. J Pharmacol Exp Ther. 296:284–292.
- Matheson, J. M., Johnson, V. J. and Luster, M. I. 2005. Immune mediators in a murine model for occupational asthma: Studies with toluene diisocyanate. Toxicol Sci. 84:99–109.
- Matheson, J. M., Johnson, V. J., Vallyathan, V. and Luster, M. I. 2005. Exposure and immunological determinants in a murine model for toluene diisocyanate (TDI) asthma. Toxicol Sci. 84:88–98.
- Morris, R. G., Hargreaves, A. D., Duvall, E. and Wyllie, A. H. 1984. Hormone-induced cell death. 2. Surface changes in thymocytes undergoing apoptosis. Am J Pathol. 115:426–436.
- Mosmann, T. R. and Coffman, R. L. 1989. TH1 and TH2 cells: Different patterns of lymphokine secretion lead to different functional properties. Annu Rev Immunol. 7:145–173.
- Murray, J. S. 1998. How the MHC selects Th1/Th2 immunity. Immunol Today. 19:157–163.
- Nakae, S., Komiyama, Y., Nambu, A., Sudo, K., Iwase, M., Homma, I., Sekikawa, K., Asano, M. and Iwakura, Y. 2002. Antigen-specific T-cell sensitization is impaired in IL-17-deficient mice, causing suppression of allergic cellular and humoral responses. Immunity. 17:375–387.
- Narita, S., Goldblum, R. M., Watson, C. S., Brooks, E. G., Estes, D. M., Curran, E. M. and Midoro-Horiuti, T. 2007. Environmental estrogens induce mast cell degranulation and enhance IgE-mediated release of allergic mediators. Environ Health Perspect. 115:48–52.
- Oboki, K., Ohno, T., Saito, H. and Nakae, S. 2008. Th17 and allergy. Allergol Int 57:121–134.
- Peat, J. K. and Li, J. 1999. Reversing the trend: Reducing the prevalence of asthma. J Allergy Clin Immunol. 103:1–10.
- Plitnick, L. M., Loveless, S. E., Ladics, G. S., Holsapple, M. P., Smialowicz, R. J., Woolhiser, M. R., Anderson, P. K., Smith, C. and Selgrade, M. J. 2003. Identifying airway sensitizers: Cytokine mRNA profiles induced by various anhydrides. Toxicology. 193:191–201.
- Rao, T. and Richardson, B. 1999. Environmentally induced autoimmune diseases: Potential mechanisms. Environ Health Perspect. 107 Suppl 5:737–742.
- Ritz, S. A., Cundall, M. J., Gajewska, B. U., Swirski, F. K., Wiley, R. E., Alvarez, D., Coyle, A. J., Stampfli, M. R. and Jordana, M. 2004. The lung cytokine microenvironment influences molecular events in the lymph nodes during Th1 and Th2 respiratory mucosal sensitization to antigen in vivo. Clin Exp Immunol. 138:213–220.
- Santana, M. A. and Rosenstein, Y. 2003. What it takes to become an effector T-cell: The process, the cells involved, and the mechanisms. J Cell Physiol. 195:392–401.
- Shim, J. J., Dabbagh, K., Ueki, I. F., Dao-Pick, T., Burgel, P. R., Takeyama, K., Tam, D. C. and Nadel, J. A. 2001. IL-13 induces mucin production by stimulating epidermal growth factor receptors and by activating neutrophils. Am J Physiol Lung Cell Mol Physiol. 280:L134–L140.
- Sobel, E. S., Gianini, J., Butfiloski, E. J., Croker, B. P., Schiffenbauer, J. and Roberts, S. M. 2005. Acceleration of autoimmunity by organochlorine pesticides in (NZB x NZW)F1 mice. Environ Health Perspect. 113:323–328.
- Staples, J. E., Fiore, N. C., Frazier, D. E. Jr, Gasiewicz, T. A. and Silverstone, A. E. 1998. Over-expression of the anti-apoptotic oncogene, bcl-2, in the thymus does not prevent thymic atrophy induced by estradiol or 2,3,7,8-tetrachlorodibenzo-p-dioxin. Toxicol Appl Pharmacol. 151:200–210.
- Szabo, S. J., Kim, S. T., Costa, G. L., Zhang, X., Fathman, C. G. and Glimcher, L. H. 2000. A novel transcription factor, T-bet, directs Th1 lineage commitment. Cell. 100:655–669.
- Takeuchi, Y., Kosaka, T., Hayashi, K., Takeda, M., Yoshida, T., Fujisawa, H., Teramoto, S., Maita, K. and Harada, T. 2002. Thymic atrophy induced by methoxychlor in rat pups. Toxicol Lett. 135:199–207.
- Vanoirbeek, J. A., Mandervelt, C., Cunningham, A. R., Hoet, P. H., Xu, H., Vanhooren, H. M. and Nemery, B. 2003. Validity of methods to predict the respiratory sensitizing potential of chemicals: A study with a piperidinyl chlorotriazine derivative that caused an outbreak of occupational asthma. Toxicol Sci. 76:338–346.
- Vermes, I., Haanen, C., Steffens-Nakken, H. and Reutelingsperger, C. 1995. A novel assay for apoptosis. Flow cytometric detection of phosphatidylserine expression on early apoptotic cells using fluorescein labelled Annexin V. J Immunol Methods. 184:39–51.
- Wang, F., Roberts, S. M., Butfiloski, E. J., Morel, L. and Sobel, E. S. 2007. Acceleration of autoimmunity by organochlorine pesticides: A comparison of splenic B-cell effects of chlordecone and estradiol in (NZBxNZW)F1 mice. Toxicol Sci. 99:141–152.
- Ward, M. H., Colt, J. S., Metayer, C., Gunier, R. B., Lubin, J., Crouse, V., Nishioka, M. G., Reynolds, P. and Buffler, P. A. 2009. Residential exposure to polychlorinated biphenyls and organochlorine pesticides and risk of childhood leukemia. Environ Health Perspect. 117:1007–1013.
- Wiltrout, R. W., Ercegovich, C. D. and Ceglowski, W. S. 1978. Humoral immunity in mice following oral administration of selected pesticides. Bull Environ Contam Toxicol. 20:423–431.
- Xu, X., Dailey, A. B., Talbott, E. O., Ilacqua, V. A., Kearney, G. and Asal, N. R. 2010. Associations of serum concentrations of organochlorine pesticides with breast cancer and prostate cancer in U.S. adults. Environ Health Perspect. 118:60–66.
- Yanagisawa, R., Takano, H., Inoue, K., Koike, E., Sadakane, K. and Ichinose, T. 2008. Effects of maternal exposure to di-(2-ethylhexyl) phthalate during fetal and/or neonatal periods on atopic dermatitis in male offspring. Environ Health Perspect. 116:1136–1141.
- Zhang, N., Hartig, H., Dzhagalov, I., Draper, D. and He, Y. W. 2005. The role of apoptosis in the development and function of T-lymphocytes. Cell Res. 15:749–769.