Abstract
This study aimed to investigate the phagocytic potential of macrophages in progeny of breeder hens kept on an OTA-contaminated diet. For this purpose, 84 White Leghorn (WL) layer breeder hens (40-weeks-of-age) were divided into seven groups (A–G). Hens in Group A were fed a commercial layer ration while those in Groups B–G were kept on a diet amended with 0.1, 0.5, 1.0, 3.0, 5.0, or 10.0 mg OTA/kg, respectively, for up to 3 weeks (n = 12/treatment group; n = 4/time sub-group/treatment group). Fertile eggs were set for hatching on a weekly basis to get the progeny of each week separately. Hatched chicks (n = 10 from each group) were injected with India ink at day 14-of-age to study the in vivo phagocytosis of carbon particles. At day 30, abdominal macrophages were collected from 15 chicks/group and were used to assess their ex vivo/in vitro phagocytic potential against sheep red blood cells (SRBC) as well as for nitrite production upon challenge with lipopolysaccharide (LPS). The phagocytic indices of the reticuloendothelial system of all three sets of progeny (chicks obtained from hens fed OTA for 7, 14, and 21 days) were significantly lower than values seen with Group A chicks. The number of macrophages that were actively phagocytic, the number of SRBC internalized per macrophage, and the extent of nitrite production after stimulation with LPS were each significantly lower in the cells obtained from chicks of breeder hens that had been maintained on the OTA-contaminated diets. The findings of this study clearly showed that there are immunosuppressive effects—in terms of depressed in vivo and in vitro macrophage functionality—in progeny of OTA-fed breeder hens.
Introduction
Mycotoxins, the secondary metabolites of some toxigenic fungi, have remained a topic of interest for researchers since their initial discovery. The list of significant mycotoxins for live-stock and human health concern includes aflatoxins, ochratoxins, zearalenone, T-2 toxins, deoxynivalenol, patulin, and fumonisin (Price et al., Citation1993; Kpodo et al., Citation1996; Atkins and Norman, Citation1998; Abdulkadar et al., Citation2004; Agag, Citation2004; Magnoli et al., Citation2007). Human exposure results primarily from: (I) direct consumption of mycotoxin-contaminated foods; (II) ingestion of mycotoxin by-products obtained by consumption of animals (associated products, i.e., meat, eggs, milk, etc.) that had been fed contaminated rations; and, (III) passage from mother to child through breast milk (Frye and Chu, Citation1978; Zimmerli and Dick, Citation1995; Rizzo et al., Citation2002; Navas et al., Citation2005; Hassan et al., Citation2006; Almela et al., Citation2007; Binder et al., Citation2007).
Ochratoxins are characterized by a wide range of toxicological effects. Ochratoxin A (OTA), in particular, is well known for its nephrotoxic activity (Huff et al., Citation1975; Glahn, Citation1993; Wafa et al., Citation1998; Stoev et al., Citation2002; Dinis et al., Citation2007). OTA is also considered to be hepatotoxic, immunotoxic, neurotoxic, teratogenic, genotoxic, and carcinogenic (Group 2B human possible carcinogen (IARC, Citation1993)) (Kumar et al., Citation2004; Elaroussi et al., Citation2006; Sava et al., Citation2006; Koynarski et al., Citation2007; Wangikar et al., Citation2007). OTA in cereal/grains (Hanif et al., Citation2006; Zinedine et al., Citation2006; Liu et al., Citation2007) has been seen to be found in commercial poultry feed (Kichou and Walser, Citation1993; Dalcero et al., Citation1998; Rosa et al., Citation2006) and, as a result, in the tissue and eggs of these exposed birds (Niemiec et al., Citation1994; Biro et al., Citation2002), and, finally, in the blood/plasma of humans (Grosso et al., Citation2003; Dinis et al., Citation2007). The immunotoxic activities of natural and experimental ochratoxicosis have been reported in different mammalian and avian species. A significant depression in cytotoxic T-lymphocyte activity, natural killer cell activity, and bactericidal capability of macrophages was noted in male Wistar rats fed OTA at 450 µg/kg body weight for 28 days (Alvarez et al., Citation2004). Feeding OTA-contaminated diet at 8 µg/g feed for 3 weeks resulted in a depressed phagocytic activity of heterophils in broiler chicks (Chang and Hamilton, Citation1980).
The role of phagocytic cells in the body defense is pivotal, by virtue of their dual role in innate and acquired immunity. On exposure to stimuli, they acquire new functional capabilities, change their cell surface (antigenic) profile, and secrete a series of various products that, in turn, up- or down-regulate activities of other cells. Apart from this accessory function, the secretion of reactive oxygen intermediates and ability to kill pathogens or tumor cells are key roles performed by phagocytic cells. While depression in macrophage phagocytic potential due to ochratoxicosis has been reported by many researchers, the literature is still lacking regarding effects of this mycotoxin on the cells of progeny of animals whose mothers were fed OTA-contaminated feeds.
Therefore, in keeping with this concern, this study was designed to assess the phagocytic potential of the macrophages (both in vivo and in vitro) from the progeny of breeder hens that had been maintained for various periods of time on OTA-contaminated diets. As we have previously reported on the (immuno)toxic effects of these diets in the breeder hens themselves (ul-Hassan et al., Citation2010, Citation2011a), we did not examine them/their cells in detail in the current study.
Materials and methods
Ochratoxin and feed
Lyopholized spores of Aspergillus ochraceus (CECT 2948, Culture Collection Center, University De Valencia, Valencia, Spain) were rehydrated in nutrient broth and inoculated on sterile potatoes dextrose agar (PDA) plates and the plates were incubated at 28°C for 5 days. The colony characteristics of the A. ochraceus were observed; for microscopic studies, slide cultures were stained with lactophenol cotton blue. Spores of A. ochraceus were re-cultured on PDA slants and incubated at 28°C for 2 weeks.
For the production of OTA, wheat grains were used as a substrate as described by Trenk et al. (Citation1971) and ul-Hassan et al. (Citation2011a). Ochratoxin A was extracted from the fermented wheat in acetonitrile-water and quantified using high-performance liquid chromatography (HPLC) and fluorescent detection methods (Bayman et al., Citation2002).
Basal diet for the laying hens (16% total protein and 2700 Kcal/Kg metabolizable energy) was prepared without addition of toxin binder, vitamins, minerals, and antibiotics. Prior to use, each batch of the basal diet was analyzed for ochratoxin, aflatoxin, and zearalenone to ensure that the levels of each were < 1.0 µg/Kg. OTA-contaminated feeds were prepared by incorporation of known quantities of OTA. For this purpose, fermented wheat grains were extracted by soaking in a 3-fold quantity of chloroform (1:3) overnight and then filtered through cotton cloth. All the chloroform was then evaporated and the concentrated residues re-suspended into polyethylene glycol (PEG). This suspension was then evenly mixed in the required quantity of basal feed to prepare the experimental feeds containing each desired concentration of OTA. Prior to being used for feeding, the OTA concentration in each experimental diet was verified by HPLC.
Induction of ochratoxicosis in White Leghorn breeder hens
All animal experiments were conducted according to the rules and regulations of the Animal Ethics Committee, Faculty of Veterinary Science, at the University of Agriculture, Faisalabad (Pakistan). Eighty-four White Leghorn (WL) layer breeder hens (40 weeks-of-age) were procured from a breeder farm (whose birds were maintained under standard environmental conditions and were routinely vaccinated) and then kept in battery cages in the Department of Pathology at the University of Agriculture Faisalabad, Pakistan. Animal rooms were kept at 26°C, with a 65% relative humidity, and with a 16-h/8-h light-dark cycle; all hens had access to fresh water ad libitum. After acclimatization for 1 week on basal layer ration, the hens were divided into seven groups (A–G), each consisting of 12 birds. Hens in Group A were kept on basal layer ration, while those in Groups B, C, D, E, F, and G were fed OTA-contaminated feed at 0.1, 0.5, 1.0, 3.0, 5.0, or 10.0 mg OTA/Kg feed, respectively, for a period of up to 21 days. In this study, there was an average feed intake of ≈ 105 g/hen/day over the course of the experiment. This meant that each bird consumed from 10.5–1050 µg OTA daily (at the corresponding range from the lowest to highest OTA level, respectively) during the 21-day period.
All hens used in this experiment were checked to be certain they did not harbor any infectious organisms (e.g., mycoplasma, Salmonella); hens were also vaccinated to be certain they yielded infection-free eggs/chicks. During the experiment, hen antibody titres against different diseases were also assessed periodically to be certain there were no infectious outbreaks occurring. After hatching, chicks were routinely vaccinated to avoid the chance of possible immunosuppression or interference in the phagocytic process from any source other than the OTA that had potentially been transferred to them from the hens during their maturation in the eggs.
Healthy breeder roosters of WL were maintained on basal layer ration for semen collection. Semen was collected by an abdominal massage method and diluted (1:1) with semen extender (OVODYL, IMV, L’Aigle Cedex, France). Laying hens were inseminated on alternate days by using artificial insemination gun and straws (IMV). Hatching eggs were then collected on a daily basis and set for incubation on a weekly basis. Prior to setting, eggs were stored at 15°C. To verify that OTA has indeed crossed into the eggs, and to begin to discern the kinetics of any deposition, OTA levels were assessed in randomly-selected eggs on alternate days as well as after the withdrawal of OTA-contaminated feed. The egg samples were extracted using the methods of Sizoo and Egmond (Citation2005) and validated in our laboratory for OTA analyses in eggs. Prior to injection onto the HPLC system (described above) for OTA quantitation, each sample was passed through an immunoaffinity column bearing OTA-specific antibodies.
The progeny, which were obtained on a weekly basis, were maintained separately for 30 days under standard environmental conditions and on a basal layer starter ration (21% total protein and 2900 Kcal/Kg metabolizable energy) verified not to contain OTA or Aflatoxin-B1 (AFB1) residues at a level > 1.0 µg/Kg ration. Animal rooms were kept at 33°C during the first week of age (and reduced by ≈2.5°C/week thereafter), a 60% relative humidity, and with a 12-h/12-h light-dark cycle; all chicks had access to fresh water ad libitum.
Assessment of phagocytic activity of reticuloendothelial cells in the peripheral blood (via measures of carbon clearance activity)
At day 14-of-age, 10 chicks/group from each weekly setting were used for the determination of phagocytic activity of their reticuloendothelial systems by measuring clearance of colloidal carbon from the peripheral blood, as described by CitationSugiura et al. (2001) with minor modification. Briefly, Black India Ink (Pelikan, 4001) was centrifuged at 3000 x g for 30 min and the supernatant fraction was collected and then injected into the right wing vein (at 1 ml/Kg body weight [BW]) of each chick. Blood samples (200 µl/chick) were collected from the left wing vein at 3 and 15 min post-ink injection. The collected blood samples were immediately transferred into 4 ml aqueous sodium citrate (1%) solution and then centrifuged for 4 min at 50 x g. Optical density of the supernatant was measured at 675 nm in a Spectro 20D + Rs-232 spectrophotometer (LaboMed, Inc., Culver City, CA). The phagocytic index was calculated according to the following equation:
where t3 = 3 min, t15 = 15 min, ODt3 = optical density at 3 min after the injection of carbon solution, and ODt15 = optical density at 15 min after the injection of carbon solution.
Preparation of peritoneal macrophages for ex vivo experiments
Macrophage functions were assessed following the method of Qureshi and Havenstein (Citation1994) with some modifications (described in ul-Hassan et al., Citation2011b). The total number of abdominal exudate cells (AEC) was counted on a hemacytometer and adjusted to needed levels for use in the experiments outlined below.
(a) Macrophage phagocytic ability
A 1% suspension of sheep red blood cells (SRBC, in RPMI growth medium) was prepared for use as particulate antigen for the AEC. Macrophage monolayers were established by the addition of 1 × 106 AEC/ml from each sample into sterile Petri dishes. After 1 h of incubation, non-adherent cells were washed away. The remaining macrophage monolayers were then co-incubated at 41°C in a humidified 5% CO2 atmosphere for 60 min with a 1.0 ml suspension of SRBC that was added to each dish. After washing away the non-internalized SRBC, the coverslips were then stained with Wright-Giemsa stain. A total of 200 macrophages were then scored for phagocytosis and number of SRBC engulfed/macrophage.
(b) Nitrite production (as index for oxidative killing)
Lipopolysaccharide (LPS, Escherichia coli O55: B5, Sigma)-induced nitrite production by the isolated abdominal macrophages was quantified as described by Green et al. (Citation1982). After 1 h incubation at 41°C in a 5% CO2 atmosphere, the non-adherent cells were removed and the remaining cells were then exposed to LPS (1 µg/well) for 24 h. After the incubation period, an aliquot of 200 µl culture supernatant was collected from each well and each mixed with equal volume of Griess reagent in a corresponding well of a 96-well microtiter plate. The plate was incubated at 37°C for 15 min and the optical density (OD) in each well was then measured at 540 nm in a microplate ELISA reader (Biochrom, Holliston, MA). Nitrite levels in the supernatant samples were then extrapolated from a standard curve that was generated in parallel from known concentration sodium nitrite solutions.
Statistical analysis
All data were subjected to analysis of variance tests. Means of the different groups were compared using a Duncan’s Multiple Range test within a MSTATC statistical package (Department of Crop and Soil Sciences, Michigan State University, East Lansing, MI). Data were considered significantly different from one another at a p-value ≤ 0.05.
Results
Kinetics and dose-relatedness of OTA deposition in eggs
The analyses of OTA levels in randomly-selected eggs from hens maintained on the different OTA-contaminated diets indicated that at the three lowest levels (e.g., 0, 0.1, and 0.5 mg OTA/Kg feed) there were no detectable (i.e., or were below limits of detection) levels of the mycotoxin at any time in the 28-day study period (). Hens fed 1.0 or 3.0 mg OTA/Kg feed achieved maximal measurable levels of 3.6 or 4.5 ng OTA/g egg, respectively, only toward the end of the feeding regimen (i.e., around day 17). In contrast, those hens fed 5.0 or 10.0 mg OTA/Kg feed had quite substantive levels of the mycotoxin in their eggs by day 5. With the hens consuming 5.0 mg OTA/Kg feed, OTA levels in the eggs never seemed to vary much over the period from day 5 to day 22, and dropped somewhat sharply once the toxin had been removed from the hens’ diet (i.e., from day 23 and thereafter). This suggested that there was the possibility that the previously-consumed OTA was being rapidly cleared or, alternatively, sequestered in other body sites in the hens such that transference to the eggs no longer was readily occurring. With the hens in the highest exposure group, i.e., 10.0 mg OTA/Kg feed, the levels of OTA in their eggs appeared to reach a maximum by day 7 of the regimen and remained in the range of 14.6–17.9 ng/g egg up to day 21. As with the other ‘high-dose’ group, levels in eggs dropped over the period after the toxin had been removed from the hens’ diet.
Table 1. OTA residues (ng/g) in eggs of breeder hens fed OTA over a period of 21 days.
The egg data from the 1.0, 3.0, 5.0, and 10.0 mg OTA/Kg feed regimens clearly suggest that the amount of material that can be transferred to the eggs seems ‘saturable’. Whether this is a phenomenon associated with the eggs themselves or with the hens is not clear. It would seem that if the amount of deposition was attributable to a ‘saturation’ effect of other body sites being needed to occur prior to deposition into the eggs, then this point of saturation was most likely attained at the lowest of these four dosages. However, while this effect appears to be both dose- and time-related, the pattern does not remain consistent. For example, while the Group F hens consumed ≈ 1.7-times as much OTA/Kg diet than Group E hens—and their egg burdens eventually reflected this same relative difference (i.e., 8.1 vs 4.5 ng/g on day 17)—hens in Group G consumed twice as much OTA/Kg diet than the Group E hens—but their eggs had 2–3-times the OTA burden. Further investigation of the kinetics of transference is clearly warranted to obtain a better understanding of how this toxin is reaching the offspring of these avian hosts.
Phagocytic index of reticuloendothelial system
The Phagocytic Index (K) of the reticuloendothelial systems of the progeny of breeder hens that had been maintained on OTA-contaminated diets is shown in . The in situ phagocytic activity of chicks obtained from the breeder hens fed OTA-contaminated diets for 7 days were significantly depressed in Groups C, D, E, F, and G offspring as compared to in the chicks belonging to Group A (). The K-values associated with the chicks from breeder hens fed OTA-contaminated feed for 14 days showed similar dose-related outcomes to the chicks from hens kept on the contaminated diet for 7 days, i.e., K-values were significantly lower in the chicks belonging to OTA-fed hens (except for those in Group B) relative to those of Group A chicks (). In contrast, as a result of 21 days of consuming OTA-contaminated feed at any of the test levels, hens yielded chicks who had K-values that were all significantly lower () as compared to the values of the normal hens’ chicks (control).
Figure 1. Phagocytic Index (K) values for the reticuloendothelial system of chicks obtained from breeder hens that had been maintained on OTA contaminated diets. Hens fed OTA-bearing diet for (a) 7, (b) 14, or (c) 21 days. Values shown represent mean (± SD) K-values of 10 chicks/OTA (hen) dose. Value significantly different from control at * p < 0.05, ** p < 0.01, or *** p < 0.001.
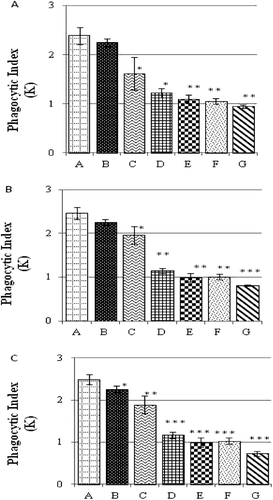
Macrophage functions assays
The peritoneal macrophages of chicks from OTA-treated hens were assessed for both their phagocytic potential (against SRBC) and nitrite production (in response to LPS) (). The percentages of cells showing phagocytic activity were dose-relatedly decreased in the cells from the progeny of breeder hens fed OTA for 7 days. The effect was maximal among the cells from chicks in Group G. Only 17.89% (± 1.02, mean ± SD) of cells from these chicks displayed phagocytic activity against the SRBC challenge; in comparison, cells from chicks in Group A were 27.00% (± 2.00) positive for SRBC uptake. Even when cells did take up the SRBC, there were differences in the relative capacities of those active cells. Specifically, the numbers of SRBC internalized per macrophage were significantly lower in the cells of the chicks from Group G (i.e. 1.42 [± 0.42]) as compared to those for cells from Group A chicks (i.e., 2.36 [± 0.08]). Nitrite production values for macrophages stimulated with LPS also showed dose-related effects of the OTA treatments. Specifically, significantly lower production values were noted in cells from the chicks in Groups E (17.32 [± 5.74]), F (15.69 [± 4.97]), and G (14.83 [± 3.61]) when compared with values noted with chicks from Group A (26.95 [± 3.76]).
Table 2. Macrophage function of chicks obtained from hens fed OTA-contaminated feed for 3 weeks.
A more severe decrease in the function of abdominal macrophages in the progeny of hens kept on OTA-contaminated feed for longer durations, i.e., 21 days, was noted. The number of macrophages showing phagocytic activity were significantly lower in chicks from Groups F (21.11 [± 3.10]%) and G (17.44 [± 2.69]%) compared with the values for cells of chicks from Group A (i.e., 25.66 [± 2.33]%). The number of SRBCs internalized by macrophages harvested from the chicks obtained from the OTA-fed hens (at all feeding levels for 21 days) were also significantly reduced as compared to values associated with Group A cells. The most severe depression was noted in the cells from chicks in Groups F (1.48 [± 0.40] SRBC/macrophage) and G (1.24 [± 0.12]) when compared against the value of 2.67 (± 0.27) for the Group A chick cells. Nitrite production levels by cells in response to LPS were also significantly lower in the chicks from hens fed OTA for 21 days, except in cells from the Group B chicks—these displayed a non-significant difference. The least value of 17.55 (± 2.03) µM was noted in cells from Group G chicks; the highest value of (26.50 [± 4.09] µM was noted with cells from the chicks in Group A.
Discussion
The mycotoxin content in naturally contaminated poultry feed/its ingredients depends on many factors like geographical conditions, the type of mycobiota, and, most importantly, storage conditions associated with the materials to be used in the feed. Keeping in mind this concern and also the controversy reported in the literature regarding the rate of transmission of OTA from hen to eggs, this led us to use a wide range of OTA in the hens’ diets (i.e., from reported non-toxic levels of 0.1 mg/Kg, and then to gradually higher levels of 0.3, 0.5, 1.0, 3.0, 5.0, and ultimately the many-fold toxic level of 10 mg/Kg).
A significant decrease in the in vivo phagocytic index of the chicks obtained from the breeder hens kept on an OTA-contaminated diet was observed as compared to the values shown by the chicks in group A (obtained from the hens kept on basal layer ration). Unfortunately, there is no parallel report in the literature about the phagocytic potential of the reticuloendothelial system of the chicks obtained from the OTA-fed dams. However, Michael et al. (Citation1973) reported the impairment of the reticuloendothelial system of the chicks during aflatoxicosis. The noted depresssion in the phagocytic index of the chicks may be due to cytotoxic effects of OTA in the reticuloendothelial system. The degenerative and apoptotic changes in the immunological organs/cells during ochratoxicosis (Muller et al., Citation1999; Alvarez-Erviti et al., Citation2005; Al-Anati and Petzinger, Citation2006) provide a significant basis to assume the depression in in vivo phagocytic potential of the reticuloendothelial system.
The major cells types capable of phagocytosis in the birds are heterophils, thrombocytes, and macrophages (Lam Citation1998). In this study, the phagocytic potential and nitrite production (in response to LPS stimulation) by peritoneal macrophages harvested from chicks of OTA-fed dams revealed significant depression. There are no studies in the literature describing the effects on the phagocytic potential of macrophages in progeny of hens fed OTA-contaminated diet. However, a significant decrease in the phagocytic potential of heterophils harvested from chicks fed OTA-contaminated diets (at 4 and 8 µg/g feed) was observed by Chang and Hamilton (Citation1980). In one of our recent studies (ul-Hassan et al., Citation2011a), a significant decrease in immunological organ weights and in levels of immunoglobulin(s)-bearing cells was noted in the spleen and bursa of Fabricius of the progeny of breeder hens kept on OTA-contaminated rations.
Although we cannot postulate upon the exact mechanism for the observed effects of OTA on the phagocytic processes in the progeny, there have been some suggestions as to how OTA might act in a host to bring about these/similar changes. These have included: (A) OTA induces ‘lazy leukocyte syndrome’ due to decreased directional and non-directional locomotion of cells (Chang and Hamilton Citation1980); and (B) ochratoxicosis results in a significant depression in the production (i.e., maturation from stem cell to final fully-active/-capable cell) of phagocytic cells, and, as such, results in the appearance of an overall reduction in the phagocytic process in situ (but not necessarily at individual cell level) (Alvarez et al., Citation2004). Unlike in those studies, in the present study the chicks (‘immunologic hosts’) were not directly exposed to OTA—rather, their exposures to OTA occurred pre-natally (i.e., in ovo). It is assumed here that as there was a decrease in the weights of immune system organs in chicks of OTA-fed dams (see our previous studies), effects on the production/maturation of the phagocytic cells in the chicks could not be ruled out. Ongoing studies in our laboratory are seeking to examine bone marrow/surface markers of expression on various phagocytic cell types in the chicks to help resolve this issue and provide a clearer (initial) mechanism of OTA-mediated immunotoxicity following in ovo exposures.
In studies with non-avian hosts, i.e., male Wistar rats, a significant depression in the activity of cytotoxic T-lymphocytes and natural killer cells, and in the bactericidal capabilities of macrophages was noted after feeding OTA at 450 µg/Kg body weight for 28 days (Alvarez et al., Citation2004). The feeding of OTA-contaminated diet has also been shown to result in a significant decrease in chemotactic activity, as well as interleukin (IL)-1 and tumor necrosis factor (TNF)-α production by mice and their isolated peritoneal macrophages (Klinkert et al., Citation1981; Dhuley, Citation1997). Taking together these results in rodent hosts with those in the above-cited studies in fowl, we conclude that the noted decrease in the phagocytic index of the reticuloendothelial system and then in the ex vivo nitrite production and phagocytic activity of heterophils of progeny chicks we observed here may be attributed to transfer of OTA into the hen’s egg that, in turn, ultimately affects development/functionality of the immunological cells in these hatchlings.
Of course, the relevance of these studies to the non-avian situation is an important issue as exposure to mycotoxins, including OTA, routinely might occur under conditions wherein foodstuffs become contaminated in the field or under less-than-ample storage conditions. In an experiment where female Fisher rat dams were fed lead acetate, Miller et al. (Citation1998) reported a significant decrease in the ex vivo and in vivo immunological status of rat progeny who were not exposed to any additional lead apart from that ingested by their dams. Taking into account the similarities in immunotoxic effects from lead in the progeny of lead-fed rat dams and the noted changes in the immunological status of the progeny of breeder hens kept on OTA-contaminated diets, it could be assumed that there might also exist some immunotoxic effects of OTA in the progeny of mammalian hosts accidentally-exposed to OTA in their daily diets. Of course, the different chemistries/solubilities of lead ions as compared to those of the far-bulkier OTA residues might mean that the relative kinetics of exposure/dosing from mother-to-offspring could be vastly different. Future studies in our laboratories and those of colleagues will endeavor to examine the effects from OTA-contaminated diets provided to rodent dams on both the mothers and their offspring. Only then will the true relevance of the current studies with the hens/chicks become much clearer.
Nevertheless, based upon the results of the present study, we conclude that a presence of OTA in rations provided to breeder hens can result in significant depression of the immunological status of their progeny.
Acknowledgments
The authors highly acknowledge the financial support of the Higher Education Commission of Pakistan during the study period.
Declaration of interest
The authors report no conflicts of interest. The authors are alone responsible for the content and writing of the paper.
References
- Abdulkadar A. H., Al-Ali A. A., Al-Kildi A. M., and Al-Jedah J. H. 2004. Mycotoxins in food products available in Qatar. Food Cont. 15:543–548.
- Agag B. I. 2004. Mycotoxins in foods and feeds, 1 - Aflatoxins. Ass. Univ. Bull. Environ. Res. 7:173–205.
- Al-Anati L., and Petzinger E. 2006. Immunotoxic activity of Ochratoxin A. J. Vet. Pharmacol. Ther. 29:79–90.
- Almela L. Rabe V., Sanchez B., Torrella F., Lopez-Perez J. P., Gabaldon J. A., and Guardiola L. 2007. Ochratoxin A in red paprika: Relationship with the origin of the raw material. Food Microbiol. 24:319–327.
- Alvarez L., Gil A. G., Ezpeleta O., Garciajalo J. A., and Cerain A. L. 2004. Immunotoxic effects of Ochratoxin A in the Wistar rats after oral administration. Food Chem. Toxicol. 42:825–834.
- Alvarez-Erviti L., Leache C., González-Peñas E., and de Cerain A. L. 2005. Alterations induced in vitro by Ochratoxin A in rat lymphoid cells. Hum. Exp. Toxicol. 24:459–466.
- Atkins D. and Norman J. 1998. Mycotoxins and food safety. Nutr. Food Sci. 5:260–266.
- Bayman P., Baker J. L., Doster M. A., Michailides T. J., and Mahoney N. E. 2002. Ochratoxin production by Aspergillus ochraceus Group and Aspergillus Alliaceus. Appl. Environ. Microbiol. 68:2326–2329.
- Binder E. M., Tan L. M., Chin L. J., Handle J., and Richard J. 2007. Worldwide occurrence of mycotoxins in commodities, feeds and feed ingredients. Anim. Feed Sci. Tech. 137:265–282.
- Biro K., Solti L., Vetro I. B., Bago G., Glavits R., Szabo E., and Gremmels J. F. 2002. Tissue distribution of ochratoxin A as determined by HPLC and ELISA and histopathological effects in chickens. Avian Pathol. 31:141–148.
- Chang C. F. and Hamilton P. B. 1980. Impairment of phagocytosis by heterophils from chickens during ochratoxicosis. Appl. Environ. Microbiol. 39:572–575.
- Dalcero A., Magnoli C., Luna M., Ancasi G., Reynoso M. M., Chiacchieara S., Miazzo R., and Palacio G. 1998. Mycoflora and naturally occurring mycotoxins in poultry feeds in Argentina. Mycopathologia 141:37–43.
- Dhuley J. N. 1997. Effect of some Indian herbs on macrophage functions in Ochratoxin A treated mice. J. Ethnopharmacol. 58:15–20.
- Dinis A. M. P., Lino C. M., and Pena A. S. 2007. Ochratoxin A in nephropathic patients from two cities of central zone in Portugal. J. Pharma. Biomed. Ana. 44:553–557.
- Elaroussi M. A., Mohamed F. R., El-Barkouky E. M., Atta A. M., Abdou A. M., and Hatab M. H. 2006. Experimental ochratoxicosis in broiler chickens. Avian Pathol. 35:263–269.
- Frye C. E. and Chu F. S. 1978. Distribution of ochratoxin A in chicken tissues and eggs. J. Food Safety 1:147–159.
- Glahn R. P. 1993. Mycotoxins and the avian kidneys: Assessment of physiological function. World’s Poult. Sci. J. 49:242–250.
- Green L. C., Wagner D. A., Glogowski J., Skipper P. L., Wishnok P. S., and Tannebaum S. R. 1982. Analysis of nitrate, nitrite, and [15N]-nitrite in biological fluid. Anal. Biochem. 126:131–138.
- Grosso F., Said S., Mabrouk I., Fremy J. M., Castegnaro M., Jemmali M., and Dragacci S. 2003. New data on the occurrence of Ochratoxin A in human sera from patients affected or not by renal diseases in Tunisia. Food Chem. Toxicol. 41:1133–1140.
- Hanif N. Q., Naseem M., Khatoon S., and Malik N. 2006. Prevalence of mycotoxins in poultry rations. Pak. J. Sci. Indu. Res. 49:120–124.
- Hassan A. M., Sheashaa H. A., Abdel-Fattah M. F., Ibrahim A. Z., Gaber O. A., and Sobh M. A. 2006. Study of Ochratoxin A as an environmental risk that causes renal injury in breast-fed Egyptian infants. Pediatr. Nephrol. 21:102–105.
- Huff W. E., Wyatt R. D., and Hamilton P. B. 1975. Nephrotoxicity of dietary Ochratoxin A in broiler chickens. Appl. Microbial. 30:48–51.
- International Agency for Research on Cancer. 1993. In: IARC Monograph on the Evaluation of Carcinogenic Risks to Humans. Lyon: IARC, pp. 489.
- Kichou F. and Walser M. M. 1993. The natural occurrence of Aflatoxin B1 in Moroccan poultry feed. Vet. Hum.Toxicol. 35:105–108.
- Klinkert W., Lorkowski G., Creppy E. E., Dirheimer G., and Roschenthaler R. 1981. Inhibition of macrophage migration by Ochratoxin A and citrinin, and prevention by phenylalanine of the ochratoxin A-induced inhibition. Toxicol. Euro. Res. 3:185–189.
- Koynarski V., Stoev S., Grozeva N., Mirtcheva T., Daskalov H., Mitev J., and Mantle P. 2007. Experimental coccidiosis provoked by Eimeria acervulina in chicks simultaneously fed on Ochratoxin A-contaminated diet. Res. Vet. Sci. 82:225–231.
- Kpodo K., Serensenb A. K., and Jakobsen M. 1996. The occurrence of mycotoxins in fermented maize products. Food Chem. 56:147–153.
- Kumar A., Jindal N., Shukla C. L., Asrani R. K., Ledoux D. R., and Rottinghaus G. E. 2004. Pathological changes in broiler chickens fed Ochratoxin A and inoculated with Escherichia coli. Avian Pathol. 33:413–417.
- Lam K. M. 1998. Alteration of chicken heterophil and macrophage functions by the infectious bursal disease virus. Microbial Pathogensis 25:147–155.
- Liu R., Yu Z., He Q., and Xu Y. 2007. An immunoassay for Ochratoxin A without the mycotoxin. Food Control 18:872–877.
- Magnoli C., Astoreca A. L., Chiacchiera S. M., and Dalcero A. 2007. Occurrence of Ochratoxin A and ochra-toxigenic mycoflora in corn and corn based foods and feeds in some South American countries. Mycopathologia 163:249–260.
- Michael G. Y., Thaxton J. P., and Hamilton P. B. 1973. Impairment of the reticuloendothelial system of chickens during aflatoxicosis. Poult. Sci. 52:1206–1207.
- Miller T. E., Golemboski K. A., Ha R. S., Bunn T., Sanders F. S., and Dietert R. R. 1998. Developmental exposure to lead causes persistent immunotoxicity in Fisher 344 rats. Toxicol. Sci. 42:129–135.
- Muller G., Kielstein P., Rosner H., Berndt A., Heller H., and Kohler H. 1999. Studies of the influence of Ochratoxin A on immune and defense reactions in weaners. Mycoses 42:495–505.
- Navas S. A., Sabino M., and Rodriguez-Amaya D. B. 2005. Aflatoxin M1 and Ochratoxin A in a human milk bank in the city of Sao Paulo, Brazil. Food Addit. Contam. 22:457–462.
- Niemiec J., Borzemska W., Goliński P., Karpińska E., Szeleszczuk P., and Celeda T. 1994. The effect of Ochratoxin A on egg quality, development of embryos and the level of toxin in eggs and tissues of hens and chicks. J. Anim. Feed Sci. 3:309–316.
- Price W. D., Lovell R. A., and McChesney D. G. 1993. Naturally occurring toxins in feedstuffs: Center for veterinary medicine perspective. J. Anim. Sci. 71:2556–2562.
- Qureshi M. A. and Havenstein G. B. 1994. A comparison of immune performance of a 1991 commercial broiler with a 1957 random-bred strain when fed “typical” 1957 and 1991 broiler diets. Poult. Sci. 73:1805–1812.
- Rizzo A., Eskola M., and Atroshi F. 2002. Ochratoxin A in cereals, foodstuffs and human plasma. Euro. J. Plant Pathol. 108:631–637.
- Rosa C. A., Ribeiro J. M., Fraga M. J., Gatti M., Cavaglieri L. R., Magnoli C. E., Dalcero A. M., and Lopes C. W. 2006. Mycoflora of poultry feeds and ochratoxin-producing ability of isolated Aspergillus and Penicillium species. Vet. Microbiol. 10:89–96.
- Sava V., Reunova O., Velasquez A., Harbison R., and Sanchez-Ramos J. 2006. Acute neurotoxic effects of fungal metabolite Ochratoxin A. Neurotoxicol. 27:82–92.
- Sizoo E. A. and Egmond H. V. 2005. Analysis of duplicate 24-hour diet samples for Aflatoxin B1, Aflatoxin M1, and Ochratoxin A. Food Addit. Contam. 22:163–172.
- Stoev S. D., Daskalov H., Radic B., Domijan A., and Peraica M. 2002. Spontaneous mycotoxic nephropathy in Bulgarian chickens with un-clarified mycotoxin etiology. Vet. Res. 33:83–93.
- Sugiura H., Inaba R., Iwata H., Nishida H., and Tanaka T. 1998. Modifying effects of the Maharishi Amrit Kalash 4 and 5 on the phagocytic and digestive functions of macrophages in male ICR mice. Environ. Heal. Prevent. Med. 3:50–54.
- Trenk H. L., Butz M. E., and Chu F. S. 1971. Production of ochratoxins in different cereal products by Aspergillus ochraceus. Appl. Microbiol. 21:1032–1035.
- ul-Hassan Z., Khan M. Z., Khan A., and Javed I. 2010. Pathological responses of White Leghorn breeder hens kept on Ochratoxin A-contaminated feed. Pak. Vet. J. 30:118–123.
- ul-Hassan Z., Khan M. Z., Khan A., Javed I., and Saleemi M. K. 2011a. Immunological status of progeny of breeder hens kept on Ochratoxin A (OTA)-contaminated feed. J. Immunotoxicol. 8:122–130.
- ul-Hassan Z., Khan M. Z., Saleemi M. K., Khan A., Javed I., and Hussain A. 2011b. Immunological status of White Leghorn chicks hatched from eggs inoculated with Ochratoxin A (OTA). J. Immunotoxicol. DOI: 10.3109/1547691X.2011.568020.
- Wafa E. W., Yahya R. S., Sobh M. A., Eraky I., El-Baz M., El-Gayar H. A. M., Betbeder A. M., and Creppy E. E. 1998. Human ochratoxicosis and nephropathy in Egypt: A preliminary study. Hum. Exp. Toxicol. 17:124–129.
- Wangikar P. B., Sinha N., Dwivedi P., and Sharma A. K. 2007. Teratogenic effects of Ochratoxin A and Aflatoxin B1 alone and in combination on post-implantation rat embryos in culture. J. Turk. Germ. Gyn. Ass. 8:357–364.
- Zimmerli B. and Dick R. 1995. Determination of Ochratoxin A at the ppt level in human blood, serum, milk and some foodstuffs by high-performance liquid chromatography with enhanced fluorescence detection and immunoaffinity column cleanup: Methodology and Swiss data. J. Chromatogr. 666:85–99.
- Zinedine A., Brera C., Elakhdari S., Catano C., Debegnach F., Angelinib S., Santis B. D., Faid M., Benlemlih M., Minardi V., and Miraglia M. 2006. Natural occurrence of mycotoxins in cereals and spices commercialized in Morocco. Food Control 17:868–874.