Abstract
Asthma is a complex pulmonary inflammatory disease, which is characterized by airway hyper-responsiveness, airflow obstruction, and airway inflammation. Exposure to a number of chemicals including formaldehyde (FA) can lead to asthma. This study aimed to explore the underlying role of FA exposure in occupational asthma, especially when it is combined with allergen exposure. Balb/c mice were randomly divided into six groups (n = 6/group): (1) saline control; (2) ovalbumin (OVA)-immunized (OVAimm) only; (3) 0.5 mg FA/m3 exposure; (4) OVAimm + 0.5 mg FA/m3; (5) 3.0 mg FA/m3 FA exposure; and, (6) OVAimm + 3.0 mg FA/m3. These low and high exposure FA levels were adopted from current (0.5 mg/m3) and original (3.0 mg/m3) Chinese Occupational Threshold Limit Values. Experiments were conducted after 3 week of combined exposure and a 1-week challenge with aerosolized OVA. Airway hyper-responsiveness, pulmonary tissue damage, eosinophil infiltration, and increased interleukin (IL)-4 and IL-6 levels in lung tissues were found in the OVA + 3.0 mg FA/m3 hosts as compared to values seen in the OVA-immunized only mice. The results here suggest to us that FA exposure can induce and aggravate asthma in Balb/c mice when it is combined with OVA immunization.
Introduction
Formaldehyde (FA) is an occupational air pollutant and an ingredient used to prepare urea-FA, a resin that is widely used in office and home fixtures (Sherman et al., Citation2004; Gilbert et al., Citation2005). Indoor air FA pollution is widespread, especially in developing countries like China. In a recent review (Tang et al., Citation2009), the authors collected and analyzed over 200 Chinese and English documents from scientific journals; they concluded that the total mean occupational FA exposure level is 1.37 mg/m3 in factories (n = 1050), 1.46 mg/m3 in anatomy and pathology laboratories in medical colleges and hospitals (n = 215), and 0.256 mg/m3 in typical offices in China (n = 351). Thus, it appears that FA exposure is a serious environmental and occupational health problem and its health effects warrant further attention.
Recent epidemiological studies have been conducted for investigating the interrelationship between FA exposure and asthma (Franklin et al., Citation2000; Youk et al., Citation2001; Rumchev et al., Citation2002; Gautrin et al., Citation2003; Vandenplas et al., Citation2004; Casset et al., Citation2006; Mi et al., Citation2006; Syamlal et al., Citation2009). Whether FA causes asthma is still under debate; there are only a few reports on FA and respiratory sensitization (Salthammer et al., Citation2010). In a recent review focusing on the effects of FA, Arts et al. (Citation2008) evaluated the data available and concluded that it is still unknown whether there is a causal relationship between FA and allergic asthma or whether FA induces airway irritation resembling asthmatic reactions.
Another way to investigate the causal relationship between FA exposure and asthma is to undertake a toxicological study, but relative reports are limited when compared with epidemiological studies. Induction of airway hyper-responsiveness and asthma from FA inhalation exposure remains a debated and controversial issue. Previous studies have indicated that FA exposure can induce and aggravate asthma, i.e., after FA exposure the T-helper (TH)-2 cell system is activated, followed by an increase in IgE expression and other asthmatic pathological changes. Jung et al. (Citation2007) reported that FA exposure alone results in an increased count of eosinophils, higher levels of IgE and interleukin (IL)-4, and lung inflammation in mice. Similar results were also found in a rat model (Qiao et al., Citation2009). When combined with ovalbumin (OVA) sensitization, FA exposure can induce the following: increased serum levels of anti-OVA IgE in a mouse model (Tarkowski and Gorski, Citation1995); a high ratio (i.e., 10/12) in guinea pigs with positive signs by sensitive pulmonary function testing (Riedel et al., Citation1996); and, lung histological changes (i.e., pulmonary inflammation, airway remodeling), increased airway resistance, eosinophil infiltration, and increased IL-4 levels in bronchoalveolar lavage fluid (BALF) in rats (Qiao et al., Citation2009). Tavares-de-Limaa and colleagues (see Franco et al., Citation2006, Citation2009, Citation2010) obtained a different series of results. They found that FA exposure alone induces lung neutrophilic but not eosinophilic inflammation in rats; together with OVA immunization, FA exposure can evoke a low-intensity lung inflammation as indicated by the reduced enumerated number of inflammatory cells in BALF.
This study sought to clarify the discrepancy between results of previous studies, as well as to establish a representative experimental platform for further studies on molecular mechanisms underlying any FA-induced asthma.
Materials and methods
Animals
Balb/c mice (males, 7–8-week-old, 18–20 g) were purchased from the Hubei Experimental Animal Center (Wuhan, China) and maintained in a pathogen-free room at temperatures between 20–25°C and 50–70% humidity, with a 12-h light/dark cycle. All animals were provided access to standard rodent chow and distilled water ad libitum. All experimental procedures were approved by the Office of Scientific Research Management of Huazhong Normal University, with a Certification on Application for the Use of Animals (dated December 22, 2006). A total of six mice in each group was utilized so as to minimize the number of experimental animals needed while still ensuring (the validity of) statistical power.
Preparation of gaseous FA
To perform whole-body exposures, gaseous FA at pre-set concentrations of 0.5 and 3.0 mg/m3 was generated with 10% formalin (Sigma, St. Louis, MO) that was diluted with filtered house air prior to delivery into a small exposure chamber (Model WH-2, Yuxin S&T Development Co. Ltd., Wuhan, China). The test animals were placed in a glass vessel (exposure chamber) connected to the FA generator and exposed to the gaseous FA. During the experiment, exposure parameters were controlled automatically based on chamber readings. Air temperature, humidity, and ventilation rate were maintained at 23 ± 0.5°C, 45 ± 5.0%, and 1.0 L/min, respectively. The low and high FA levels used were based on Chinese Occupational Threshold Limit Values (Administration of Technology Supervision of People’s Republic of China, Citation2002) of 0.5 mg FA/m3 (current standard) and 3.0 mg FA/m3 (abandoned standard).
Challenge protocol
Balb/c mice were randomly allocated into six groups (n = 6/group): (1) saline control; (2) ovalbumin (OVA)-immunized (OVAimm) only; (3) 0.5 mg FA/m3 exposure; (4) OVAimm + 0.5 mg FA/m3; (5) 3.0 mg FA/m3 FA exposure; and, (6) OVAimm + 3.0 mg FA/m3. The details of FA challenge and normal asthma model preparation protocols are shown in .
Figure 1. OVA-combined FA exposure protocol. (1) saline control group; (2) OVA-immunized only group (OVA only); (3) 0.5 mg FA/m3 exposure group (FA0.5); (4) OVA-combined 0.5 mg FA/m3 exposure group (FA0.5+OVA); (5) 3.0 mg FA/m3 exposure group (FA3.0); and (6) OVA-combined 3.0 mg FA/m3 exposure group (FA3.0+OVA).
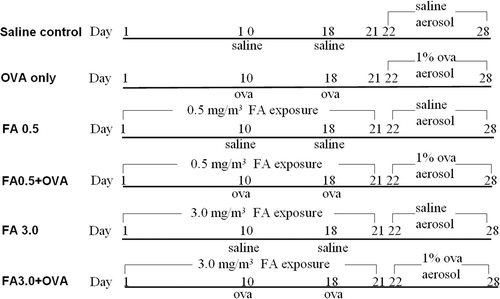
In the OVA-immunized only (OVAimm) group, each mouse was injected intravenously on Days 10 and 18 with 200 µl of a normal saline solution containing 20 mg OVA (Sigma) and 20 mg aluminum hydroxide each time, followed by an aerosol challenge with 1% OVA for 30 min/d on Days 22–28 using an ultrasonic nebulizer (Model Yuyue 402A-I, Yuyue Medical Apparatus and Instruments Co., Danyang, China). The saline control group was treated in the same manner, but exposed to saline instead of OVA on Days 10, 18, and 22–28. To determine the effect of allergen-combined FA exposure, the OVAimm + 0.5 mg FA/m3 (FA0.5+OVA) and OVAimm + 3.0 mg FA/m3 (FA3.0+OVA) groups were treated the same as the OVAimm only group, except that the FA exposure (6 h/d) period varied from Day 1 to 21. In the FA0.5 and FA3.0 only groups, saline was substituted for OVA.
To prevent adversely affecting measures of lung cytokines/pulmonary architecture due to any possible influence from the methacholine used in airway hyper-responsiveness (AHR; see below) assessments, dedicated groups of mice (for each of the above regimens) were employed. One set of 36 mice was treated for 28 days according to the above-regimen(s) and then used for AHR tests and BALF sample collection; a second set of 36 mice was treated as above and then used directly (i.e., non-lavaged) for cytokine (left lung) and histopathologic (right lung) analyses.
Airway hyper-responsiveness measurements
Airway hyper-responsiveness as an indicator of lung function was tested 24 h after the final aerosol exposure using an AniRes 2005 lung function system (Bestlab, AniRes 2005, Version 2.0, Beijing, China). Animals were anesthetized intraperitoneally with 90 mg/kg sodium pentobarbital (Urchen, Shanghai, China) and then connected to a computer-controlled ventilator via a cannula that had been surgically implanted in the trachea using protocols we have described previously (Qiao et al., Citation2009). The respiratory rate was pre-set at 95 times/min and the expiration/inspiration time ratio was set at 1.5:1. When the baseline parameters were stable, a 27-gauge needle was inserted into the jugular vein and methacholine (O-acetyl-β-methylcholine chloride, MCh; Sigma) was administered through a catheter; increasing dosages of 0, 0.025, 0.05, 0.1, and 0.2 mg MCh/kg were injected every 5 min. Mouse airway responsiveness in each dosing cycle was examined by measuring expiratory resistance (Re), inspiratory resistance (Ri), and dynamic lung compliance (CLdyn). The relative area (R-area) was defined as the area under the peak curve of Re or Ri and beyond the baseline level during the 250-s timeframe (Note: the missing 30 s to fill out the ‘5 min’ dosing cycle was used to freshly prepare the next MCh dose before its injection). The distance from the wave trough to the baseline level of the CLdyn curve was adopted to quantitatively assess lung compliance (Drazen et al., Citation1999).
Bronchoalveolar lavage fluid (BALF) collection and cell counts
BALF was collected immediately after the conclusion of the airway hyper-responsiveness measurements. Specifically, after the final 0.2 mg MCh/kg challenges, the mice were removed from the operation box and euthanized by cervical dislocation. Intratracheal cannulation was then performed using a 1 ml syringe; once the cannula was place, 0.5 ml saline was injected into the trachea and lungs. The chest of each mouse was gently massaged and rinsed for ≈1 min, and then the liquid withdrawn with the same syringe. The process was repeated three times; the total quantity of BALF was 1.2–1.3 ml for each sample.
Cell samples in the BALF were collected by centrifugation (1000 rpm, 10 min, 4°C) and re-suspended in 1 ml saline. Total cell counts were obtained from 500 µl of the cell suspension using a blood cell analysis system (Sysmex KX-21, Kobe, Japan). Another 500 µl was prepared for eosinophil counts. Eosinophils were stained using Eosin Y sodium solution (Amresco, high purity grade, Solon, OH), and then assessed using a hemacytometer and a DM 4000B microscope (Leica, Wetzlar, Germany). Neutrophils or monocytes were not counted due to the limited BALF.
Pulmonary histopathological analyses
Right lungs from non-lavaged mice were isolated for histopathology slice preparation. All samples were incubated in fixative (saturated 2,4,6-trinitrophenol:formalin:glacial acetic acid [15:5:1 v/v/v]) for 24 h at room temperature, then cut into pieces, and stained with hematoxylin and eosin (H&E, Sigma). The stained pieces were then embedded in paraffin, sectioned into 10-µm slices and observed using the DM 4000B microscope. Results were then reported in terms of slight, moderate, or severe changes. Specifically, the designation: ‘Slight’ refers to a slight thickening of the airway wall without damage/folding on the cuticular layer of the airway, or cell infiltration around airway and vessels; ‘Moderate’ refers to a bit of thickening of the airway wall and there some folding on the cuticular layer, and a little cell infiltration around airway and vessels; and, ‘Severe’ refers to obvious thickening of the airway wall and severe damage/folding on the cuticular layer, with mucus inside the airway and great amounts of cell infiltration around airways and vessels.
Detection of the IL-4, IL-6, and interferon (IFN)-γ
Levels of IL-4, IL-6, and IFNγ in non-lavaged left lung tissue homogenates were assessed using commercial ELISA kits according to manufacturer’s instructions (eBioscience, San Diego, CA). The sensitivities of each ELISA kit was 15 pg/ml. Homogenates were prepared by placing the tissue sample (in 0.9 ml of normal saline) in a glass made homogenizer and then processing the tissues using 15 strokes of a loose-fitting glass pestle. Cellular debris was then pelleted by centrifugation at 2300 × g for 5 min at 4°C; the resulting supernatant was transferred to Eppendorf tubes for storage at−80°C until utilized for these cytokine analyses.
Statistical analysis
All data were analyzed and plotted using Origin 6.0 software. Before performing any analyses, all data were assessed for normal distribution. Thereafter, both a one-way analysis of variance (ANOVA) and a Student’s t-test were applied to evaluate significant differences. A p value < 0.05 was used to evaluate statistical significance.
Results
Assessment of airway hyper-responsiveness
The results show that Re and Ri rose in a dose-dependent manner, while CLdyn decreased because of an increase in the distance from the wave through to the baseline level (). All of the OVA-treated mice, including those in the OVA only, FA 0.5 + OVA, and FA 3.0 + OVA groups, exhibited greater airway responses to MCh when compared with those of the saline controls. The FA 0.5 + OVA and FA 3.0 + OVA groups had a significantly enhanced airway response compared with the OVA-immunized-only group and the saline control group. Interestingly, the FA 3.0 + OVA mice showed a very marked change in their airway responses compared with mice in the OVA-immunized-only group, resulting in an extreme decline in lung function. On the other hand, the two groups exposed to FA only (FA 0.5 and FA 3.0) did not display any significant changes (relative to controls), even though their responsiveness was higher than that of the saline mice but lower than that of the OVA-immunized-only mice.
Figure 2. Airway hyper-responsiveness assessment in mice. With different doses of MCh: (A) R-area of Re; (B) R-area of Ri; and, (C) distance from peak value of CLdyn to baseline level, where ‘difference’ refers to the difference between baseline and peak value in CL measurements. Value significantly different compared with saline control (*p < 0.05, **p < 0.01) or OVA-immunized-only group (##p < 0.01).
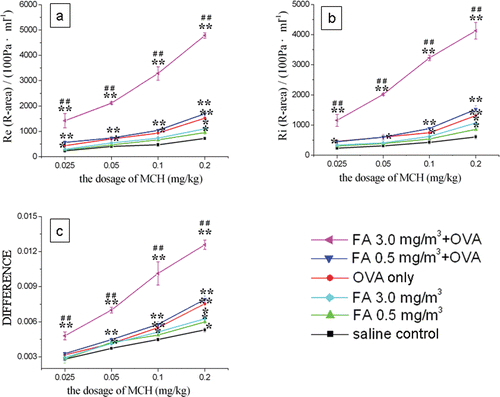
Pulmonary histopathology
Histopathological changes associated with changes in lung function (re: airway hyper-responsiveness) were assessed. There were clear pulmonary histopathological changes, including inflammatory cell infiltration, airway wall thickening, and remodeling (each of which are associated with asthma in humans) in all OVA-treated groups (i.e., OVA-immunized-only, FA 0.5 + OVA and FA 3.0 + OVA; ). The most severe changes, including cell infiltration and airway remodeling, were found in the FA 3.0 + OVA mice. In addition, there were slight changes observed in the FA3.0 group compared with the saline control group.
Figure 3. Histopathology for lung inflammation (tissue cell infiltration) and airway remodeling. Lung tissue was fixed, stained with H&E, and sectioned in 10-µm slices. →: Bronchial remodeling – –▸: lung tissue cell infiltration; N: normal conditions; S: slight changes;M: moderate changes; V: severe changes. Magnification = 20X.
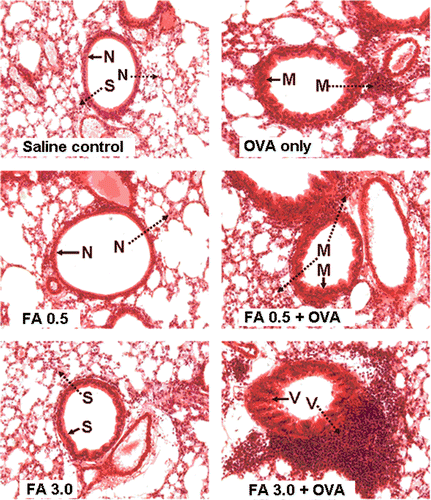
Eosinophil infiltration in BALF
The ratios of eosinophils to total cells contained in BALF of the FA exposure groups (i.e., FA 0.5, FA 0.5 + OVA, FA 3.0, and FA 3.0 + OVA) were all significantly increased compared with ratios among the saline controls (, p < 0.01). Furthermore, OVA immunization significantly intensified the increased eosinophil infiltration induced by FA (p < 0.01).
Cytokine detection
Both FA exposure and OVA-combined FA exposure enhanced IL-4 and IL-6 levels in pulmonary tissue homogenates ( and ). FA 3.0 exposure, but not FA 0.5 exposure, induced a significant increase in IL-4 and IL-6 levels, even compared with the OVA-immunized only group. Among all the groups, FA 3.0 + OVA exposure induced the highest IL-4 and IL-6 levels. However, IFNγ levels were not significantly different among the groups ().
Figure 5. Pulmonary tissue IL-4 levels in the different experimental groups. Value significantly different compared with saline control (*p < 0.05, **p < 0.01) or OVA-immunized-only group (##p < 0.01).
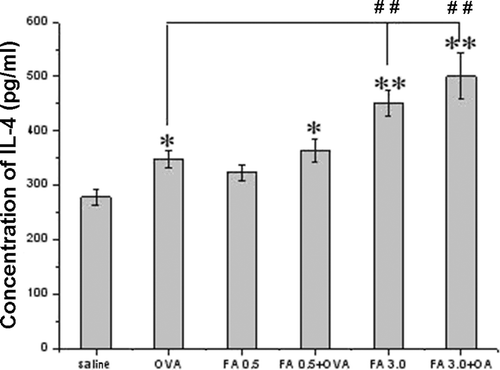
Discussion
Considerations of the experimental model
Mice are usually used as animal models for imitating allergic asthma, as they are small and inexpensive animals (Karol, Citation1994; Drazen et al., Citation1999; Allen et al., Citation2009). A recent study indicated that the particular mouse strain that is used has considerable and variable impacts on different aspects of the asthma phenotype, and human phenotypic characteristics of chemically-induced occupational asthma are best reproduced in TH2-biased mice, and in particular, in Balb/c mice (De Vooght et al., Citation2010).
The main reasons we used the mouse as an experimental subject are as follows. First, its allergic reactions tend to be mediated by the TH2 lymphocyte system and pulmonary eosinophilic inflammation is induced, and these phenomena are also important variables for human asthma diagnosis. Second, the results with mice can be compared with other studies, as the mouse is the most commonly used model in asthma studies and an ideal species for investigation at the interface of lung biology and lung function of allergic asthma. Third, six groups of mice were utilized in this study, whereas there were only five groups used in the earlier-cited rat model Qiao et al. paper (Citation2009); that study lacked a group for the single effect of FA alone at one of the doses it had been used in combination with OVA. In other words, the experimental design in the current study is more complete than that in the Qiao et al. paper.
Nevertheless, both this and the Qiao et al. (Citation2009) papers are markedly improved in terms of indices determination and model development than those proposed by other groups, i.e., the models mentioned in many other papers might be defined as ‘animal models’ used for related toxicity investigations, but not specifically (as an ‘asthma model’) for the study of the pathogenesis of asthma. Specifically, those papers only provided indirect evidence from some other physiologic aspect, e.g., the aim of studies by Jung et al. (Citation2007) and Fujimaki et al. (Citation2004) was to examined the effect of FA on inflammation, while that of Franco et al. (Citation2006, Citation2009, Citation2010) was to ascertain the influence of FA on general lung function. In contrast, the common goal of both this and the Qiao et al. paper is asthma, and the indices measured involved multiple aspects and related complicated immunological process involved in the pathogeneses of asthma.
Parameters for evaluating allergic asthma in the mouse model
Based on the parameters suggested by Karol (Citation1994), we established a system for evaluating allergic asthma of animal models. The results of our experiments agree with this system, and we have successfully established a FA-induced asthma model in Balb/c mice in our laboratory.
Exposure conditions
Previous studies have found that after FA exposure, anti-FA IgE was neither present in the mouse body (Tarkowski and Gorski, Citation1995) nor in the human body (Kramps et al., Citation1989; Liden et al., 1993, Krakowiak et al., Citation1998), but the level of anti-OVA IgE in mice exposed to FA was increased after FA exposure (Tarkowski and Gorski, Citation1995). This finding suggests that FA is not an antigen, but an adjuvant (Wolkoff et al., Citation2010); therefore, FA may only induce asthma when it occurs with allergen (i.e., OVA) combined exposure. On the other hand, FA is an irritant that may induce non-immunological asthma; the latter has been defined as an “asthma” (more or less akin to reactive airways syndrome) that usually arises after several hours of single exposure to a relatively high concentration of stimulating factor by Vandenplas and Malo (Citation2003); under these conditions, the effects of FA without OVA needed to be evaluated.
In this study, the exposure levels were determined by referring to the current standard and the abandoned standard of the Chinese Occupational Threshold Limit Values. Similar levels of indoor FA are often found in occupational places, even in residential housing in China. Therefore, in the current study, we used concentrations that can be found in the home or work place. Some of the previous studies (Franco et al., Citation2006, Citation2009, Citation2010) did not indicate the real FA exposure concentration in units of ppm or mg/m3 and the actual exposure levels may have been far higher than that in real life. Consequently, the biological effects such as the IgE or IgG1 expression and eosinophil count in BALF were different among the studies (Tarkowski and Gorski, Citation1995; Riedel et al., Citation1996; Jung et al., Citation2007; Qiao et al., Citation2009; see ).
The exposure time and frequency are also very important aspects (see ). FA exposure for 6 or 8 h every day lasting 2 or 3 week has been found to successfully establish FA-induced asthma (Riedel et al., Citation1996; Jung et al., Citation2007; Qiao et al., Citation2009). Exposure times that are too long (Fujimaki et al., Citation2004) or too short (Franco et al., Citation2006, Citation2009, Citation2010) may result in different conclusions.
Table 1. Comparison of FA exposure conditions and previous study results among the known relative toxicological studies.
Conclusions
(1) The results of our toxicological study suggest that using the Balb/c mouse as an animal model of FA exposure alone (FA 3.0) can only induce mild pulmonary inflammation (i.e. increased levels of TH2 and pro-inflammatory cytokines and an increased number of eosinophils) in the airways, but no asthma occurs. If FA exposure is combined with OVA sensitization, serious damage can be caused in lung function and airway remodeling, and then asthma can occur. (2) The above conclusions cannot be directly applied to humans, because species differences in immune responses cannot be ignored, even if the Balb/c mouse is the best animal model for chemically-induced occupational asthma. (3) The protocol used in our experiments may provide an experimental platform for further studies for understanding molecular mechanisms of FA-induced asthma.
Acknowledgements
We gratefully acknowledge Edanz Editing China for revising the language.
Declaration of interest
This work was financially supported by National Science Foundation for Distinguished Young Scholars (50725620). The funders had no role in study design, data collection and analysis, decision to publish, or preparation of the manuscript.
References
- Administration of Technology Supervision of People’s Republic of China. 2002. GBZ 2-2002: Occupational Exposure Limit for Hazardous Agents in the Workplace. Beijing.
- Allen, J. E., Bischof, R. J., Sucie Chang, H. Y., Hirota, J. A., Hirst, S. J., Inman, M. D., Mitzner, W. and Sutherland, T. E. 2009. Animal models of airway inflammation and airway smooth muscle remodelling in asthma. Pulm Pharmacol Ther. 22:455–465.
- Arts, J. H., Muijser, H., Kuper, C. F. and Woutersen, R. A. 2008. Setting an indoor air exposure limit for formaldehyde: Factors of concern. Regul Toxicol Pharmacol. 52:189–194.
- Casset, A., Marchand, C., Purohit, A., le Calve, S., Uring-Lambert, B., Donnay, C., Meyer, P. and de Blay, F. 2006. Inhaled formaldehyde exposure: Effect on bronchial response to mite allergen in sensitized asthma patients. Allergy. 61:1344–1350.
- De Vooght, V., Vanoirbeek, J. A., Luyts, K., Haenen, S., Nemery, B. and Hoet, P. H. 2010. Choice of mouse strain influences the outcome in a mouse model of chemical-induced asthma. PLoS ONE. 5:e12581.
- Drazen, J. M., Finn, P. W. and De Sanctis, G. T. 1999. Mouse models of airway responsiveness: physiological basis of observed outcomes and analysis of selected examples using these outcome indicators. Annu Rev Physiol. 61:593–625.
- Franklin, P., Dingle, P. and Stick, S. 2000. Raised exhaled nitric oxide in healthy children is associated with domestic formaldehyde levels. Am J Respir Crit Care Med. 161:1757–1759.
- Lino dos Santos Franco, A., Damazo, A. S., Beraldo de Souza, H. R., Domingos, H. V., Oliveira-Filho, R. M., Oliani, S. M., Costa, S. K. and Tavares de Lima, W. 2006. Pulmonary neutrophil recruitment and bronchial reactivity in formaldehyde-exposed rats are modulated by mast cells and differentially by neuropeptides and nitric oxide. Toxicol Appl Pharmacol. 214:35–42.
- Lino dos Santos Franco, A., Domingos, H. V., Damazo, A. S., Breithaupt-Faloppa, A. C., de Oliveira, A. P., Costa, S. K., Oliani, S. M., Oliveira-Filho, R. M., Vargaftig, B. B. and Tavares-de-Lima, W. 2009. Reduced allergic lung inflammation in rats following formaldehyde exposure: Long-term effects on multiple effector systems. Toxicology. 256:157–163.
- Lino-dos-Santos-Franco, A., Domingos, H. V., de Oliveira, A. P., Breithaupt-Faloppa, A. C., Peron, J. P., Bolonheis, S., Muscará, M. N., Oliveira-Filho, R. M., Vargaftig, B. B. and Tavares-de-Lima, W. 2010. Differential effects of formaldehyde exposure on the cell influx and vascular permeability in a rat model of allergic lung inflammation. Toxicol Lett. 197:211–218.
- Fujimaki, H., Kurokawa, Y., Kunugita, N., Kikuchi, M., Sato, F. and Arashidani, K. 2004. Differential immunogenic and neurogenic inflammatory responses in an allergic mouse model exposed to low levels of formaldehyde. Toxicology. 197:1–13.
- Gautrin, D., Newman-Taylor, A. J., Nordman, H. and Malo, J. L. 2003. Controversies in epidemiology of occupational asthma. Eur Respir J. 22:551–559.
- Gilbert, N. L., Guay, M., David Miller, J., Judek, S., Chan, C. C. and Dales, R. E. 2005. Levels and determinants of formaldehyde, acetaldehyde, and acrolein in residential indoor air in Prince Edward Island, Canada. Environ Res. 99:11–17.
- Jung, W. W., Kim, E. M., Lee, E. H., Yun, H. J., Ju, H. R., Jeong, M. J., Hwang, K. W., Sul, D., and Kang, H. S. 2007. Formaldehyde exposure induces airway inflammation by increasing eosinophil infiltrations through the regulation of reactive oxygen species production. Environ Toxicol Pharmacol. 24:174–182.
- Karol, M. H. 1994. Animal models of occupational asthma. Eur Respir J. 7:555–568.
- Krakowiak, A., Górski, P., Pazdrak, K. and Ruta, U. 1998. Airway response to formaldehyde inhalation in asthmatic subjects with suspected respiratory formaldehyde sensitization. Am J Ind Med. 33:274–281.
- Kramps, J. A., Peltenburg, L. T., Kerklaan, P. R., Spieksma, F. T., Valentijn, R. M. and Dijkman, J. H. 1989. Measurement of specific IgE antibodies in individuals exposed to formaldehyde. Clin Exp Allergy. 19:509–514.
- Lidén, S., Scheynius, A., Fischer, T., Johansson, S. G., Ruhnek-Forsbeck, M. and Stejskal, V. 1993. Absence of specific IgE antibodies in allergic contact sensitivity to formaldehyde. Allergy. 48:525–529.
- Mi, Y. H., Norbäck, D., Tao, J., Mi, Y. L. and Ferm, M. 2006. Current asthma and respiratory symptoms among pupils in Shanghai, China: Influence of building ventilation, nitrogen dioxide, ozone, and formaldehyde in classrooms. Indoor Air. 16:454–464.
- Qiao, Y., Yan, Y., Yang, J. W., Lu, Z. S., and Yang, X. 2007. Study on the mechanism of asthma caused by the acquired atopy induced by formaldehyde. Chinese J Microbiol Immunol. 27:298–299.
- Qiao, Y., Li, B., Yang, G., Yao, H., Yang, J., Liu, D., Yan, Y., Sigsgaard, T. and Yang, X. 2009. Irritant and adjuvant effects of gaseous formaldehyde on the ovalbumin-induced hyper-responsiveness and inflammation in a rat model. Inhal Toxicol. 21:1200–1207.
- Riedel, F., Hasenauer, E., Barth, P. J., Koziorowski, A. and Rieger, C. H. 1996. Formaldehyde exposure enhances inhalative allergic sensitization in the guinea pig. Allergy. 51:94–99.
- Rumchev, K. B., Spickett, J. T., Bulsara, M. K., Phillips, M. R. and Stick, S. M. 2002. Domestic exposure to formaldehyde significantly increases the risk of asthma in young children. Eur Respir J. 20:403–408.
- Salthammer, T., Mentese, S. and Marutzky, R. 2010. Formaldehyde in the indoor environment. Chem Rev. 110:2536–2572.
- Sherman, M. H. and Hodgson, A. T. 2004. Formaldehyde as a basis for residential ventilation rates. Indoor Air. 14:2–8.
- Syamlal, G., Mazurek, J. M. and Bang, K. M. 2009. Prevalence of lifetime asthma and current asthma attacks in U.S. working adults: An analysis of the 1997-2004 National Health Interview Survey data. J Occup Environ Med. 51:1066–1074.
- Tang, X., Bai, Y., Duong, A., Smith, M. T., Li, L. and Zhang, L. 2009. Formaldehyde in China: Production, consumption, exposure levels, and health effects. Environ Int. 35:1210–1224.
- Tarkowski, M. and Gorski, P. 1995. Increased IgE antiovalbumin level in mice exposed to formaldehyde. Int Arch Allergy Immunol. 106:422–424.
- Vandenplas, O. and Malo, J. L. 2003. Definitions and types of work-related asthma: A nosological approach. Eur Respir J. 21:706–712.
- Vandenplas, O., Fievez, P., Delwiche, J. P., Boulanger, J. and Thimpont, J. 2004. Persistent asthma following accidental exposure to formaldehyde. Allergy. 59:115–116.
- Wolkoff, P. and Nielsen, G. D. 2010. Non-cancer effects of formaldehyde and relevance for setting an indoor air guideline. Environ Int. 36:788–799.
- Youk, A. O., Marsh, G. M., Stone, R. A., Buchanich, J. M. and Smith, T. J. 2001. Historical cohort study of US man-made vitreous fiber production workers: III. Analysis of exposure-weighted measures of respirable fibers and formaldehyde in the nested case-control study of respiratory system cancer. J Occup Environ Med. 43:767–778.