Abstract
Chronic exposure to organochlorine pesticides (OCP) has been suspected of causing immunoregulatory abnormalities that eventually lead to development and progression of Systemic Lupus Erythematosus (SLE), but the role of these non-genetic stimuli has remained poorly understood. The objectives of the study were to quantify the levels of different OCP residues in the blood of SLE patients and to study the effects of in vitro treatment of peripheral blood mononuclear cells (PBMC) from these patients and healthy controls with OCP. Levels of different OCP residues in the blood were measured by gas-liquid chromatography. Isolated PBMC were treated in vitro with hexachlorocyclohexane (HCH), o,p’-dichlorodiphenyltrichloroethane (DDT), or phytohemagglutinin-M (PHA-M) for 72 h, then stained with different dye-labeled monoclonal antibodies to analyze alterations in T-lymphocytes using flow cytometry. Levels of different TH1 and TH2 cytokines were also estimated by ELISA. Significantly higher levels of p,p’-DDE and β-HCH were detected in the blood of SLE patients than in healthy controls. HCH exposure markedly increased the percentages of CD3+CD4+ T-lymphocytes and expression of CD45RO+ on CD4+ and CD8+ T-lymphocytes, but decreased CD4+CD25+ T-lymphocytes in SLE patients. DDT exposure increased the percentages of CD3+CD4+ T-lymphocytes and decreased those of CD4+CD25+ T-lymphocytes in SLE patients as compared to healthy controls. No significant responsiveness of patient PBMC to PHA-M stimulation was observed indicating suppression of T-lymphocytes by these OCP. Further, both HCH and DDT decreased the levels of IL-2 and IFNγ but had no effect on IL-4 levels in SLE patients. DDT also increased significantly the levels of IL-10 in patients. It is likely that higher levels and prolonged durations of exposure to HCH and DDT may significantly influence T-lymphocyte sub-sets and cytokine expression in vivo that could lead to the development or exacerbation of SLE.
Introduction
Autoimmune diseases are multifactorial in nature and likely involve both environmental and genetic components. Systemic lupus erythematosus (SLE), like many other autoimmune diseases, is a chronic disorder of uncertain cause with a varied clinical course. It is more common among women, and onset is especially likely during childbearing years (Lahita, Citation1996). Beyond the overwhelming evidence that more women are affected by SLE than men, the etiology of this disease is not well understood. Evidence suggests that a combination of factors play a role in the development of SLE, including genetic, environmental, hormonal, and viral influences (Roubinian et al., Citation1978; D’Cruz, Citation2000; Dooley and Hogan, Citation2003). While it is well known that genetic susceptibility plays an important role in the etiology of SLE, previous reports have suggested additional factors may be involved (Deapen et al., Citation1992; Jarvinen et al., Citation1992).
Epidemiologic studies suggest a possible link between pesticides and a variety of autoimmune diseases, but such an association remains speculative. Investigations of exposure to pesticides and estrogenic compounds have been areas of considerable research interest during the last decade. Organochlorine pesticides (OCP; e.g., hexachlorocyclohexane (HCH), dieldrin, o,p’-dichlorodiphenyltrichloroethane (DDT), endosulfan, etc.) differ from other chemical substances because these are purposively spread into the environment. As a consequence, a great part of the human population is exposed. Due to their persistent and lipophilic nature, these halogenated aromatic compounds bioaccumulate in the food chain and may contribute to acute and chronic illnesses. They can stimulate, suppress, or deregulate the immune system, depending on concentration and duration of dose, toxicity/bioreactivity, total body load, and the nutritionat status of the individual. OCP that are known to alter the immune system are DDT, chlordane, aldrin, lindane, hexachlorobenzene, mirex, and arochlor (a polychloro-orobiphenyl) (Glick, Citation1974; Street and Sharma, Citation1975; Giurgea et al., Citation1978; Silkworth and Loose, 1980). Direct T-lymphocyte triggering is found with many OCP. They often cause suppression of T-suppressor (Ts) lymphocytes (Rea et al., Citation1986). Recently, the predominance of anti-nuclear antibody (ANA) in human sera was found to be associated with lifetime exposure to some pesticides, suggesting that occupational exposures to pesticides may be related to the development of SLE (Vine et al., Citation2001; Holsapple, Citation2002). Chemical or other compounds with immunomodulating potential have been suspected of stimulating the initiation and progression of SLE, but the role of these non-genetic stimuli remains poorly understood. Evidence suggests that chronic exposure to pesticides may be playing a role in induction of immunoregulatory abnormalities that eventually lead to SLE.
Despite the (epidemiologic) evidence noted above, a clear understanding of the role of pesticides in influencing SLE development is still in its infancy; scant literature is available to clarify the relationship between dose and effect. Similarly, little is known about the effects of chronic OCP exposure on autoimmune responses. Nonetheless, it is clear that the potential risk to public health, especially in agricultural communities where exposure is widespread, is significant. The amount and timing of exposure to different pesticides may play a significant and complex role in the pathogenesis of autoimmune diseases like SLE. Therefore, a better understanding of the etiopathogenic mechanism of SLE is required in order to clarify the multiple interactions between pesticide exposures and immune cells.
In this study, we investigated and quantified levels of various OCP residues in the blood of SLE patients. In addition, we analyzed, using peripheral blood mononuclear cell (PBMC) samples obtained from SLE patients, different T-lymphocyte sub-sets, i.e., T-helper inducer naive/memory (CD3+CD4+, CD4+CD45RA+/RO+), T-suppressor cytotoxic naive/memory (CD3+CD8+, CD8+CD45RA+/RO+), and activated T-lymphocytes (CD4+CD25+), before and after exposure of these PMBC to different OCP, and also quantified the levels of different TH1 and TH2 cytokines released by these treated cells.
Materials and methods
Patients and samples
Patients attending the Department of Dermatology at University College of Medical Sciences and Guru Teg Bahadur Hospital, Delhi, were enrolled in the study. All the patients (n = 13) fulfilled the American College of Rheumatology classification criteria for SLE. The majority of patients presented with mucocutaneous lesions (70%) and non-erosive polyarthritis (65%). Other clinical manifestations were nephropathy (25%), neuropsychiatric disorder (15%), and anti-phospholipid syndrome (10.5%). The study sample included 76.9% females. All the patients included were either new cases or were not taking any steroids/immunosuppressive drugs/other therapeutic agents for at least 6 months prior to enrolment. Healthy age-matched volunteers (n = 20) were also enrolled for comparisons. The Ethics Committee of UCMS and GTB Hospital (Delhi) approved this study. Written informed consent was obtained from each patient before enrollment in the study.
Peripheral venous blood (≈ 10 ml) was collected aseptically from each patient into EDTA vacutainer tubes and used for pesticide residue quantification and isolation of PBMC. The profile and characteristics of SLE patients and healthy controls studied are listed in .
Table 1. Profile and characteristics of SLE patients and healthy controls studied.
Extraction of pesticides from blood samples and quantification
Blood samples were extracted using HPLC-grade hexane and acetone (2:1 v/v; Merck, Mumbai, India) according to the method of Bush et al. (Citation1984). Briefly, to 1 ml blood in a 50 ml flask, hexane (6 ml) and acetone (3 ml) were added and the contents were shaken at room temp for 30 min in a mechanical shaker. The extract was centrifuged for 10 min at 2000 rpm and the clear hexane layer transferred to a clean test tube. The remaining portion was again extracted twice using the same process and the hexane fractions were added to the previous solvent fractions. Sample clean-up was done via column chromatography following USEPA method 3620B (USEPA, Citation1989). Eluate was collected into a 100 ml beaker and the hexane present evaporated to concentrate the sample. The latter was then re-dissolved in hexane for further analysis.
Quantification of pesticide residue levels was done using a Perkin Elmer Gas Chromatograph equipped with a [63Ni]-selective Electron Capture Detector (Perkin Elmer, Waltham, MA). The column used was Elite-GC DB-5, 60 m × 0.25 mm [ID]. The final extract (1 µl) was injected at a temperature of 170°C with a hold time of 1 min. The temperature was raised from 170°C to 225°C at a rate of 5°C/min (with a hold time of 5 min) and finally from 225°C to 275°C at a rate of 6°C/min (with a hold time of 15 min). The total run length was 40 min/sample. Identification of analytes was confirmed by spiking with known standards. Quantitative analysis of pesticide residues in each sample was done by comparing the peak area with those obtained from a chromatogram of a mixed OCP standard (Sigma Aldrich, St. Louis, MO) of known concentration.
PBMC preparation
Isolation of PBMC was done according to a modification of the method of Boyum (Citation1968). Briefly, the samples were diluted 1:1 with Hank’s Balanced Salt Solution (HBSS), layered over HiSep LSM (Hi-Media Laboratories Pvt. Ltd., Mumbai, India) and centrifuged for 30 min at 500x g at room temperature. The cloudy layer at the interface was carefully aspirated and washed using HBSS. The viability of the cells was measured by a trypan blue exclusion assay; values were consistently greater than 95%. PBMC obtained were re-suspended at a final concentration of 1 × 106 cells/ml in RPMI 1640 media supplemented with 10% fetal bovine serum, 100 IU penicillin/ml, 100 µg streptomycin/ml, and 2 mM L-glutamine (all from Hi-Media Laboratories).
Stimulation of PBMC
PBMC (1 × 106 cells/ml) in RPMI 1640 were seeded into wells of a 12-well culture plate (Nalgene Nunc, Rochester, NY) and treated with vehicle, β-HCH (100 nM), or DDT (50 µM) (each OCP in dimethyl sulfoxide [DMSO]) and incubated at 37°C in humidified air containing 5% CO2 for 72 h. In all cases, DMSO final concentration was 0.01%. All tests were done in triplicate. After 72 h, the plates were centrifuged, the medium in each well was removed, and cells were washed with RPMI 1640. Fresh medium was added and the cells were (re-)stimulated with 10 µg phytohemagglutinin-M (PHA-M)/ml (Sigma) for 24 h. In separate wells, fresh PBMC (1 × 106 cells/ml) were also stimulated with 10 µg/ml PHA-M for 72 h for comparisons. For this study, we chose to use the PHA-M stimulation based on: (1) preliminary experiments that demonstrated reproducible decreases in SLE patient T-lymphocyte (particularly CD3+CD4+ and CD4+CD45RO+) proliferative responses and IL-2 production after PHA-M stimulation (outcomes consistent with published observations; Alcocer-Varela and Alarcon-Segovia, Citation1982; Linker-Israeli et al., Citation1983); and (2) controversial data that have been reported regarding responses of SLE T-lymphocytes to anti-CD3 monoclonal antibodies (see Stekman et al., Citation1991; Blasini et al., Citation1993).
The concentrations of the different OCP and the PHA stimulant were selected on the basis of some preliminary (data not shown) experiments and the literature. These values for the OCP were purposely selected to be well below the previously reported values that would induce overt cytotoxicity to cultured human PBMC/lymphocytes. Specifically, with regard to β-HCH, Huber et al. (Citation1990) reported an EC50 [cytotoxicity] of 100 µM and Roux et al. (Citation1979) showed that even a dose of 200 µM β-HCH (for 72 h) had no effect on the viability of cultured human lymphocytes. For DDT, a study by Yanez et al. (Citation2004) using PBMC cultured with 40–100 µg DDT/ml (113–282 µM) for 72 h indicated that with the highest dose there was only a 3% drop in viability of the cells from that of untreated controls. Nevertheless, all cells were checked microscopically to confirm that the doses of the OCP used were in fact not cytotoxic to the seeded cells.
Staining of PBMC and fluorescence-activated cell sorting (FACS)
Upon completion of the incubation, the PBMC were harvested by centrifugation at 500 x g for 5 min and the cell-free culture supernatants were removed and frozen at −80°C for use in cytokine analyses later. Harvested PBMC were washed three times with wash buffer (0.5% BSA + 0.1% NaN3 in phosphate-buffered saline [PBS, pH 7.4]) before staining with combinations of conjugated monoclonal antibodies (MAb). Each MAb, i.e., anti-CD3, -CD4, -CD8, -CD45RA, -CD45RO, and -CD25, was conjugated to peridine chlorophyll (PerCP), fluorescein isothio-cynate (FITC), phycoerythrin (PE), or allophycocyanin (APC). All the antibodies including corresponding isotype controls were purchased from BD Biosciences (Gurgaon, India).
Staining was done on ice for 30–60 min in the dark after which cells were washed three-times with wash buffer and fixed with 200 µl ice-cold 2% paraformaldehyde (in PBS). FACS scanning was performed within 18 h using a FACS-Calibur system (BD Biosciences). A minimum of 20,000–30,000 events was collected per condition. All data were analyzed using WinMDI 2.9 software (http://www.mybiosoftware.com/cytometry). Appropriate isotype control antibodies were included in all analyses.
Cytokine quantification ELISAs
Cytokine quantification kits were obtained from BD Biosciences. Levels of interleukin (IL)-2, IL-4, IL-10, and interferon (IFN)-γ were determined in culture supernatants, according to manufacturer’s instructions. The lower level of detection of all the kits was ≥4 pg/ml.
Statistical analyses
Data is presented as the mean (± SD). Quantitative pesticide residue variables between patients and healthy controls were tested using unpaired Student’s t-test. The variations between proportions of T-lymphocyte sub-populations—as well as of cytokine levels—were analyzed by a two-way analysis of variance (ANOVA) followed by a Tukey’s test. p-values of <0.05 were taken to denote significance.
Results
Levels of organochlorine pesticides in the peripheral blood of SLE patients
Concentrations of various OCP residues in the peripheral blood samples of SLE patients and healthy controls are summarized in . Among the OCPs investigated in this study, significantly higher levels of p,p’-DDE (the metabolite of DDT) and β-HCH (an isoform of HCH) were detected in patients than in controls. Although higher levels of α-HCH and α-endosulfan were also observed in patients than controls, the differences were not statistically significant.
Table 2. OCP levels (ng/ml) in peripheral blood of SLE patients and healthy controls (HC).
Effects of in vitro exposure to HCH and DDT on T-lymphocyte sub-sets in SLE patients
To determine the effect of total HCH (technical grade) and DDT exposure on T-lymphocytes sub-population profiles, harvested PBMC were treated in vitro with vehicle, HCH, or DDT for 72 h, washed, and then (re-)stimulated with PHA-M (10 µg/ml) for 24 h. In unstimulated PBMC, CD4+ T-lymphocyte levels were significantly reduced in patients as compared to in the healthy controls (). However, a significant increase in the percentage (relative to baseline level among unstimulated counterpart cells) of CD4+ T-lymphocytes was seen among the PBMC of the SLE patients upon exposure to 100 mM HCH or 50 µM DDT (with greatest increase occurring with HCH). Treatment with either OCP had no similar significant effect on the cells from the healthy controls. Furthermore, HCH treatment of the SLE PBMC resulted in significantly higher levels of CD4+ T-lymphocytes relative to levels among similarly treated PBMC from the healthy controls. When the PBMC were treated with PHA-M (at 10 µg/ml) alone, no significant differences were observed in CD4+ T-lymphocytes of patients; however, significant differences were observed in the case of healthy controls relative to the proportions in unstimulated cells.
Figure 1. Changes in CD3+CD4+ and CD3+CD8+ T-lymphocyte sub-sets in patients with SLE and healthy controls (HC) upon exposure to HCH (100 mM), DDT (50 µM), and/or PHA-M (10 µg/ml). Mean percentages (± SD) of (a) CD3+CD4+ and (b) CD3+CD8+ T-lymphocytes. *p < 0.05 vs HC; #p < 0.05 vs unstimulated cells.
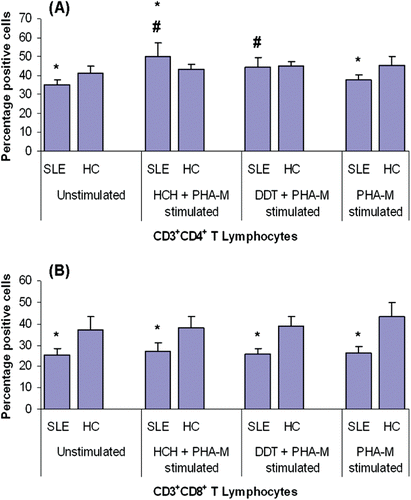
Similarly, CD8+ T-lymphocytes were found in significantly lower levels in patients as compared to in controls when unstimulated. However, in contrast with their CD4+ counterparts, there were no significant increases in levels among the PBMC from either the patients (or for that fact, the healthy controls) upon exposure to either OCP (). On the other hand, when PBMC were stimulated with PHA-M only, significantly higher percentages of CD8+ T-lymphocytes were observed in the case of the healthy controls, but not with the cells from SLE patients.
Effects of in vitro exposure to HCH and DDT on expression of naïve (CD45RA) and memory (CD45RO) markers on CD4+ and CD8+ T-lymphocytes of SLE patients
Potential changes in expression of CD45RA and CD45RO on CD4+ and CD8+ T-lymphocytes were also analyzed. The difference in levels of CD45RA+CD4+ T-lymphocytes among the population of unstimulated PBMC from SLE patients and healthy controls was not significant (); however, there were significantly increased levels of CD45RA+CD8+ T-lymphocytes among the SLE PBMC (). Treatment of harvested PBMC with 100 mM HCH for 72 h resulted in significantly decreased (relative to baseline levels among unstimulated counter-part cells) levels of CD45RA+CD4+ and CD45RA+CD8+ T-lymphocytes in the SLE PBMC system. DDT (50 µM) treatment, on the other hand, caused a similar effect only on the CD8+ T-lymphocytes. Again, treatment with either OCP had no similar (significant) effect on PBMC from the healthy control subjects. Stimulation with PHA-M alone caused significantly increased CD45RA+ expression on CD4+ T-lymphocytes in the PBMC obtained from both patients and healthy controls; in contrast, PHA-M treatment only affected this expression on CD8+ T-lymphocytes in the PBMC from the healthy controls.
Figure 2. CD45RA expression on CD4+ and CD8+ T-lymphocytes from patients with SLE and healthy controls (HC) after treatment with HCH, DDT, and/or PHA-M. CD45RA expression on (a) CD4+ and (b) CD8+ T-lymphocytes. Values presented as mean percentages (± SD). *p < 0.05 vs HC; #p < 0.05 vs unstimulated cells.
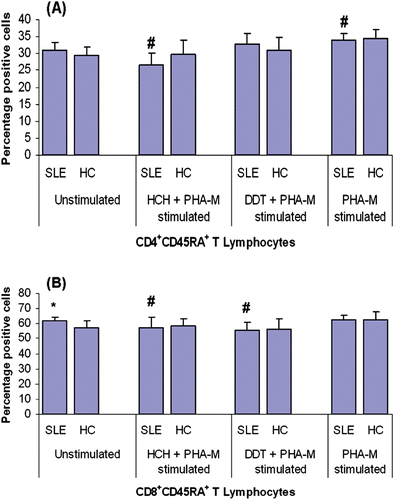
There was no statistically significant difference in the levels of CD45RO+CD4+ cells among the PBMC from either population (), as was the case with CD45RA+CD4+ cells. However, in contrast with the above-noted CD45RA results, the SLE PBMC contained significantly lower levels (compared to among healthy control PBMC) of CD45RO+CD8+ T-lymphocytes (). HCH exposure significantly increased the expression of both CD45RO+CD4+ and CD45RO+CD8+ lymphocytes in the SLE PBMC system (relative to baseline levels among unstimulated counterpart cells), but appeared to have no significant effect on its expression among healthy control PBMC. DDT treatment caused no significant change in the levels of CD45RO+CD4+ and CD45RO+CD8+ lymphocytes among the PBMC from either population. Notably, PHA-M stimulation alone resulted in significantly increased expression of CD45RO+ on both CD4+ and CD8+ T-lymphocytes among the PMBC from the healthy controls, but seemed to have no effect on the cells from the SLE patients.
Figure 3. CD45RO expression on CD4+ and CD8+ T-lymphocytes from patients with SLE and healthy controls (HC) after exposure to HCH, DDT, and/or PHA-M. CD45RO expression on (a) CD4+ and (b) CD8+ T-lymphocytes. Values are presented as mean percentages (± SD). *p < 0.05 vs HC; #p < 0.05 in comparison to unstimulated cells.
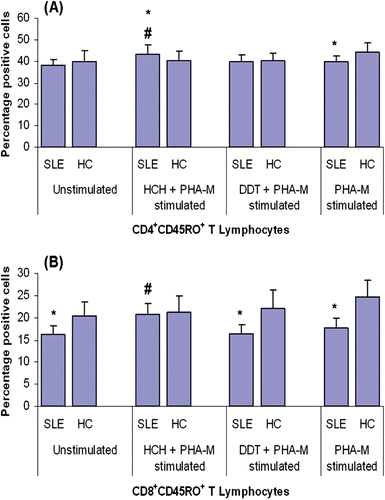
Effects of in vitro exposure to HCH and DDT on expression of CD25 on CD4+ T-lymphocytes of SLE patients
Analysis of the levels of CD25+CD4+ T-lymphocytes among the unstimulated PMBC samples revealed that levels of these cells were significantly reduced in samples from the SLE patients as compared to those among the healthy control PBMC (, ). In examining the effect from HCH and DDT exposures (each in the co-presence of PHA-M) on expression of these cells, an even further decrease in percentages was noted among the SLE PBMC. In contrast, treatment of control PBMC led to nominal (albeit insignificant) increases in levels of these cells. Interestingly, PHA-M stimulation alone marginally increased the percentages of CD25+CD4+ T-lymphocytes in PMBC from the SLE patients, but significantly so among the cells from the healthy controls.
Table 3. Comparison of the relative levels of CD4+CD25+ T-lymphocytes between SLE patients and healthy controls (HC) when unstimulated or treated with HCH and PHA-M, DDT and PHA-M, or with PHA-M alone.
Effect of in vitro exposures to HCH on the secretion of IL-2, IL-4, IL-10, and IFN-γ from human lymphocytes
Levels of cytokines produced/released by the HCH-treated SLE PBMC were compared against those from unstimulated SLE PBMC and the cells from healthy control subjects. Unstimulated cells from SLE patients produced significantly lower levels of IL-2 than cells from healthy controls (). When PBMC from these groups were exposed to 100 mM HCH for 72 h, a decrease in secretion of IL-2 was noted with both populations’ cells; however, the decrease was significant only with the healthy control PBMC. There were no significant differences/induced changes in IL-4 levels among any of the cell groups examined. Unstimulated SLE PBMC secreted significantly higher levels of IL-10 compared to that by the healthy control PBMC. Interestingly, while exposure to HCH caused no significant change in IL-10 secretion levels among the SLE PBMC, it did cause significant reduction among the control cells. Baseline levels of IFNγ release were significantly lower among the SLE PBMC compared to that by their healthy control counterparts. HCH treatment caused these levels to be decreased even further; a similarly significant reduction in IFNγ levels was also observed with the HCH-treated healthy control PBMC.
Figure 5. Cytokine levels (pg/ml) produced by PBMC from SLE patients (n = 13) and healthy controls (HC) (n = 20) in unstimulated, HCH-treated, DDT-treated, and/or PHA-M-stimulated culture conditions. Mean percentages (± SD) of (a) IL-2, (b) IL-4, (c) IL-10, and (d) IFNγ. *Difference is significant at p < 0.05.
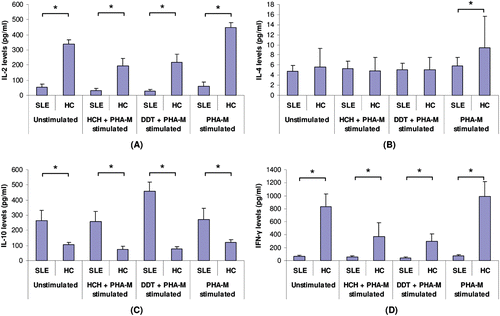
Effect of in vitro exposures to DDT on the secretion of IL-2, IL-4, IL-10, and IFNγ from human lymphocytes
When PBMC were exposed to 50 µM DDT for 72 h, IL-2 secretion was significantly decreased in cells recovered from both SLE patients and healthy controls (relative to baseline level among unstimulated counterpart cells). DDT exposure caused no significant alterations in secretion of IL-4 in both groups. In contrast, significantly increased secretion of IL-10 was observed in SLE PBMC on exposure to DDT. These levels were twice those released by unstimulated SLE PBMC and up to 6-times higher compared to DDT-treated healthy control cells (). There was no significant difference in IFNγ secretion by patient PBMC upon DDT exposure; however, a significant decrease was observed with the cells from the healthy controls. IFNγ secretion by unstimulated PBMC of healthy controls was up to 12-times greater than that of the patient cells.
SLE PBMC exposed only to mitogen PHAM at 10 µg/ml showed no significant increase (compared to unstimulated counterpart cells) in any of the cytokines quantified; however, significantly higher levels of IL-2, IL-4, and IL-10 were released into the culture medium by healthy control PBMC as compared to by their unstimulated counterpart cells. The levels of IFNγ were also increased in healthy control PBMC, but the difference was not statistically significant ().
Discussion
Organochlorine pesticides (OCP) pervade the ecosystem due to wide usage. In animal models, OCP exposure accelerated SLE progression (Sobel et al., Citation2005). Occupational exposure to pesticides is also attendant with a risk of developing SLE (Cooper et al., Citation2004). The estrogen-like effects of OCP (Andersen et al., Citation2002) and the influence of estrogen on murine models of SLE (Roubinian et al., Citation1978) permit one to conclude that OCP may influence development of SLE. To better understand how OCP might exert this influence, we sought to determine if improper triggering of cell activation mechanisms could account, in part, for altered T-lymphocyte proliferation and cytokine production in SLE. To achieve this aim, we examined quantitative and functional interactions between OCP (i.e., HCH and DDT) and T-lymphocytes of genetically-/immunologically-defective and persistently pesticide-exposed SLE subjects.
In our study, levels of p,p’-DDE (DDT metabolite) and HCH (β-isoform) were higher in SLE patients than in healthy controls; α-endosulfan levels were also higher, but comparable to control values. The higher DDT and HCH levels in the SLE subjects compared to in control subjects from the same locale could be attributable to genetic defects in xenobiotic metabolizing enzymes such as CYP1A1, GSTM1 and NAT-2 (von Schmiedeberg et al., Citation1999; Horiuchi et al., Citation2009). Such defects would allow the pesticides to accumulate in the body. Although the results here clearly indicate higher blood levels of the two OCP of interest, our analytical approaches did not allow us to distinguish between burdens that might have been associated with individual cell (RBC vs WBC) types or even with other blood constituents (proteins, etc.).
Animal studies have illustrated effects of OCP on humoral and cell-mediated immune responses (Barnett and Rodgers, Citation1994; Banerjee et al., Citation1996). As thymic atrophy was a consistent finding (Dencker et al., Citation1985; Fine et al., Citation1990; Holladay et al., Citation1991) it would seem that components critical to cell-mediated immunity are sensitive targets for certain OCP. Here, ex vivo treatment of PBMC with DDT of HCH exposure affected CD4+ T-lymphocyte levels to the greatest extent with cells from the SLE subjects, as compared to that seen with vehicle-treated SLE PBMC and pesticide-treated control PBMC. These results were in keeping with the findings of Raziuddin et al. (Citation1990), who reported that changes in CD4+ T-lymphocyte levels correlate with SLE (disease) activity, for reasons still not yet defined. Furthermore, deficient Ts-lymphocyte activity is also common in SLE (Kammer and Stein, Citation1990). The present study confirmed that CD8+ lymphocyte levels were low in the PBMC of SLE patients. Interestingly, in contrast to the CD4+ cells, no great effects on CD8+ T-lymphocyte levels were noted with OCP exposure.
T-lymphocytes from SLE patients display a memory phenotype, with relatively low numbers of naive and Ts-lymphocytes (Fritsch et al., Citation2006). We, however, observed significantly increased expression of CD45RA+CD8+ lymphocytes in the PBMC from SLE subjects. This ‘heightened’ expression was significantly reduced by ex vivo DDT or HCH exposure. HCH treatment also caused significant decreases in CD45RA+CD4+ lymphocyte levels from baseline. Conversely, the HCH led to increased CD45RO+CD4+ and CD45RO+CD8+ cell expression in these patients’ PBMC. There are reports of a concomitant expression of both CD45RA and CD45RO associated with effector T-lymphocyte phenotypes, but the relationship of this population to naïve/memory cells is not understood (Swain et al., Citation1996; Farber, Citation1998). These concomitant expressions may have contributed to a skewing of the sums of CD45RA+/RO+ CD4+ and CD45RA+/RO+ CD8+ lymphocyte values here. Nevertheless, the outcomes here suggest that OCP like HCH and DDT (or their metabolites)—as seen with other OCP (Colosio et al., Citation2005; Esquivel-Sentíes et al., Citation2010)—may alter the function of certain elements of the immune response, thereby enhancing the risk for immunomodulated outcomes, including autoimmunity.
PHA-M stimulation (without prior treatment with OCP) here led to a reduced proliferation by SLE patient CD4+ and CD8+ T-lymphocytes. This might be a result of higher levels of p,p’-DDE and both α- and β-HCH in their blood. Sub-optimal lymphoproliferative activity (i.e., decreased responses to PHA, ConA, PWM) and modest changes in total white blood cell and lymphocyte levels have been noted in populations exposed to DDT/other OCP (McConnachie and Zahalsky, Citation1992; Backer, Citation1995; Vine et al., Citation2001). Our study also showed that PHA-M stimulation caused a significant increase in CD45RA+CD4+ lymphocyte levels among the PBMC from both SLE patients and healthy controls. However, increased expression of CD45RA+CD8+ lymphocytes was observed only with healthy control PBMC. This suggested a reduction in naïve suppressor (CD8+CD45RA+) lymphocyte levels in SLE. CD45RO+CD4+ and CD45RO+CD8+ cell levels among the PMBC from SLE patients did not significantly change after PHA-M stimulation; however, they were significantly increased in PMBC from the controls. Overall, the observations here indicated that SLE patient T-lymphocytes were less responsive to PHA-M stimulation—potentially as a result of the higher HCH and DDT levels in their blood.
The results of the studies of the effects of the OCP ± PHA-M on CD25+CD4+ lymphocyte expression are in concordance with an earlier study showing reduced T-regulatory (Treg) cell levels in SLE patients (Mudd et al., Citation2006). When left unstimulated, the percentages of this cell type were significantly lower in the patients (by ≈ 35% relative to control values). Treatment of SLE PBMC with HCH or DDT (in the presence of PHA-M) caused a modest further reduction in these cells’ percentages (relative to unstimulated SLE values); in contrast, treatment of control PBMC led to nominal increases (albeit insignificant) in these cells. As repeated OCP exposure is associated with decreased response to mitogen (McConnachie and Zahalsky, Citation1992; Backer, Citation1995; Vine et al., Citation2001), it is possible that the ex vivo exposure of SLE patients’ T-lymphocytes to HCH or DDT may have simply resulted in a ‘piling on’ effect, i.e., the additional ‘new’ OCP may have only nominally deterred proliferation/cell functional activity beyond any already-reduced baseline activity. Clearly, the SLE cells are capable of responding to PHA-M, but significantly less-so than control cells; treatment with PHA-M alone led to increases in CD25+CD4+ levels of 15% vs 35%, respectively. The pesticide-induced drop-off relative to values with the PHA-M alone lead us to conclude that the exogenous HCH or DDT may have imparted an additional burden on the cells’ ability to interact with/respond to this mitogen and/or triggered a distinct effect that led to decrements in cell numbers (even in any absence of a new effect on mitogen responsivity). We suggest the latter in light of an earlier study wherein DDT-induced apoptosis was noted (Pérez-Maldonado et al., 2004).
IL-2 has been implicated in the ability of Treg cells to control T-lymphocyte expansion (Malek and Bayer, Citation2004; Scheffold et al., Citation2005). The Treg are thought to control T-lymphocyte expansion by secreting IL-10 and inducing an IL-2 negative feedback mechanism (Lee et al., Citation1999; Yu et al., Citation2003). Decreased IL-2 production results in a reduced activation-induced cell death and a persistence of autoreactive and memory type T-lymphocytes. Our results agree with studies reporting reductions in IL-2 levels in the sera of SLE patients (Tenbrock and Tsokos, Citation2004). We observed that unstimulated cells from the SLE patients produced significantly lower levels of IL-2 and IFNγ (TH1 cytokines) than did PBMC from healthy controls. Treatment with HCH or DDT resulted in further reductions in levels of these cytokines by PBMC from both populations. This suggests a possible role for OCP in causing TH1 cytokine reduction (that, in turn, impacts on Treg function) in SLE patients. In contrast, there were no significant differences between patients and healthy control PBMC IL-4 secretion patterns, and neither HCH nor DDT altered levels significantly. In vivo constitutive expression of IL-4 has been reported to induce autoimmune-type disorders in mice (Erb et al., Citation1997); on the other hand, Santiago et al. (Citation1997) suggested that IL-4 might protect against a genetically-linked lupus-like autoimmune syndrome.
SLE is also characterized by abnormally high IL-10 production (Hagiwara et al., Citation1996). Our results show that unstimulated patient PBMC secreted significantly higher IL-10 levels than healthy control cells. This is in agreement with data obtained from untreated SLE patients whose PBMC exhibited increased spontaneous synthesis of IL-10 (Llorente et al., Citation1993). High IL-10 secretion by monocytes/lymphocytes is responsible for heightened immunoglobulin production (Llorente et al., Citation1995). In our study, ex vivo exposure to HCH caused a small decrease in IL-10 levels in SLE PBMC, but resulted in significant decreases in healthy control cells. However, DDT exposure caused a 2-fold increase in secretion of IL-10 in patient cells. This heightened expression in the case of DDT may reflect an ongoing negative loop, as IL-10 is known to inhibit the production of IFNγ by TH1 lymphocytes (Moore et al., Citation1990, Citation1993; Hagiwara et al., Citation1996). IL-10 also down-regulates the development of cell-mediated responses (Gazzinelli et al, Citation1992; Ding et al., Citation1993), but stimulates B-lymphocytes (Rousset et al, Citation1992). This latter effect likely plays a major role in the polyclonal B-lymphocyte hyperactivity associated with development of diseases like SLE.
Conclusions
In conclusion, OCP—specifically, HCH and DDT—were found in high levels in blood of SLE patients. It is likely that higher levels and prolonged exposure to these pesticides may have significantly influenced T-lymphocyte sub-sets and cytokine expression in vivo. The high IL-10 expression in these patients was independent of disease severity and could affect production of IL-2 or IFNγ in these hosts. Furthermore, incessant low dose exposure to these OCP may functionally handicap the patients’ cells to respond to extraneous cytokines, resulting in induction of a form of cell exhaustion or anergy. Although the clinical significance of these findings is uncertain, the described changes in lymphocyte sub-sets and cytokine formation by these cells could be critical in the development/exacerbation of SLE or other autoimmune diseases.
Addendum
Adaptive Treg cells mature in peripheral sites from CD4+ precursors where they acquire the expression of markers, including CD25 (along with CTLA4 and GITR/AITR). Only upon up-regulation of the transcription factor Foxp3 do Treg cells begin to express any suppressive effect. In the study here, only expression of CD25+ on CD4+ T-lymphocytes—and not FoxP3—was measured. We know we cannot make an outright direct linkage between changes in CD4+CD25+ levels to those in ‘active’ Treg cells; CD25 is also expressed on non-regulatory T-lymphocytes (especially in a setting of immune activation such as during a response to pathogens or during chronic inflammatory states, etc.) (Isomaki et al., Citation2005) or activated T-lymphocytes (Baecher-Allan et al., Citation2001). Any analysis of CD4+CD25+ alone under those conditions might not be able to reflect if there were concurrent decrements in CD4+CD25+FoxP3+ cell levels (i.e., these would be masked).
However, as: (1) CD25 and FoxP3 expression are concurrently induced upon lymphocyte activation with various stimuli (Allan et al., Citation2007; Pillai et al., Citation2007; Wang et al., Citation2007); (2) human CD4+ lymphocytes with the highest CD25 expression levels are regarded to represent Treg (in contrast to CD4+ cells with intermediate CD25 expression); and (3) active functioning Treg cells are obligated to be CD4+CD25+FoxP3+ (Sakaguchi, Citation2005; Campbell and Ziegler, Citation2007), all active functional Treg cells must express CD25+. Thus, unless a host is in a pathologic state that engenders extreme shifts in non-regulatory T-lymphocyte and/or changes in adaptive Treg cell levels, then changes in the levels/percentages of CD4+CD25+ cells should reflect, in great part, concurrent changes in active functioning immune response suppressing CD4+CD25+FoxP3+ Treg cell levels.
Acknowledgments
The authors thank Mr Kavindra Kumar and Mr Arun for their assistance in the laboratory work. This work was financially supported by the Department of Science and Technology, Govt. of India (File no. SR/SO/HS-82/2005). Indian Council of Medical Research (ICMR), New Delhi is acknowledged for providing Senior Research fellowship (Ref. 45/3/2011/Imm/BMS) to SAD.
Declaration of interest
The authors report no conflicts of interest. The authors alone are responsible for the content and writing of the paper.
References
- Alcocer-Varela, J., and Alarcon-Segovia, D. 1982. Decreased production of and response to IL-2 by cultured lymphocytes from patients with Systemic Lupus Erythematosus. J. Clin. Invest. 69:1388–1392.
- Allan, S. E., Crome, S. Q., Crellin, N. K., Passerini, L., Steiner, T. S., Bacchetta, R., Roncarolo, M. G., and Levings, M. K. 2007. Activation-induced FoxP3 in human T-effector cells does not suppress proliferation or cytokine production. Int. Immunol. 19:345–354.
- Andersen, H. R., Vinggaard, A. M., Rasmussen, T. H., Gjermandsen, I. M., and Bonefeld-Jorgensen, E. C. 2002. Effects of currently used pesticides in assays for estrogenicity, androgenicity, and aromatase activity in vitro. Toxicol. Appl. Pharmacol. 179:1–12.
- Backer, L. C. 1995. Pet dogs as sentinels for human exposure to environmental pollution. In: Biomonitors and Biomarkers as Indicators of Environmental Change (Butterworth, F. M., Corkum, L. D., and Guzmán-Rincon, J., Eds.). New York: Plenum Press, pp. 193–206.
- Baecher-Allan, C., Brown, J. A., Freeman, G. J., and Hafler, D. A. 2001. CD4+CD25high regulatory cells in human peripheral blood. J. Immunol. 167:1245–1253.
- Banerjee, B. D., Koner, B. C., and Ray, A. 1996. Immunotoxicity of pesticides: Perspectives and trends. Indian J. Exp. Biol. 34:723–733.
- Barnett, J. B., and Rodgers, H. E. 1994. Pesticides. In: Immunotoxicology and Immunopharmacology, 2nd Edition (Dean, J. H., Luster, M. I., Munson, A. E., and Kimber, I., Eds.). New York: Raven Press, pp. 191–212.
- Blasini, A. M., Stekman, I. L., Leon-Ponte, M., Caldera, D., and Rodriguez, M. A. 1993. Increased proportion of responders to a murine anti-CD3 monoclonal antibody of the IgG1 class in patients with systemic lupus erythematosus (SLE). Clin. Exp. Immunol. 94:423–428.
- Boyum, A. 1968. Isolation of mononuclear cells and granulocytes from human blood. Isolation of monuclear cells by one centrifugation, and of granulocytes by combining centrifugation and sedimentation at 1 g. Scand. J. Clin. Lab. Invest. Suppl. 97:77–89.
- Bush, B., Snow, J., and Koblintz, R. 1984. Polychlorobiphenyl (PCB) congeners, p,p’-DDE and hexachlorobenzene in maternal and fetal cord blood from mothers in upstate New York. Arch. Environ. Contam. Toxicol. 13:517–527.
- Campbell, D. J., and Ziegler, S. F. 2007. FoxP3 modifies the phenotypic and functional properties of regulatory T-cells. Nat. Rev. Immunol. 7:305–310.
- Colosio, C., Birindelli, S., Corsini, E., Galli, C. L., and Maroni, M. 2005. Low level exposure to chemicals and immune system. Toxicol. Appl. Pharmacol. 207:320–328.
- Cooper, G. S., Parks, C. G., Treadwell, E. L., St., Clair, E. W., Gilkeson, G. S., and Dooley, M. A. 2004. Occupational risk factors for the development of systemic lupus erythematosus. J. Rheumatol. 31:1928–1933.
- D’Cruz, D. 2000. Autoimmune diseases associated with drugs, chemicals, and environmental factors. Toxicol. Lett. 112:421–432.
- Deapen, D., Escalante, A., Weinrib, L., Horwitz, D., Bachman, B., Roy-Burman, P., Walker, A., and Mack, T. M. 1992. A revised estimate of twin concordance in systemic lupus erythematosus. Arthritis Rheum. 35:311–318.
- Dencker, L., Hassoun, E., d’Argy, R., and Alm, G. 1985. Fetal thymus organ culture as an in vitro model for the toxicity of 2,3,7,8-tetrachlorodibenzo-p-dioxin and its congeners. Mol. Pharmacol. 27:133–140.
- Ding, L., Linsley, P. S., Huang, L. Y., Germain, R. N., and Shevach, E. M. 1993. IL-10 inhibits macrophage co-stimulatory activity by selectively inhibiting the up-regulation of B7 expression. J. Immunol. 151:1224–1234.
- Dooley, M. A., and Hogan, S. L. 2003. Environmental epidemiology and risk factors for autoimmune disease. Curr. Opin. Rheumatol. 15:99–103.
- Erb, K. J., Ruger, B., von Brevern, M., Ryffel, B., Schimpl, A., and Rivett, K. 1997. Constitutive expression of interleukin (IL)-4 in vivo causes autoimmune- type disorders in mice. J. Exp. Med. 185:329–339.
- Esquivel-Sentíes, M. S., Barrera, I., Ortega, A., and Vega, L. 2010. Organophosphorous pesticide metabolite (DEDTP) induces changes in the activation status of human lymphocytes by modulating IL-2 receptor signal transduction pathway. Toxicol. Appl. Pharmacol. 248:122–133.
- Farber, D. L. 1998. Cutting-edge commentary: Differential TCR signaling and the generation of memory T-cells. J. Immunol. 160:535–539.
- Fine, J. S., Gasiewicz, T. A., Fiore, N. C., and Silverstone, A. E. 1990. Pro-thymocyte activity is reduced by perinatal 2,3,7,8-tetrachlorodibenzo-p-dioxin exposure. J. Pharmacol. Exp. Ther. 255:128–132.
- Fritsch, R. D., Shen, X., Illei, G. G., Yarboro, C. H., Prussin, C., Hathcock, K. S., Hodes, R. J., and Lipsky, P. E. 2006. Abnormal differentiation of memory T-cells in systemic lupus erythematosus. Arthritis Rheum. 54:2184–2197.
- Gazzinelli, R. T., Oswald, I. P., James, S. L., and Sher, A. 1992. IL-10 inhibits parasite killing and nitrogen oxide production by IFNγ-activated macrophages. J. Immunol. 148:1792–1796.
- Giurgea, R., Witterberger, C., Frecus, G., Manciulea, S., Borsa, M., Coprean, D., and Ilyes, S. 1978. Effects of some organochlorine pesticides on the immunological reactivity of white rats. Archiv. fur Exp. Vet. 32:769–774.
- Glick, B. 1974. Antibody-mediated immunity in the presence of mirex and DDT. Poultry Sci. 53:1476–1485.
- Hagiwara, E., Gourley, M. F., Lee, S., and Klinman, D. K. 1996. Disease severity in patients with SLE correlates with an increased ratio of IL-10: IFNγ-secreting cells in the peripheral blood. Arthritis Rheum. 39:379–385.
- Holladay, S. D., Lindstorm, P., Blaylock, B. L., Comment, C. E., Germolec, D. R., Heindell, J. J., and Luster, M. I. 1991. Perinatal thymocyte antigen expression and postnatal immune development altered by gestational exposure to tetrachlorodibenzo-p-dioxin (TCDD). Teratology 44:385–393.
- Holsapple, M. P. 2002. Autoimmunity by pesticides: A critical review of the state of the science. Toxicol. Lett. 127:101–109.
- Horiuchi, T., Washio, M., Kiyohara, C., Tsukamoto, H., Tada, Y., Asami, T., Ide, S., Kobashi, G., Takahashi, H., and the Kyushu Sapporo SLE Study Group. 2009. Combination of TNF-RII, CYP1A1 and GSTM1 polymorphisms and the risk of Japanese SLE: Findings from the KYSS study. Rheumatology 48:1045–1049.
- Huber, H. C., Huber, W., and Ritter, U. 1990. Simple in vitro test systems for determining the toxicity of environmental chemicals: Micro-cultures of human lymphocytes and monoxenic ciliate cultures. Zentral. Hyg. Umweltmed. 189:511–526.
- Isomaki, P., Clark, J. M., Panesar, M., and Cope, A. P. 2005. Pathways of T-cell activation and terminal differentiation in chronic inflammation. Curr. Drug Targets Inflamm. Allergy 4:287–293.
- Jarvinen, P., Kaprio, J., Makitalo, R., Koskenvuo, M., and Aho, K. 1992. Systemic lupus erythematosus and related systemic diseases in a nationwide twin cohort: An increased prevalence of disease in MZ twins and concordance of disease features. J. Intern. Med. 231:67–72.
- Kammer, G. M., and Stein, R. L. 1990. T-Lymphocyte immune dysfunction in systemic lupus erythematosus. J. Lab. Clin. Med. 115:273–282.
- Lahita, R. G. 1996. The connective tissue diseases and the overall influence of gender. Int. J. Fertil. Menopausal Stud. 41:156–165.
- Lee, I. H., Li, W. P., Hisert, K. B., and Ivashkiv, L. B. 1999. Inhibition of IL-2 signaling and signal transducer and activator of transcription (STAT)5 activation during T-cell receptor-mediated feedback inhibition of T-cell expansion. J. Exp. Med. 190:1263–1274.
- Linker-Israeli, M., Bakke, A. C., Kifridou, R. C., Gendler, K. S., Gillis, S., and Horwitz, D. A. 1983. Defective production of IL-1 and IL-2 in patients with systemic lupus erythematosus. J. Immunol. 130:2651–2655.
- Llorente, L., Richaud-Patin, Y., Wijdenes, J., Alcocer-Varela, J., Maillot, M. C., Durand-Gasselin, I., Fourrier, B. M., Galanaud, P., and Emilie, D. 1993. Spontaneous production of IL-10 by B-lymphocytes and monocytes in systemic lupus erythematosus. Eur. Cytokine Netw. 4:421–427.
- Llorente, L., Zou, W., Levy, Y., Richaud-Patin Y., Wijdenes, J., Alcocer-Varela, J., Morel-Fourrier, B., Brouet, J. C., Alarcon-Segovia, D., Galanaud, P., and Emilie, D. 1995. Role of interleukin-10 in the B-lymphocyte hyperactivity and autoantibody production of human systemic lupus erythematosus. J. Exp. Med. 181:839–844.
- Malek, T. R., and Bayer, A. L. 2004. Tolerance, not immunity, crucially depends on IL-2. Nat. Rev. Immunol. 4:665–674.
- McConnachie, P. R., and Zahalsky, A. C. 1992. Immune alterations in humans exposed to the termiticide technical chlordane. Arch. Environ. Health 47:295–301.
- Moore, K. W., O’Garra, A., de Waal Malefyt, R., Vieira, P., and Mossman, T. R. 1993. Interleukin-10. Annu. Rev. Immunol. 11:165–190.
- Moore, K. W., Vieira P., Fiorentino, D. F., Trounstine M. L., Khan, T. A., and Mossman, T. R. 1990. Homology of cytokine synthesis inhibitory factor (IL-10) to the Epstein–Barr virus gene BCRFI. Science 248:1230–1234.
- Mudd, P. A., Teague B. N., and Farris, A. D. 2006. Regulatory T-cells and systemic lupus erythematosus. Scand. J. Immunol. 64:211–218.
- Perez-Maldonado, I. N., Diaz-Barriga, F., de la Fuente H., Gonzalez-Amaro, R., Calderon J., and Yanez, L. 2004. DDT induces apoptosis in human mononuclear cells in vitro and is associated with increased apoptosis in exposed children. Environ. Res. 94:38–46.
- Pillai, V., Ortega, S. B., Wang, C. K., and Karandikar, N. J. 2007. Transient regulatory T-cells: A state attained by all activated human T-cells. Clin. Immunol. 123:18–29.
- Raziuddin, S., Nur, M. A., and Al-Wabel, A. A. 1990. Increased circulating HLA−DR+ CD4+ T-cell in systemic lupus erythematosus: Alterations associated with prednisolone therapy. Scand. J. Immunol. 31:139–145.
- Rea, W. J., Johnson, A. R., Youdim, S., Fenyves, E. J., and Samadi, N. 1986. T- and B-Lymphocyte parameters measured in chemically sensitive patients and controls. Clin. Ecol. 4:11–14.
- Roubinian, J. R., Talal, N., Greenspan, J. S., Goodman, J. R., and Siiteri, P. K. 1978. Effect of castration and sex hormone treatment on survival, anti-nucleic acid antibodies, and glomer-ulonephritis in NZB/NZW F1 mice. J. Exp. Med. 147:1568–1583.
- Rousset, F., Garcia, E., Defrance, T., Peronne, C., Hsu, D. H., Kastelein, R., Moore, K. W., and Banchereau, J. 1992. Interleukin-10 is a potent growth and differentiation factor for activated human B-lymphocytes. Proc. Natl. Acad. Sci. USA. 89:1890–1893.
- Roux, F., Treich, I., Brun, C., Desoize, B., and Fournier, E. 1979. Effect of lindane on human lymphocyte responses to phytohemagglutinin. Biochem. Pharmacol. 28:2419–2426.
- Sakaguchi, S. 2005. Naturally arising Foxp3-expressing CD25+CD4+ regulatory T-cells in immunological tolerance to self and non-self. Nat. Immunol. 6:345–352.
- Santiago, M. L., Fossati, L., Jacquet, C., Muller, W., Izui, S., and Reininger, L. 1997. Interleukin-4 protects against a genetically linked lupus-like autoimmune syndrome. J. Exp. Med. 185:65–70.
- Scheffold, A., Huhn, J., and Hofer, T. 2005. Regulation of CD4+CD25+ regulatory T-cell activity: It takes (IL-) two to tango. Eur. J. Immunol. 35:1336–1341.
- Silkworth, J. B., and Loose, L. D. 1979. Environmental chemical-induced modification of cell-mediated immune responses. Adv. Exper. Med. Biol. 12:499–522.
- Sobel, E. S., Gianini, J., Butfiloski, E. J., Croker, B. P., Schiffenbauer, J., and Roberts, S. M. 2005. Acceleration of autoimmunity by organochlorine pesticides in (NZB x NZW)F1 mice. Environ. Health Perspect. 113:323–328.
- Stekman, I. L., Blasini, A. M., Leon-Ponte, M., Baroja, M. L., Abadi, I., and Rodriguez, M. A. 1991. Enhanced CD3-mediated T-lymphocyte proliferation in patients with systemic lupus erythematosus. Arthritis Rheum. 34:459–467.
- Street, J. C., and Sharma, R. P. 1975. Alteration of induced cellular and humoral immune responses by pesticides and chemicals of environmental concern: Quantitative studies of immunosuppression by DDT, Arochlor 1254, Carbaryl, Carbofuran, methylparathion. Toxicol. Appl. Pharmacol. 32:587–602.
- Swain, S. L., Croft, M., Dubey, C., Haynes, L., Rogers, P., Zhang, X., and Bradley, L. M. 1996. From naive to memory T-cells. Immunol. Rev. 150:143–167.
- Tenbrock, K., and Tsokos, G. C. 2004. Transcriptional regulation of interleukin 2 in SLE T-cells. Int. Rev. Immunol. 23:333–345.
- USEPA (United States Environmental Protection Agency). 1989. Evaluation of Sample Extract Cleanup Using Solid-Phase Extraction Cartridges - Project report. Washington, DC: USEPA [online] Available at: http://nepis.epa.gov/Exe/ZyNET.EXE?ZyActionL=Register&User=anonymous&Password=anonymous&Client=EPA&Init=1><title>EPA%20-%20Home%20Page%20for%20the%20 Search%20site</title><link%20rel=. Accessed on 02 July 2011.
- Vine, M. F., Stein, L., Weigle, K., Schroeder, J., Degnan, D., Tse, C. K., and Backer, L. 2001. Plasma 1,1-dichloro-2,2-bis(p-chlorophenyl)ethylene (DDE) levels and immune response. Am. J. Epidemiol. 153:53–63.
- von Schmiedeberg, S., Fritsche, E., Rönnau, A. C., Specker, C., Golka, K., Richter-Hintz, D., Schuppe, H. C., Lehmann, P., Ruzicka, T., Esser, C., Abel, J., and Gleichmann, E. 1999. Polymorphisms of the xenobiotic-metabolizing enzymes CYP1A1 and NAT-2 in systemic sclerosis and lupus erythematosus. Adv. Exp. Med. Biol. 455:147–152.
- Wang, J., Ioan-Facsinay, A., van der Voort, E. I., Huizinga, T. W., and Toes, R. E. 2007. Transient expression of FOXP3 in human activated non-regulatory CD4+ T-cells. Eur. J. Immunol. 37:129–138.
- Yanez, L., Borja-Aburto, V. H., Rojas, E., de la Fuente, H., Gonzalez-Amaro, R., Gomez, H., Jongitud, A. A., and Diaz-Barriga, F. 2004. DDT induces DNA damage in blood cells. Studies in vitro and in women chronically exposed to this insecticide. Environ. Res. 94:18–24.
- Yu, C. R., Mahdi, R. M., Ebong, S., Vistica, B. P., Gery, I., and Egwuagu, C. E. 2003. Suppressor of cytokine signaling 3 regulates proliferation and activation of T-helper cells. J. Biol. Chem. 278:29752–29759.