Abstract
Cell-mediated immunity offers protection against virus-infected cells and tumor cells, involves activation of natural killer (NK) cells, production of antigen-specific cytotoxic T-lymphocytes, and release of various cytokines in response to an antigen. Administration of an ethanolic extract of Aerva lanata was found to stimulate cell-mediated immunological responses in normal and tumor-bearing BALB/c mice. A significant enhancement in NK cell activity in both normal and tumor-bearing hosts was observed after administration of A. lanata. Antibody-dependent cellular cytotoxicity (ADCC) and antibody-dependent complement-mediated cytotoxicity (ACC) were significantly enhanced as well in both sets of treated hosts. In addition, in vivo production of IL-2 and IFNg were each significantly enhanced by extract treatment. The stimulatory effect of A. lanata on cytotoxic T-lymphocyte (CTL) production was determined by Winn’s neutralization assay using CTL-sensitive EL4 thymoma cells. A. lanata treatment caused a significant increase in CTL production in both in vivo and in vitro models, in each case as indicated by a significant increase in the life-spans of tumor-injected mice. Taken together, all of these results in the murine model indicate that administration of an ethanolic extract of A. lanata could enhance the cell-mediated anti-tumor response.
Introduction
Despite vast improvements in detection, diagnosis, and treatment over the past decade, cancer still remains the major public health problem in India as well as in the Western world. Currently, one in four deaths in the United States is due to cancer (Jemal et al., Citation2004). Worldwide, cancer is the second leading cause of death (after cardiovascular disease) and in the United Kingdom it is the leading cause of death. Even today, classical anti-proliferative cytotoxic agents form the basis of many current treatment regimens (Atkinson et al., Citation2008). Despite being potent with the ability to kill a large number of tumor cells, the clinical efficacy of these agents is limited by their unavoidable toxicity to “normal” cells and the suppression of the immune system. It has been suggested that the “escape” of growing tumors from host immune surveillance could be associated with this immunosuppression in a tumor-bearing host (Kobayashi et al., Citation1990).
An immunomodulator is a substance that has an effect on the immune system; it induces either immunostimulation or immunosuppression (Thejass and Kuttan, Citation2007). Immunostimulation via natural substances is considered to be a most promising way for the prevention and cure of neoplastic diseases. Immunomodulatory agents mediate their actions by activating cytotoxic effector cells such as cytotoxic T-lymphocytes (CTL), natural killer (NK), lymphocytes, macrophages, and neutrophils.
Natural plant products have played a pivotal role in the health care of many cultures, both ancient and modern. Several medicinal herbs have been shown to promote immunity by augmenting specific cellular and humoral immune response. Several medicinal plants used in the Indian system of holistic medicine (known as Ayurveda) are considered to possess immuno-modulatory properties. Aerva lanata, a member of the Amaranthaceae family, is an important medicinal plant growing throughout the plains of India (Chopra, Citation1956). It is widely used in Indian folk medicine for the treatment of several disorders such as diabetes mellitus, urinary calculi, hematemesis, bronchitis, nasal bleeding, cough, etc. Among its myriad of constituents, A. lanata is reported to contain Aerva flavonoid, glycosides, aervitrin, aervolanine, aervoside, amyrin, betulin, campesterol, canthin-6-one, 10-hydroxy-canthin-6-one, 10-methoxy-canthin-6-one, β-carboline-1-propionic acid, chrysin, β-ecdysone, daucosterol, hentriacontane, narcissin, β-sitosterol, syringic acid, feruloyl tyramine, and vanillic acid (Zapesochnaya et al., Citation1991; Citation1992; Vetrichelvan and Jegadeesan, Citation2002). To date, the specific agent(s) in A. lanata preparations that is/are responsible for each of the individual medicinal effects remain to be conclusively defined.
Our previous studies with an ethanolic extract of A. lanata showed that it could enhance the total white blood cell (WBC) count, number of splenic plaque-forming cells, circulating antibody titres, ex vivo proliferation of splenocytes, thymocytes, and bone marrow cells, and inhibit Dalton’s lymphoma ascites (DLA) induced solid tumor development in mice (Siveen and Kuttan, 2011a). Also, it has been observed that a partially-purified fraction (PEF) of a petroleum ether extract of this plant was cytotoxic to DLA, Ehrlich ascites, and B16F10 cell lines in vitro (Nevin and Vijayammal, Citation2003). Therefore, the major objectives of the present study were to investigate the effect of A. lanata extract (ethanolic) on cell-mediated immune responses under normal as well as tumor-bearing conditions in a host. These studies also sought to evaluate the effect of this extract on the generation and activities of cytotoxic T-lymphocytes, key effector cells in mediating host resistance to tumors.
Materials and methods
Cell lines
K-562 and EL4 (thymoma) cells were obtained from National Centre for Cell Sciences, Pune, India. Ehrlich ascites carcinoma (EAC) cells were originally obtained from Cancer Institute, Mumbai, India. Sheep red blood cells (SRBC) were freshly collected from sheep maintained in the animal house of Amala Cancer Research Centre (Kerala, India) and placed/maintained in Alsever’s solution.
Animals
BALB/c (male, 25–28 g, 6–8-wk-old) mice were from the Amala Cancer Research Centre breeding section; C57BL/6 mice (male, 25–28 g, 6–8-wk-old) were from the National Institute of Nutrition, Hyderabad, India. All mice were maintained in a pathogen-free room at temperatures between 20–25°C and 50-70% humidity, with a 12-h light/dark cycle, and provided with mouse chow (Sai Durga Feeds and Food, Bangalore, India) and filtered water ad libitum. All experiments were carried out with the prior approval of the Institutional Animal Ethics Committee (IAEC) and were conducted strictly adhering to the guidelines of the Committee for the purpose of Control and Supervision of Experiments on Animals (CPCSEA) constituted by the Animal Welfare Division of the Government of India.
Reagents
Tissue culture media RPMI 1640 and DMEM were purchased from Hi-Media Laboratories (Mumbai, India). Tritiated (3H)-thymidine and chromium-51 (51Cr) were purchased from BRIT (Mumbai, India). Specific quantitative sandwich ELISA kits for mouse interleukin (IL)-2 and interferon (IFN)-g were purchased from Pierce Biotechnology (Rockford, IL). Mitomycin C was obtained from SISCO Research Laboratory (Mumbai, India). All other reagents were of analytical reagent quality.
Preparation of plant extract
Authenticated Aerva lanata obtained from Amala Ayurveda Pharmacy was shade-dried and powdered; whole plant material was used to prepare the extract. Specifically, ≈ 100 g of powder was subjected to 85% ethanolic extraction in a Soxhlet apparatus for 24 h. The solvent was then evaporated to dryness at 42°C under reduced pressure using a rotary evaporator; extract yield was 16.7% (w/w). The extract was re-dissolved in a minimum amount of dimethyl sulfoxide (DMSO, 0.1%) for subsequent use in in vitro studies. For animal experiments, the material was re-suspended in 1% gum acacia to yield samples that could then deliver a dose of 10 mg extract/kg body weight. The choice to use this dosage was based on earlier toxicity studies in which mice received this extract by intraperitoneal (IP) injection, and survival was monitored (see Siveen and Kuttan, 2011a).
Toxicological evaluation of ethanolic extract of A. lanata
BALB/c mice were divided into six groups (n = 48, 8 per group). Group I was kept without any treatment as untreated control. The vehicle control group (Group II) received 1% gum acacia, while the experimental groups (Groups III–VI) received A. lanata extract (5, 10, 50, or 100 mg/kg body weight [BW]) intraperitoneally daily for 14 days. All mice were observed for mortality, behavioral changes, and changes in body weight. All mice were euthanized by cervical dislocation on the day after the final treatment (i.e., Day 15). Selected organs such as liver, spleen, thymus, kidney, and lungs were asceptically removed and their weights recorded. Blood was collected by heart puncture; the serum was isolated and used for the analysis of hepatic and renal function. Liver function markers, such as alkaline phosphatase (ALP) (King, Citation1965) and glutamate pyruvate transaminase (GPT) (Bergmeyer and Bernt, Citation1980), and markers of kidney function such as creatinine (Toro and Ackermann, Citation1975) and blood urea nitrogen (Murray, Citation1984) were determined.
Determination of the effect of A. lanata on cell-mediated immune response
BALB/c mice were divided into three groups (n = 36 per group). Group I animals were induced to form ascites by injection of Ehrlich ascites carcinoma (EAC) cells (1 × 106 cells/mouse, IP) and kept as untreated controls. Group II animals were treated with A. lanata extract at a dose of 10 mg/kg BW/day, IP (note: dose selected based on results of toxicity study above) for 5 consecutive days. Group III mice received five daily doses of A. lanata and then EAC cells (1 × 106 cells/animal, IP) 24 h after the final extract treatment. Animals in all of the above-mentioned groups were then sacrificed at different time intervals (n = 3/timepoint/group), beginning at 24 h post-tumor induction. One additional group (Group IV, n = 6) contained naïve mice without any treatment at all, and was sacrificed at the first timepoint with the other groups. In all cases, after euthanization, spleen and blood were collected; spleens were processed to yield single cell suspensions using standard protocols (Kruisbeek, Citation2003) that in turn were used as effector cells in NK activity and antibody-dependent cell-mediated cytotoxicity (ADCC) assays. Serum was isolated from the blood for use in the estimation of antibody-dependent complement-mediated cytotoxicity (ACC).
Determination of the effect of A. lanata on NK cell activity
NK cell activity was determined using a 4-h [51Cr] release assay (Kim et al., Citation1980) and K-562 cells as targets. Briefly, [51Cr]-labeled K-562 cells (1 × 104 cells/well) were incubated with spleen (effector) cells (effector:target [E:T] ratio = 100:1) in 96-well round-bottom titre plates for 4 h at 37°C. Total and spontaneous releases were determined by incubating target cells in the presence and absence of 1 N HCl under the same conditions. After this period, culture supernatant was isolated from each well and the released [51Cr] in this material was counted in a g-counter (Perkin Elmer, Waltham, MA). The experiment was performed in triplicate and percentage cell lysis was calculated as:
Determination of the effect of A. lanata on ADCC
ADCC activity was determined using a 4-h [51Cr] release assay (Kim et al., Citation1980). Spleen cells were used as the effector cells and [51Cr]-labeled SRBC were used as the target cells. Anti-SRBC antibody was raised in rabbits and was used as a source of antibody in the ADCC assay. The labeled target cells (1 × 104 cells/well) were incubated with effector cells in 96-well round-bottom titre plates for 4 h at 37°C (E:T ratio = 100:1), along with 0.1 ml of anti-SRBC antibody. After this period, aliquots of culture supernatant were isolated, the released [51Cr] in this material counted in the g-counter, and the percent lysis calculated as above.
Determination of the effect of A. lanata on ACC
The serum isolated from the mice on various days was heat-inactivated at 56°C for 30 min. Fresh rabbit serum diluted with RPMI 1640 was used as a source of complement. To an aliquot (1 ml) of serially-diluted sera sample (in RPMI 1640), 100 µl of EAC (1 × 104 cells) and 50 µl of complement were added. The reaction final volume was made up to 2 ml with RPMI 1640 and the sample incubated at 37°C for 3 h. Samples with cells alone, cells treated with complement, and cells treated with antibody were maintained in parallel as controls. Cytotoxicity was then assessed by a trypan blue exclusion method (Gupta and Bhattacharya, Citation1983). All tests were performed in triplicate.
Determination of the effect of A. lanata on serum cytokine levels
BALB/c mice were divided into four groups (n = 6/group). Group I animals were kept as normal controls. Group II mice were treated with A. lanata (10 mg/kg BW/day, IP) for 5 consecutive days. Group III animals received EAC cells (1 × 106 cells/animal, IP) and were kept as untreated controls. Group IV mice received EAC cells (1 × 106 cells/animal, IP) and then were treated with five daily doses of A. lanata. All groups of mice were sacrificed 24 h after the final dose of extract; blood was collected and serum separated for use in the estimation of IL-2 and IFNg. Quantitative ELISA kits were used for these analyses and manufacturer protocols were followed throughout. The sensitivities of each kit were 3 pg/ml for IL-2 and < 10 pg/ml for IFNg.
Effect of A. lanata on CTL generation
Allo-immunization
Alloimmunization was carried out by injecting spleen cells (2 × 107 cells) from C57BL/6 mice subcutaneously to BALB/c mice. Three systems were used to generate effector cells.
Allo-immunized mice were treated with five doses of A. lanata. Control animals were kept without further treatment. The effector cells were isolated from these animals 7 days after allo-immunization (System A).
Effector cells were produced by a 5-day mixed-lymphocyte culture (MLC) of spleen cells (responder cells from A. lanata-treated and -untreated mice) with mitomycin C (50 µg/ml)-treated spleen cells (stimulator cells) of C57BL/6 mice (System B).
Induction of CTL (effector cells) production (System C) was performed by culturing splenic lymphocyte (2 × 107 cells) from normal BALB/c mice (responder cells) and mitomycin C (50 µg/ml)-treated spleen cells (1 × 107 cells, effector cells) from C57BL/6 at 37°C in a 5% CO2 atmosphere for 5 days, in the presence and absence of A. lanata.
Winn’s neutralization test
Allospecific CTL activity was assayed by Winn’s neutralization test according to the method of Kobayashi et al. (Citation1990). For this, 1 × 107 alloimunized spleen cells from BALB/c mice (effector cells) were mixed with 5 × 105 EL4 cells (target cells) in RPMI medium at an E:T ratio of 20:1. The cells were incubated for 1 h at 37°C in a 5% CO2 atmosphere, and 5 × 104 cells were then injected IP into BALB/c mice. The mice were then observed daily to monitor survival. Survival times of treated mice were ultimately compared against those of mice that received tumor cells only. Significant increases in mean survival days of the treated hosts compared with the tumor control animals were considered indicative of enhanced CTL activity.
Effect of A. lanata on in vivo generation of CTL
Six groups of BALB/c mice (n = 6/group) were used for this study. Group I animals received EL4 cells alone (5 × 104 cells/0.1 ml, IP). Group II hosts received EL4 (5 × 104 cells/0.1 ml IP) and five doses of A. lanata. Group III animals received EL4 cells that had been incubated with spleen cells from normal allo-immunized animals (System A). Group IV mice received EL4-incubated normal allo-immunized spleen cells and continued to receive A. lanata for 5 additional days. Animals in Groups V and VI received EL4 cells that had been incubated with spleen cells from A. lanata-treated allo-immunized mice. One group (Group V) of animals was kept without any further treatment, while the other (Group VI) received five doses of A. lanata. All animals were observed for survival (time). Blood was collected via tail-vein bleeding from each animal 2 days after the final dose of A. lanata, and levels of IL-2 and IFNg were assessed as outlined above.
Effect of A. lanata on CTL (in vitro) production
CTL was generated in vitro by two methods (i.e., Systems B and C) as described above. In System B, five groups of animals were used (n = 6/group). Group I animals received EL4 cells alone (5 × 104 cells/0.1 ml). Group II received EL4 cells that had been incubated with effector cells generated using normal spleen cells in System B. Group III animals were similar to those of Group II, but continued to receive A. lanata treatment for a further 5 days. Group IV animals received EL4 that had been incubated with effector cells generated using spleen cells from A. lanata-treated spleen cells, in System B, whereas Group V animals were similar to those of Group IV, but continued to receive A. lanata for a further 5 days.
In System C, three groups of animals were used. Group I received EL4 cells alone. Group II received EL4 cells incubated with normal co-cultured spleen cells. Group III received EL4 cells incubated with co-cultured spleen cells in the presence of A. lanata. All animals were observed for their survival after these treatments.
Statistical analysis
Values are expressed as mean ± SD. The statistical analysis was done with a one-way analysis of variance (ANOVA) and a Tukey’s post-hoc test using InStat software (Graphpad Software Inc., La Jolla, CA). Significance was accepted at p-values < 0.05.
Results
Toxicity evaluation of ethanolic extract of A. lanata
The no-observed-adverse-effect level (NOAEL) for A. lanata extract was 50 mg/kg BW (Alexeeff et al., Citation2002) (). A. lanata extract at a dose of 100 mg/kg caused observable toxicity, i.e., behavior changes, increased mortality, weight loss, and alterations in renal functions. Short-term administration of A. lanata extract at doses 5, 10, and 50 mg/kg body weight for 14 days did not produce any mortality, change in behavior, body weight, relative organ weight, or hepatic/renal functions when compared with those values in untreated control mice. However, as there was a non-significant increase in blood urea levels in the 50 mg/kg BW group (unpublished data), we selected a dose of 10 mg/kg BW for use in the in vivo studies here-after in order to mitigate the potential for confounding nephrotoxicity impacting our results.
Table 1. Toxicity profile of ethanolic extract of A. lanata.
Effect on NK cell activity
The effect of A. lanata on NK cell-mediated cytotoxicity of normal and tumor-bearing animals is shown in . The percentage cell lysis of target cells mediated by NK cell activity in naïve mice was 7.84% (± 1.25; mean ± SD). Administration of A. lanata significantly enhanced NK cell activity in tumor-bearing as well as non-tumor-bearing hosts; this occurred much earlier when compared with tumor-only controls. In the case of A. lanata-treated tumor-bearing mice, maximum lysis of target cells (i.e., 47.05%) was seen on Day 5 after tumor induction, whereas in tumor-only control animals, the peak lysis (i.e., 18.82%) was observed only on Day 9. Normal animals treated with A. lanata only also showed an earlier enhancement of NK activity and the peak activity (i.e., 44.64%) was observed on Day 5.
Figure 1. Effect of A. lanata on natural killer cell activity. BALB/c mice were divided into three groups (n = 36/group). Group I animals were induced to form ascites by IP injection of Ehrlich ascites carcinoma cells (EAC, 1 × 106 cells/mouse) and kept as untreated tumor-bearing controls. Group II animals were treated with A. lanata extract (10 mg/kg BW/day, IP) for 5 consecutive days. Group III mice received five daily doses of A. lanata and then EAC cells (1 × 106 cells/animal, IP) 24 h after the final extract treatment. Animals were then sacrificed at different timepoints (n = 3/group/timepoint) thereafter. At necropsy, the spleen was removed and processed to generate single-cell suspensions; these materials were then used as effector cells to determine NK cell activity in a [51Cr]-release assay. Results are expressed as mean (± SD) percentage cell lysis.
![Figure 1. Effect of A. lanata on natural killer cell activity. BALB/c mice were divided into three groups (n = 36/group). Group I animals were induced to form ascites by IP injection of Ehrlich ascites carcinoma cells (EAC, 1 × 106 cells/mouse) and kept as untreated tumor-bearing controls. Group II animals were treated with A. lanata extract (10 mg/kg BW/day, IP) for 5 consecutive days. Group III mice received five daily doses of A. lanata and then EAC cells (1 × 106 cells/animal, IP) 24 h after the final extract treatment. Animals were then sacrificed at different timepoints (n = 3/group/timepoint) thereafter. At necropsy, the spleen was removed and processed to generate single-cell suspensions; these materials were then used as effector cells to determine NK cell activity in a [51Cr]-release assay. Results are expressed as mean (± SD) percentage cell lysis.](/cms/asset/a8637aa3-d90a-4645-a91b-bed401b4def6/iimt_a_609191_f0001_b.gif)
Effect on ADCC
Treatment with A. lanata significantly augmented ADCC in tumor-bearing as well as normal animals (). The percent cell lysis of target cells mediated by ADCC in naïve mice was 1.69% (± 0.47). In A. lanata-treated tumor-bearing hosts, maximum lysis of target cells (i.e., 43.30%) was seen on Day 9 post-tumor induction, whereas in tumor control mice the maximum activity (i.e., 14.80%) was observed only on Day 15. Peak lysis of target cells (i.e., 39.90%) in A. lanata-treated non-tumor-bearing animals was observed on Day 9.
Figure 2. Effect of A. lanata on antibody-dependent cellular cytotoxicity. BALB/c mice were divided into three groups (n = 36 per group) and treated as outlined in the legend to . Mice were then sacrificed at different timepoints (n = 3 per group/timepoint) after tumor induction. At necropsy, the spleen was removed and processed to single-cell suspensions; these materials were then used as effector cells to determine ADCC in a [51Cr]-release assay. Results are expressed as mean (± SD) percentage cell lysis.
![Figure 2. Effect of A. lanata on antibody-dependent cellular cytotoxicity. BALB/c mice were divided into three groups (n = 36 per group) and treated as outlined in the legend to Figure 1. Mice were then sacrificed at different timepoints (n = 3 per group/timepoint) after tumor induction. At necropsy, the spleen was removed and processed to single-cell suspensions; these materials were then used as effector cells to determine ADCC in a [51Cr]-release assay. Results are expressed as mean (± SD) percentage cell lysis.](/cms/asset/4f24f95b-32d0-4ec4-8418-75315c2f068a/iimt_a_609191_f0002_b.gif)
Effect on ACC
The effect of A. lanata on ACC is given in . A. lanata treatment significantly enhanced ACC in tumor-bearing as well as non-tumor-bearing mice. The percentage cell lysis of target cells mediated by ACC in naïve mice was 0.37% (± 0.06). In tumor-only controls, maximum cell lysis (i.e., 13.20%) was observed only on Day 17 post-tumor induction; a maximum cell lysis of 27.90% was noted in the A. lanata-treated tumor-bearing mice on Day 15.
Figure 3. Effect of A. lanata on antibody-dependent complement-mediated cytotoxicity. BALB/c mice were divided into three groups (n = 36 per group) and treated as outlined in the legend to . Mice were then sacrificed at different timepoints (n = 3 per group/timepoint) after tumor induction. At necropsy, blood was collected and serum then separated and incubated at 37°C for 3 h with EAC cells (1 × 106 cells/100 µl) and complement (50 µl, neat). The extent of cytotoxicity was then assessed by a trypan blue exclusion method. Results are expressed as mean (± SD) percentage cell lysis.
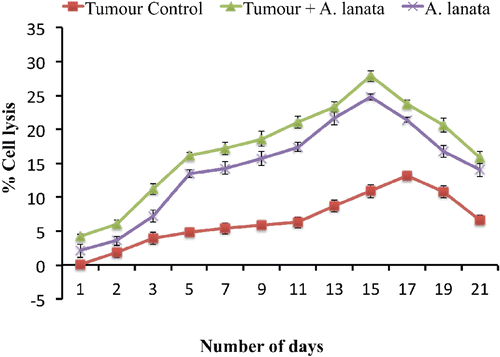
Effect on serum cytokine levels
The effect of A. lanata on serum cytokine profile is presented in . Administration of A. lanata significantly augmented serum IL-2 and IFNg levels when compared to those levels in the blood of normal (untreated/non-tumor-bearing) mice. In the tumor-only control group, IL-2 and IFNg levels were markedly reduced on Day 6 after tumor inoculation, whereas treatment with A. lanata restored IL-2 and IFNg production in tumor-bearing mice.
Table 2. Effect of A. lanata on serum cytokine profile.
Effect of A. lanata on in vivo CTL generation and survival of animals (System A)
The effect of A. lanata on CTL generation in vivo is given in . Administration of A. lanata significantly enhanced the lifespan of tumor-bearing hosts. The lifespan of untreated EL4 tumor-bearing animals was 31.4 days. Administration of A. lanata significantly increased life-span (ILS) to 43.4 days (an increase of 38.22%), whereas mice injected with EL4 cells that had been incubated with normal allo-immunized effector cells had an increased lifespan of only 37.8 days (%ILS = 20.4). When these animals were continuously treated with A. lanata for 5 more days, the survival time increased to 45.3 days (%ILS = 44.3). Animals injected with EL4 cells incubated with alloimmunized spleen cells from A. lanata-treated mice also showed enhanced survival (%ILS = 48.7). The lifespan of animals that received EL4 cells incubated with A. lanata-treated alloimmunized spleen cells was further increased to 54.6 days by the continued administration of A. lanata.
Table 3. Effect of A. lanata on in vivo CTL generation and survival of animals (System A).
Effect of A. lanata on in vitro CTL generation and survival of animals (System B)
The effect of A. lanata on the in vitro CTL generation by System B is presented in . The animals carrying EL4 cells survived for 31.4 days. The lifespan was increased when mice received EL4 cells that had been incubated with effector cells from co-cultured normal spleen cells (%ILS = 26.4). Administration of A. lanata further increased the life-span of the above animal groups to 43.0%. Survival was increased when the mice received EL4 cells that had been incubated with effector cells from A. lanata-treated spleen cells (50.3%). Lifespan was increased to a maximum of 71.9% when these hosts received A. lanata for an additional 5 days.
Table 4. Effect of A. lanata on in vitro CTL generation and survival of animals (System B).
Effect of A. lanata on in vitro CTL production and survival of animals (System C)
The effect of A. lanata on in vitro CTL production and survival of animals (System C) is presented in . The survival time (in days) of control animals treated with EL4 cells alone was 31.4 days. Life-span was increased when animals received EL4 cells incubated with co-cultured normal spleen cells (39.7 days). For the animals that received EL4 cells incubated with co-cultured normal spleen cells in the presence of A. lanata, survival time was increased − with a maximum percentage increase in life-span of 67.5%.
Table 5. Effect of A. lanata on in vitro CTL production and survival of animals (System C).
Effect of A. lanata on IL-2 and IFNγ production during in vivo CTL generation (System A)
The effect of A. lanata on IL-2 and IFNg production during in vivo CTL generation (System A) is presented in . Serum IL-2 and IFNg levels were decreased during tumor progression. Administration of A. lanata significantly enhanced the level of these cytokines in the host serum. Serum IL-2 and IFNg levels were further increased by treatment of A. lanata along with alloimmunized spleen cells. The level of serum IL-2 and IFNg in animals that received EL4 with A. lanata-treated alloimmunized spleen cells was again enhanced by the continued treatment of A. lanata for 5 days.
Table 6. Effect of A. lanata on cytokine production during in vivo CTL generation (System A).
Discussion
Cell- and humoral-mediated immune responses assume different roles in protecting the host. The primary domain of humoral mediated protection lies outside the cell. If antibodies were the only agents of immunity, pathogens that managed to evade them and colonize the intra-cellular environment would escape the immune system. Cell-mediated immune responses detect and eliminate cells that harbor intracellular pathogens, such as tumor cells that express antigens not typical of normal cells (Kindt et al., Citation2006). Earlier studies from our laboratory have shown that the administration of immunomodu-latory agents such as Withania somnifera extract (Davis and Kuttan, Citation2002a; b), Andrographis paniculata extract, and andrographolide (Sheeja and Kuttan, Citation2007a,b; 2010) augmented the anti-tumor activity of various effector cells such as NK cells and CTL, thereby mediating tumor rejection in experimental animals.
Tumor cells evade host defense mechanisms by the production of soluble factors that down-regulate the function of lymphocytes, macrophages, and NK cells (Nelson and Nelson, Citation1987). NK cells are large granular lymphoid cells derived from bone marrow that share a common early progenitor with T-lymphocytes and play an important role in immunological surveillance in neoplasia and metastasis (Cooper et al., Citation2001). Growth of Ehrlich ascites carcinoma (EAC)-induced ascites tumor resulted in dysfunction of T-lymphocyte and NK cell immunosurveillance, as evident from the untreated tumor control animals. NK cell-mediated target cell lysis is very minimal in naïve animals. Experimental data shows that treatment with A. lanata significantly increased the NK cell activity in normal as well as tumor-bearing animals. This may be due to their capacity to produce cytokines, particularly IFNg (Thejass and Kuttan, Citation2006). The lymphokine IL-2, which was identified as T-lymphocyte growth factor, alone or in combination with interferons, are capable of promoting the lytic activity of NK cells (Kuylenstierna et al., Citation2011). Administration of A. lanata significantly enhanced the production of IFNg and IL-2 in normal as well as tumor-bearing mice, and this in turn contributes to NK cell-mediated tumor cell destruction.
Natural killer cells have Fc receptors (CD16) on their surface that allow them to kill antibody-coated target cells (during antibody-dependent cell-mediated cytotoxicity, ADCC), thus providing another form of immune response (Qu and Li, Citation2010). The ethanolic extract of A. lanata is already known to increase the levels of circulating antibody (Siveen and Kuttan, Citation2011a). The elevated NK cell activity due to A. lanata administration resulted in a significant enhancement of ADCC in both normal and tumor-bearing animals when compared to naïve animals, whereas tumor control animals with low NK cell activity showed diminished ADCC activity. A similar enhancement of ACC by the administration A. lanata indicates the activation of a cell-mediated immune system.
Cytotoxic T-lymphocytes (CTL), major effector cells, have lytic capabilities and are critical in the recognition and elimination of virus-infected cells and tumor cells as well as in graft-rejection reactions. CTL can be induced in vitro by the use of the classic mixed-lymphocyte reaction (MLR), during which an allogeneic T-cell-mediated immune response is produced. In the MLR, allogeneic spleen or lymph node cells act as the antigens that stimulate the induction of CTL. The antigens against which these CTL are generated are of the major histocompatibility (H-2) complex. In vivo generation of CTL is achieved by immunization of mice with allogeneic lymphocytes (alloimmunization). These allospecific CTL are capable of lysing a variety of tumor cells in vitro (Kobayashi et al., Citation1990).
EL4 is a dimethylbenzanthracine-induced lymphoma of a C57BL/6 murine host (Gorer, Citation1950). EL4-bearing animals have shown overall decreased immune responses, especially cell-mediated immune response due to impaired generation of CTL (Win et al., Citation2002). Our present study was carried out to determine if there was an increased production of CTL induced by A. lanata. We have used three different modalities for the generation of CTL. In System A, CTL were generated in vivo by allo-immunization by spleen cells from C57BL/6 mice injected subcutaneously into other BALB/c mice. In System B, CTL were generated by the mixed lymphocyte culture (MLC) of spleen cells from allogenic strains of mice, one of which was treated with A. lanata. In System C, CTL were generated by MLC as in System B− but the A. lanata was added to the in vitro culture system. The three modalities were done to confirm that A. lanata exerted its effects on CTL generation under both in vivo and in vitro conditions. The activity of effector cells generated by in vivo as well as in vitro systems was determined by a Winn’s neutralization assay. The increased lifespan of A. lanata-treated animals compared with controls suggests that A. lanata could increase the production of CTL and thereby enhance the killing of the tumor cells. The life-span of the hosts was increased by A. lanata during in vitro treatment in Systems B and C. The increase in the life-span of the animals was directly proportional to the augmentation of CTL activity by A. lanata.
The ability of CTL to produce lymphokines such as IFNg, which are capable of inducing the generation of other potential anti-tumor effector cells, indicates that CTL can non-specifically participate in anti-tumor responses of the host (Kobayashi et al., Citation1990). Enhanced production of IL-2 and IFNg was observed in animals carrying EL4 in vivo-generated CTL by the administration of A. lanata. This observation indicated that these cytokines are responsible for the increased CTL activity that in turn kills the tumor cells.
Our present study demonstrated that Aerva lanata extract could increase NK cell and cytotoxic T-lymphocyte activity in BALB/c mice through enhanced release of IL-2 and IFNg. Tumor cell lysis mediated by NK, ADCC, ACC, and CTL activity was increased, leading to an increase in the life spans of A. lanata-treated tumor-bearing animals. Further studies using isolated compounds are in progress to identify the active principle and to determine the exact mechanism of action. We have screened several compounds among those listed earlier in the Introduction (e.g., 10-hydroxy-canthin-6-one, 10-methoxy-canthin-6-one, β-carboline-1-propionate, canthin-6-one); these preliminary studies have suggested that 10-methoxy-canthin-6-one might be an/the active ingredient in Aerva lanata that produces the above-noted (and other) effects on the immune system (Siveen and Kuttan, in press). As that work is not completed, we cannot at this time say with surety that 10-methoxy canthin-6-one itself is the only active constituent; however, our studies are clear in showing that A. lanata does impart immunomodulatory effects in situ.
Acknowledgements
The authors are sincerely thankful to Dr Ramadasan Kuttan, Research Director, Amala Cancer Research Centre, for his valuable suggestions.
Declaration of interest
The authors report no conflicts of interest. The authors alone are responsible for the content and writing of the paper.
References
- Alexeeff G. V., Broadwin R., Liaw J., and Dawson S.V. 2002. Characterization of the LOAEL-to-NOAEL uncertainty factor for mild adverse effects from acute inhalation exposures. Regul. Toxicol. Pharmacol. 36:96–105.
- Atkinson J. M., Siller C. S., and Gill J.H. 2008. Tumour endoproteases: The cutting edge of cancer drug delivery? Br. J. Pharmacol. 153:1344–1352.
- Bergmeyer H., and Bernt E. 1980. Colorimetric method for aspartate aniline aminotransferases. In: Practical Clinical Biochemistry, 5th Edition (Varley H., Gowenlock A., and Bell M., Eds.), London: William Heinemann Medical Books Ltd., pp. 741–742.
- Chopra R. N. (Ed.) 1956. Glossary of Indian medicinal plants. New Delhi: Council of Scientific & Industrial Research, pp. 329.
- Cooper M. A., Fehniger T. A., and Caligiuri M.A. 2001. The biology of human natural killer cell subsets. Trends Immunol. 22:633–640.
- Davis L., and Kuttan G. 2002a. Effect of Withania somnifera on cell-mediated immune responses in mice. J. Exp. Clin. Cancer Res. 21:585–590.
- Davis L., and Kuttan G. 2002b. Effect of Withania somnifera on CTL activity. J. Exp. Clin. Cancer Res. 21:115–118.
- Gorer P. 1950. Studies in antibody response of mice to tumour inoculation. Br. J. Cancer. 4:372–379.
- Gupta S. K., and Bhattacharya B. 1983. Cytotoxicity assays. In: A Handbook of Practical Immunology (Talwar G. P., Ed.), New Delhi: Vikas Publishing House, pp. 328–333.
- Jemal A., Tiwari R. C., Murray T., Ghafoor A., Samuels A., Ward E., Feuer E. J., and Thun M. J. 2004. Cancer statistics, 2004. CA Cancer J. Clin. 54:8.
- Kim Y., Huh N., Koren H., and Amos D. 1980. Natural killing (NK) and antibody-dependent cellular cytotoxicity (ADCC) in specific pathogen-free (SPF) miniature swine and germfree piglets. I. Comparison of NK and ADCC. J. Immunol. 125:755–762.
- Kindt T. J., Goldsby R. A., Osborne B. A., and Kuby J. (Eds.) 2006. Cell-mediated effector responses. In: Kuby Immunology 5th Edition. New York: W.H. Freeman, pp. 319–337.
- King J. 1965. The phosphatases. In: Practical Clinical Enzymology (Van D., Ed.), London: Nostrand Company Ltd., pp. 91–208.
- Kobayashi H., Aso H., Ishida N., Maeda H., Pollard R. B., and Suzuki F. 1990. Depletion of macrophages expressing IJ antigen results in efficient generation of alloreactive cytotoxic T-lymphocytes. Cell. Immunol. 128:589–602.
- Kruisbeek A. M. 2003. Isolation and fractionation of mononuclear cell populations. In: Current Protocols in Immunology (Coligan J. E.., Ed.), New York: John Wiley and Sons, pp. 3.1.1–3.1.5.
- Kuylenstierna C., Snyder-Cappione J. E., Loo C. P., Long B. R., Gonzalez V. D., Michaelsson J., Moll M., Spotts G., Hecht F. M., Nixon D. F., and Sandberg J. K. 2011. NK cells and CD1d-restricted NKT cells respond in different ways with divergent kinetics to IL-2 treatment in primary HIV-1 infection. Scand. J. Immunol. 73:141–146.
- Murray R. 1984. Non-protein compounds. In: Clinical Chemistry: Theory, Analysis and Co-relation (Kaplan L., and Pesce A., Eds.), Toronto: Mosby C.V., pp. 1230–1268.
- Nelson D., and Nelson M. 1987. Evasion of host defences by tumours. Immunol. Cell Biol. 65:287–304.
- Nevin K. G., and Vijayammal P. L. 2003. Effect of Aerva lanata on solid tumor induced by DLA cells in mice. Fitoterapia 74:578–582.
- Qu Y., and Li Y. 2010. Progress of study on anti-tumor effects of antibody-dependent cell-mediated cytotoxicity - review. J. Exp. Hematol. Chinese Assoc. Pathophysiol. 18:1370.
- Sheeja K., and Kuttan G. 2007a. Activation of cytotoxic T-lymphocyte responses and attenuation of tumor growth in vivo by Andrographis paniculata extract and andrographolide. Immunopharmacol. Immunotoxicol. 29:81–93.
- Sheeja K., and Kuttan G. 2007b. Modulation of natural killer cell activity, antibody-dependent cellular cytotoxicity, and antibody-dependent complement-mediated cytotoxicity by andrographolide in normal and Ehrlich ascites carcinoma-bearing mice. Integr. Cancer Ther. 6:66–73.
- Sheeja K., and Kuttan G. 2010. Andrographis paniculata down-regulates pro-inflammatory cytokine production and augments cell-mediated immune response in metastatic tumor-bearing mice. Asian Pac. J. Cancer Prev. 11:723–729.
- Siveen K. S., and Kuttan G. 2011a. Immunomodulatory and anti-tumor activity of Aerva lanata ethanolic extract. Immunopharmacol. Immunotoxicol. 33:423–432.
- Siveen K. S., and Kuttan G. 2011b. Modulation of humoral immune responses and inhibition of pro-inflammatory cytokines and nitric oxide production by 10-methoxycanthin-6-one. Immunopharmacol. Immunotoxicol. (in press).
- Thejass P., and Kuttan G. 2006. Augmentation of natural killer cell and antibody-dependent cellular cytotoxicity in BALB/c mice by sulforaphane, a naturally-occurring isothiocyanate from broccoli through enhanced production of cytokines IL-2 and IFN. Immunopharmacol. Immunotoxicol. 28:443–457.
- Thejass P., and Kuttan G. 2007. Immunomodulatory activity of sulforaphane, a naturally occurring isothiocyanate from broccoli (Brassica oleracea). Phytomedicine 14:538–545.
- Toro G., and Ackermann P. (Eds.) 1975. Practical Clinical Chemistry. Boston: Little Brown & Co., pp. 154.
- Vetrichelvan T., and Jegadeesan M. 2002. Anti-diabetic activity of alcoholic extract of Aerva lanata (L.) Juss. Ex Schultes in rats. J. Ethnopharmacol. 80:103–107.
- Win S., Uenaka A., and Nakayama E. 2002. Immune responses against allogeneic and syngeneic tumors in aged C57BL/6 mice. Microbiol. Immunol. 46:513–519.
- Zapesochnaya G., Kurkin V., Okhanov V., and Miroshnikov A. 1992. Canthin-6-one and β-carboline alkaloids from Aerva lanata. Planta Med. 58:192–195.
- Zapesochnaya G., Pervykh L., and Kurkin V. 1991. A study of the herb Aerva lanata. III. Alkaloids. Chem. Nat. Compd. 27:336–340.