Abstract
Polybrominated diphenyl ethers (PBDEs) are an important class of flame-retardants that are environmentally persistent and bioaccumulative. Toxicity of these compounds has become a concern because detectable levels of PBDEs are present in humans and wildlife and they are structurally similar to polychlorinated biphenyls (PCBs). This study examined the effects of the commercial penta-BDE mixture, DE-71, in adult female B6C3F1 mice on hematology, serum clinical chemistry, thyroid hormones, tissue histology, and several immunotoxicity end-points (lymphocyte proliferation, NK cell activity, splenic immunophenotypes, and SRBC-specific-IgM production). Mice were exposed via oral gavage for 28 days to achieve total administered doses (TAD) of 0, 0.5, 5, 50, or 100 mg/kg. No changes in histology, clinical chemistry, body or organ weights were observed. Serum total T3 and T4 levels were not altered by any of the DE-71 treatments. Peripheral blood monocyte numbers were decreased by the 0.5, 5, and 50 mg/kg treatments, but not by the 100 mg/kg TAD concentration. Compared to controls, mitogen-stimulated T- and B-cell proliferation was increased by the 100 mg/kg TAD concentration (ED50 = 60 mg/kg TAD [2.14 mg/kg/day] and 58 mg/kg TAD [2.57 mg/kg/day], respectively). NK cell activity was decreased compared to controls by the 100 mg/kg TAD concentration (ED50 = 20 mg/kg TAD [0.7 mg/kg/day]). No alterations were noted in thymic T-cell populations or in SRBC-specific-IgM production. Numbers of CD19+CD21−, CD19+CD21+, CD4+CD8−, CD4−CD8+, CD4−CD8−, and MHC-II+ cells in the spleen were not affected. However, the numbers of splenic CD4+CD8+ cells were decreased compared to the controls by 0.5, 5, and 100 mg/kg TAD. This study provides an assessment of the systemic toxicity and immunotoxicity of DE-71, and indicates that immune parameters are modulated at exposure concentrations lower than previously reported.
Introduction
Brominated flame retardants (BFRs) comprise a diverse group of chemicals that have been used in an array of commercial and industrial applications for fire prevention (Hakk and Letcher, Citation2003). Polybrominated diphenyl ethers (PBDEs) are one class of halogenated organic BFRs that are persistent in the environment. Three types of PBDE mixtures were manufactured and marketed: penta-BDE (containing penta-, tetra-, and some hexa-brominated congeners), octa-BDE (containing from hexa- to deca-brominated congeners), and deca-BDE (97% deca-BDE and 3% non-brominated congeners) (Mercado-Feliciano and Bigsby, Citation2008). These PBDE commercial mixtures have been used extensively as additives in industrial products due to their low cost and high performance efficiency for lowering flammability (Stoker et al., Citation2004). The penta-BDE mixture, DE-71, has been used primarily in textiles as an additive in polyurethane foams (Hale et al., Citation2002). These compounds are released into the environment as they are not chemically bound to the material they are intended to protect from burning.
Rising levels of PBDEs detected in environmental media, tissues of wildlife, and human tissues has raised concerns about possible health effects (de Wit, Citation2002; Sjödin et al. Citation2003; Birnbaum and Staskal, Citation2004; Costa et al., Citation2008; Vonderheide et al., Citation2008; Shaw and Kannan, Citation2009). The concentration of these chemicals in human serum and breast milk has exponentially increased in the last 3 decades (Noren and Meironyte, Citation2000; Sjödin et al., Citation2004; Schecter et al., Citation2005). Recent studies suggest that PBDEs may be neurotoxicants (Williams and DeSesso Citation2010), developmental neurotoxicants (Kodavanti et al., Citation2010), reproductive toxicants (Stoker et al., Citation2004; Darnerud, Citation2008), and endocrine disruptors (Darnerud, Citation2008). Immunomodulating effects of various xenobiotic classes including polycyclic aromatic hydrocarbons, halogenated aromatic hydrocarbons, organochlorine and organophosphorous pesticides, and heavy metals have been relatively well characterized (Dean, 1994). However, little is known about the immunological effects of PBDEs.
Only a few studies have characterized the potential immunotoxicity of PBDEs. Offspring of dams that received dietary exposure to deca-DBE from gestational day (GD) 10 through post-natal day (PND) 21 had increased susceptibility to respiratory syncytial virus (RSV), increased interferon (IFN)-γ in the bronchoalveolar lavage fluids and gene expression of the chemokine RANTES in the lungs of mice (Watanabe et al., Citation2008). In contrast, 5-week-old female BALB/c mice given 1% deca-BDE in their diet for 28 days exhibited no overt signs of toxicity and no increase in RSV titers (Watanabe et al., Citation2010a). Bondy et al. (Citation2011) reported no changes in delayed type hypersensitivity responses, antigen-specific IgG serum titers, or B-cell proliferation in Sprague-Dawley rats following lifetime exposure to DE-71 (gestation through 42 weeks); however, decreases in T-cell proliferation were observed in F1 females.
Suppression of sheep red blood cell (SRBC)-specific–immunoglobulin (Ig) M production but no alteration in natural killer cell (NK) activity was observed in mice orally exposed to DE-71 for 14 days (Fowles et al., Citation1994). Teshima et al. (Citation2008) demonstrated that, although Keyhole limpet hemocyanin-specific IgM titers did not change in Sprague-Dawley rats following dietary, GD10-PND21, exposure to deca-BDE, T-cell populations were reduced. This dietary exposure decreased CD4+/CD8− cells at PND21 with a subsequent rebound in this sub-population by PND77, while CD4+/CD25+Treg cells, NKRP1a+/CD4+ NK cells, and NKRP1A+/CD4− NKT cells were decreased at PND77 but not PND21. Thuvander and Darnerud (Citation1999) observed decreases in the percentage of thymic CD4−CD8− cells in mice along with decreased in vitro IgG secretion (36 mg/kg/d Bromkal70-5 DE given orally for 14 days). However, when PBDE (BDE-47 or -85) was administered to human lymphocytes in vitro, lymphocyte proliferation and immunoglobulin synthesis were not altered (Fernlöf et al., Citation1997). Studies with American kestrels (Falco sparverius) showed that PBDE exposure (BDE47, -99, -100, and −153 mixture) increased phytohemagglutinin (PHA) skin response, decreased anti-SRBC responses, reduced spleen sizes, reduced apoptosis in the bursa, and increased numbers of macrophages in the thymus (Fernie et al., Citation2005). In contrast, correlative studies with Kemp’s ridley sea turtles did not indicate relationships between immune parameters and PBDE serum levels (Swarthout et al., 2010).
PBDEs are structurally related to polychlorinated biphenyl (PCB) agents and have similar toxicological profiles (ATSDR, 2004; Birnbaum and Staskal, Citation2004) and are also known to modulate immune function. Thus, studies to assess the effects of PDBEs in a more comprehensive manner are warranted. We examined the effects of PBDEs in a murine model using traditional measures of immunotoxicity as outlined by Luster et al. (Citation1988), Citation1992), with a 28-day exposure required by the USEPA Health Effects Test Guidelines for Immunotoxicity (OPPTS 870.7800), a Harmonized Test Guideline. The total administered dose (TAD) levels in this study () were based, in part, on the range of PBDE blood levels observed in humans and wildlife (see ) and included higher concentrations to address potential increases related to reported doubling times (Johnson-Restrepo et al., Citation2005: Olsen et al., Citation2007).
Table 1. Calculations of administered DE-71 at the end of the 28-day exposure.
Table 2. Total administered dose (TAD) concentrations applied in this study were based on the following poly-brominated diphenyl ethers (PBDE) tissue concentrations found in humans and wildlife.
Materials and methods
Chemicals, antibodies, and supplies
Unless otherwise specified, all chemicals and mitogens were purchased from Sigma (St. Louis, MO). The penta-BDE technical mix DE-71 (≥ 98% chemical purity) was obtained from Cambridge Isotope Laboratories (Andover, MA); DE-71 is composed of six major congeners: BDE-99 > -47 > -100 > -153 > -154 > -85, ranging from 48.60–2.96% (wet weight) (La Guardia et al., Citation2006). Sheep erythrocytes (SRBC) in Alsever’s solution were purchased from Lonza (Walkersville, MD). Lyophilized guinea pig complement (GPC) and restoring solution, non-essential amino acids (NEAA; 10 mM, 100×) and sodium pyruvate (100 mM) were bought from GIBCO (Grand Island, NY). RPMI-1640 medium (with L-glutamine and sodium bicarbonate), phosphate-buffered saline (PBS; with or without Ca2+ and Mg2+), penicillin (5,000 IU/ml), and streptomycin (5000 µg/ml) were obtained from Cellgro (Mediatech, Herndon, VA). The radioisotopes sodium chromate ([51Cr]; specific activity = 351 mCi/mgCr), and tritium ([3H]; specific activity = 71 Ci/mmol) thymidine were acquired from ICN (Costa Mesa, CA). Fetal bovine serum (FBS) was from Gemini Bioproducts (Sacramento, CA). Fluorescein isothio-cyanate (FITC)-conjugated rat-anti-mouse CD4 and phycoerythrin (PE) conjugated rat-anti-mouse CD8 monoclonal antibodies (mAb) were purchased from Caltag (Carlsbad, CA). FITC-conjugated rat anti-mouse I-AP (MHC-II), Red-PE (R-PE)-conjugated rat anti-mouse CD21/CD35 (CR2/CR1, CD21a/CD21b), and FITC-conjugated CD19 mAbs were obtained BD Biosciences (San Jose, CA). Goat anti-mouse IgM horseradish peroxidase was purchased from Accurate Chemical and Scientific Corp (Westbury, NY). Luma Plates™, Unifilters®, and Microscint 20™ were procured from Packard (Meriden, CT). YAC-1 cells were purchased from ATCC (Manassas, VA). Flat-bottom 96-well Immuno-lon-2 ELISA microtiter plates for the IgM ELISA were obtained from Dynatech Labs (Chantilly, VA). Triton-X, tissue culture plates, and disposables were from Fisher Scientific (Atlanta, GA).
Animal care
Mice were housed in plastic shoebox cages on corncob bedding with micro-isolator lids in a HEPA filtered ventilated rack system and administered food (TekLab Sterilizable Rodent Diet, formula no. 8656; Harlan-Teklab, Madison, WI) and water ad libitum. For each treatment group, five 7–8-week-old female B6C3F1 mice (Harlan, Madison, WI) were acclimated to the conditions of the treatment room (12-h light/dark cycle, 22 ± 2°C, 60–65% relative humidity) for 1 week before dosing began. Bedding, food, and water were changed twice weekly and mice were observed daily. All procedures were approved by the Medical University of South Carolina Institutional Animal Care and Use Committee (IACUC) and conducted in an Association for Assessment and Accreditation of Laboratory Animal Care (AAALAC) accredited facility.
Animal dosing
DE-71 was administered via oral gavage (volume = 80–110 μl depending on weight) in a solution of canola oil containing 10% DMSO. Control mice were administered 10% DMSO in canola oil. Mice were dosed daily for 28 days (0, 0.017, 0.17, 1.7, or 3.6 mg/kg/day) to yield a TAD over the 28 days of 0, 0.5, 5, 50, or 100 mg/kg TAD (). No anesthesia was required or utilized for each gavaging procedure. Mice were weighed weekly and volumes adjusted if needed to maintain the TADs.
End-point assessment
The experiment was repeated three times (three trials). Due to the limited amount of blood available from mice, clinical chemistry, hematology, thyroid hormones, and SRBC-specific IgM titers were assessed singly from serum collected from one of the three trials. Lymphocyte proliferation was assessed in all three trials. NK cell activity, total cellularity, and immunophenotype of cells from the thymus and spleen were assessed in two of the three trials.
Body and organ mass, and immune organ cellularity
To calculate mass change over the 28 days, body mass was measured 1 day prior to exposures and at the termination of the experiment (weight change = final mass − start mass). Spleen, thymus, liver, kidney, uterus, brain, lung, and adrenals were collected and weighed following euthanization in a CO2-saturated environment. Organ mass was normalized for body weight and reported as a somatic index ((organ weight/body wt) × 100). Organs for histopathology assessment were placed in tissue cassettes and stored in formalin until analysis. Immune organs (spleen and thymus) were aseptically processed into single cell suspensions with the use of sterile, frosted microscope slides. Spleen and thymus samples collected from the two experimental trials that did not assess immune organ histology were assessed for differences in cellularity. Viable cell counts for sample dilutions and total cellularity determinations were obtained via trypan blue exclusion using a hemacytometer following red blood cell (RBC) lysis.
Serum chemistries and hematology
Twenty-four hours after the last day of exposure (day 28), blood was collected under isoflurane anesthesia. Whole blood was collected via the retro-orbital sinus into an EDTA-coated microtainer for hematological analysis or a non-heparinized Eppendorf tube for serum chemistries, respectively. Immediately following blood collection, mice were euthanized with CO2. Blood collected without anti-coagulant was permitted to clot for 1 h. After clot formation, the blood sample was centrifuged for 10 min at 1350 x g using a microcentrifuge (Eppendorf, 5415C) and serum was transferred into an Eppendorf tube. Samples were kept cool until processed and shipped on frozen gel packs (wrapped and insulated to prevent freezing but to allow for sample to remain cool during shipping) to the Cornell University Veterinary Diagnostic Laboratory (Ithaca, NY) for analysis.
Complete blood cell counts (CBC) (white blood cells, red blood cells, hemoglobin, packed cell volume, mean corpuscular volume, mean corpuscular hemoglobin concentration, red blood cell distribution width, mean platelet volume, and platelets) were determined using an automated analyzer (Bayer ADVIA 120, Bayer Diagnostics, Tarrytown, NY). Differential leukocyte counts (neutrophils, lymphocytes, eosinophils, and monocytes), as part of the CBC, were performed by microscopic examination of modified Wright-Giemsa stained blood smears. Serum chemistry analytes were measured with an automated analyzer (Roche Hitachi 917, Indianapolis, IN) and included: glucose, sodium, potassium, chloride, calcium, phosphate, blood urea nitrogen (BUN), creatinine, total bilirubin, alkaline phosphatase (ALP), alanine aminotransferase (ALT), aspartate transaminase (AST), creatine phosphokinase (CPK), gamma-glutamyl transferase (GGT), amylase, triglyceride, and iron.
Thyroid hormones
Twenty-four hours after the last day of exposure (day 28), whole blood was collected for the analysis of total triiodothyronine (TT3) and total thyroxine (TT4). Whole blood was permitted to clot for 1 h. After clot formation, the blood sample was centrifuged for 10 min (1350 x g) using a micro-centrifuge (Eppendorf, 5415C) and the resultant serum was transferred into an Eppendorf tube. Samples were kept cool until processed and shipped (as above) to the Cornell University Veterinary Diagnostic Laboratory (Ithaca, NY) for thyroid hormone measurements. TT4 and TT3 were measured by solid-phase [125I]-radioimmunoassays (RIA) using commercially-available kits (Coat-A-Coat® TT4, Siemens Heathcare Diagnostic Inc, Los Angeles, CA; Coat-A-Coat Total® TT3, Diagnostic Product Corp, Los Angeles, CA). Thyroid RIA was validated according to procedures described in Reimers et al. (Citation1981). All results met criteria for quality assurance, precision, and percent coefficient of variation. Limits of detection were 0.11 μg/dl for TT4 and 0.08 ng/dl for TT3.
Histology
Tissue samples of spleen, thymus, liver, adrenal, lung, brain, uterus, and kidney were placed in 10% neutral buffered formalin, embedded in paraffin, sectioned at 5 µm, and stained with hematoxylin and eosin for examination by light microscopy. Spleen and thymus samples were obtained from one of the experimental trials and a small section was collected for histopathology, while the remaining samples were used for immune assessments. A veterinary pathologist assessed the slides for abnormalties and treatment groups were blinded to the evaluator.
Lymphocyte proliferation
Spleens were processed and diluted to 2 × 106 nucleated cells/ml in RPMI-1640 (with 10% fetal bovine serum (FBS), 1% non-essential amino acids (NEAA), 1% sodium pyruvate, 10 mM HEPES, 1% penicillin/streptomycin, and 10 µM 2-mercaptoethanol) that had been adjusted to pH 7.4. Mitogens used were 2.5 µg ConA/ml (concanavalin A, Type IV-S) and 5 µg LPS/ml (lipopolysaccharide; E. coli 0111:B4) as previously described (Peden-Adams et al., Citation2008).
Natural killer (NK) cell activity
Natural killer cell activity was assessed via an in vitro cytotoxicity assay using 51Cr-labeled YAC-1 cells (Duke et al., Citation1985; Holsapple et al., Citation1988) with slight modifications to methods described in Peden-Adams et al. (Citation2008). The results are expressed in lytic units/107 splenocytes using 10% lysis as the reference point (Bryant et al., Citation1992).
Splenic and thymic immunophenotypes
Spleen and thymus samples collected from the two experimental trials that did not assess immune organ histology were assessed for differences in immunophenotypes. Spleen or thymus cells were labeled with fluorescent (PE- or FITC-conjugated) rat IgG2 mAbs specific for mouse CD4, CD8, CD19, CD21, and MHC-II and assessed by flow cytometry as previously described (Peden-Adams et al., Citation2008).
SRBC-specific IgM serum titers
Five days prior to euthanasia, mice were administered 0.1 ml of a 25% sheep red blood cell (SRBC) suspension in PBS via intraperitoneal injection (Harper et al., Citation1993). Serum was collected as described above from the samples to be assessed for DE-71 concentrations and stored at −20°C until analysis. Relative SRBC-specific IgM serum titers were measured by ELISA, as described previously (DeWitt et al., Citation2005). Briefly, flat-bottom ELISA microtiter plates were coated with 125 µl of a 2 µg/ml solution of SRBC (1.46 mg/ml stock solution diluted in phosphate buffer solution, as described by Temple et al. (Citation1993), and then incubated at 4°C for at least 16 h. Each plate included 20 wells that were coated with pooled serum collected from healthy mice 5 days after primary immunization with SRBC; an additional 16 wells had 100 µl phosphate buffer solution only. After washing, blocking of non-specific binding, and addition of serum samples (serially diluted beginning at a 1:6 or 1:7 starting dilution), secondary antibody (goat anti-mouse IgM horseradish peroxidase) was added. Following three washes, 100 µl ABTS substrate (2,2′-azino-di-3-ethylbenzthiazoline sulfonic acid: Sigma) was added to each well; the plates were incubated for 45 min at room temperature and then read at 410 nm on a SpectraMax 350 plate reader (Molecular Devices, Sunnyvale, CA). IgM antibody titers were quantified using SOFTmax Pro software (Molecular Devices) to determine the log2 serum titer for an optical density of 0.5 U from the log–log curve of optical density vs dilution (Temple et al., Citation1993).
Liver PBDE analysis
Liver samples were stored at −80°C until extraction. Concentrations of PBDEs in mouse liver were determined by a method previously described (Johnson-Restrepo et al., Citation2005; Olsen et al., Citation2007). Tissues (~ 1 g wet weight) were homogenized with anhydrous sodium sulfate and were Soxhlet extracted using dichloromethane and hexane (3:1 v/v; 400 ml). Fat content was determined gravimetrically from an aliquot of the extract. PCB congeners CB-30 and CB-204 were spiked as surrogate standards prior to extraction. The extracts were spiked with [13C]-labeled PBDE congeners as internal standards (Cambridge Isotope Laboratories, Andover, MA), and were then purified by passage through 6 g of multi-layer silica gel (100–200 mesh) column packed in a glass column (10 mm i.d.). The silica gel column was prepared by packing 2 g of silica gel, followed by 2 g of 40% acidic silica, and then by 2 g of silica gel. The silica gel bed was washed with 100 ml of hexane prior to loading of the sample extract. Extracts were then eluted with 150 mL of 25% dichloromethane in hexane. Samples were further subjected to lipid removal by treatment with concentrated sulfuric acid.
Sample extracts (2 µl) were injected into a Hewlett-Packard (HP) 6890 gas chromatograph interfaced with an HP 5973 mass spectrometer (GC-MS) (Foster City, CA). Injections were made in the splitless mode, and samples were separated on a 30 m DB-5 (5% diphenyl/dimethylpolysiloxane) analytical capillary column with a 250 µm i.d. and a 0.25 µm film thickness. The oven temperature program was set at 100°C for 1 min, and then increased by 10°C/min to 160°C for 3 min, and increased by 2.5°C/min to 260°C, then held for 10 min. The inlet and interface temperatures were set at 270°C and 300°C, respectively. The MS was operated in an electron impact (70 eV) and selected ion monitoring mode (SIM). PBDE congeners were monitored at m/z 406, 408; 486, 484; 564, 566; and 642, 644 for tri-; tetra-; penta-; and hexa-BDEs, respectively. Quantification was based on an external calibration standard. Eighteen congeners representing tri- through hexa-BDEs (congener # 17, 28, 51, 49, 48, 47, 66, 102, 100, 119, 91, 99, 85, 154, 153, 139, 140, and 138) were quantified. The detection limit of individual PBDE congeners varied from 0.2–3 ng/g, wet weight. Mean recoveries of PCB-30 and PCB-204, spiked into samples prior to extraction, were 94% and 104%, respectively. Recovery of 13C-PBDEs spiked into samples prior to lipid removal ranged from 86–99%. Values for liver below the lower limit of quantitation (LLQ) were set to ½ LLQ for calculation of total PBDE (ΣPBDE).
Statistics
Data were tested for normality (Shapiro-Wilks W-test) and homogeneity (Bartlett’s test for unequal variances) and, if needed, appropriate transformations were made. For parametric data, a one-way ANOVA was used to determine differences among doses for each end-point using JMP 4.0.2 (SAS Institute Inc., Cary, NC) in which the standard error used a pooled estimate of error variance. Dunnett’s t-test was used to compare treatment groups to the control group. Natural killer cell data were additionally tested by Kendall’s Tau for relationship analysis (p < 0.05). The dose–response for immune end-points was assessed by regression analysis. The log transformation of the end-point was plotted against the log dose. The log dose needed to achieve 50% suppression/stimulation of the immune response was determined from the regression equation and the inverse log calculated to determine the calculated ED50.
Results
Liver PBDE concentration
Concentrations of PBDE congeners in the liver collected on day 29 (24 h after the final exposure) were determined. The measured liver concentrations are presented in . The ΣPBDE concentration for liver ranged on average from 14.4 (± 1.0) to 5350 (± 634) ng/g wet weight or (328 [± 52.7] to 121,000 [± 24,900] ng/g lipid wt).
Table 3. Liver PBDE concentrations (ng/g) on both a wet weight (w.w) and lipid weight basis in female B6C3F1 mice following a 28-day oral exposure to DE-71.
Body and organ mass and immune organ cellularity
There were no signs of overt toxicity in any of the animals as indicated by weight loss, lack of activity, lack of grooming, or cloudy eyes. Mice in all groups appeared to gain weight equally over the course of the 28-day study (data not shown). Secondary immune organ mass (spleen and thymus) was not altered, nor was liver, kidney, or uterus mass (data not shown). Brain, adrenal, and lung mass also exhibited no statistical change as compared to controls (data not shown). Cellularity of the spleen and thymus was not altered following treatment with DE-71 at any of the dose levels assessed (data not shown).
Hematology, serum clinical chemistry, thyroid hormones, and organ histology
The only hematological parameter altered was numbers of peripheral blood monocytes. Monocyte numbers were significantly decreased in the 0.5, 5, and 50 mg/kg TAD treatment groups as compared to controls by 77%, 77%, and 92%, respectively (). Serum clinical chemistry profiles (data not shown) and serum TT3 and TT4 levels were not significantly affected by DE-71 treatment (). No histological alterations were noted in any of the organs assessed (data not shown).
Figure 1. Peripheral blood monocyte counts in adult female B6C3F1 mice following oral exposure to DE-71 for 28-days. Data are presented as mean ± SEM. This experiment was conducted once. Sample size for all treatments was five. TAD = Total Administered Dose (daily doses are 1/28 of the TAD). * Significantly different from control (p ≤ 0.05).
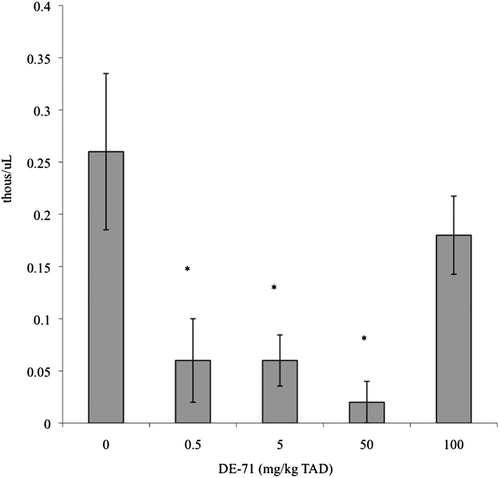
Figure 2. Serum total T3 (a) and total T4 (b) levels in adult female B6C3F1 mice following oral exposure to DE-71 for 28-days. Data are presented as mean (± SEM). This experiment was conducted once. Sample size for all treatments was five. TAD = Total Administered Dose (daily doses are 1/28 of the TAD).
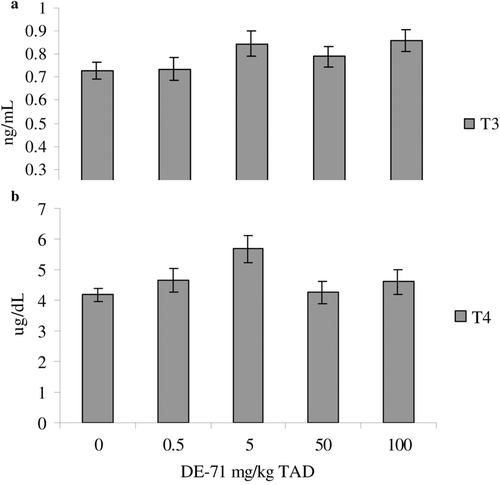
Lymphocyte proliferation, NK-cell function, SRBC-specific IgM
ConA- or LPS-induced lymphocyte proliferation following exposure to 100 mg DE-71/kg TAD (3.6 mg/kg/day) was significantly increased over controls (). The calculated effective dose values at 50% (ED50) for these increased responses were 60 mg/kg TAD and 58 mg/kg TAD, respectively (ConA ED50 = 60 mg/kg TAD = 2.14 mg/kg/day [y = 0.2006x2 − 0.2498x + 1.8589; R2 = 0.9639]; LPS ED50= 58 mg/kg TAD = 2.07 mg/kg/day [y = 0.179x2 − 0.219x + 2.1205; R2 = 0.8576]). NK-cell activity was significantly decreased by 80% following exposure to 100 mg DE-71/kg TAD (3.6 mg/kg/day; ). The calculated ED50 for this response was 20 mg/kg TAD (0.71 mg/kg/day; y = −0.1412x2 − 0.0145x + 1.4478; R2 = 0.9228) and the relationship between NK activity and administered DE-71 concentration exhibited a significant negative relationship. Serum levels of SRBC-specific-IgM were not significantly altered by any treatment ().
Figure 3. Splenic lymphocyte proliferation. Splenic lymphocyte proliferation in adult female B6C3F1 mice was measured using the tritiated thymidine method following oral exposure to DE-71 for 28-days. Unstimulated cpm counts were not significantly different between treatment groups; therefore, data are represented as the stimulation index (SI = cpm stimulated/cpm unstimulated). Data are presented as mean SI ± SEM. Sample size for all treatments was five. The cpm of the unstimulated wells averaged 701 ± 50 (mean [± SEM]). T-Cell mitogen ConA was used at a final concentration of 2.5 μg/ml; LPS from E. coli (5 μg/ml final concentration) was used to stimulate B-cells. This experiment was conducted three times. Data from a single experiment are shown, as results were representative of all experiments. TAD = Total Administered Dose (daily doses are 1/28 of the TAD). * Indicates statistical difference from controls (p ≤ 0.05). The calculated ED50s for these responses were 60 mg/kg TAD and 58 mg/kg TAD, respectively (ConA ED50 = 60 mg/kg TAD = 2.14 mg/kg/day [y = 0.2006x2 − 0.2498x + 1.8589; R2 = 0.9639]; LPS ED50 = 58 mg/kg TAD = 2.07 mg/kg/day [y = 0.179x2 − 0.219x + 2.1205; R2 = 0.8576]).
![Figure 3. Splenic lymphocyte proliferation. Splenic lymphocyte proliferation in adult female B6C3F1 mice was measured using the tritiated thymidine method following oral exposure to DE-71 for 28-days. Unstimulated cpm counts were not significantly different between treatment groups; therefore, data are represented as the stimulation index (SI = cpm stimulated/cpm unstimulated). Data are presented as mean SI ± SEM. Sample size for all treatments was five. The cpm of the unstimulated wells averaged 701 ± 50 (mean [± SEM]). T-Cell mitogen ConA was used at a final concentration of 2.5 μg/ml; LPS from E. coli (5 μg/ml final concentration) was used to stimulate B-cells. This experiment was conducted three times. Data from a single experiment are shown, as results were representative of all experiments. TAD = Total Administered Dose (daily doses are 1/28 of the TAD). * Indicates statistical difference from controls (p ≤ 0.05). The calculated ED50s for these responses were 60 mg/kg TAD and 58 mg/kg TAD, respectively (ConA ED50 = 60 mg/kg TAD = 2.14 mg/kg/day [y = 0.2006x2 − 0.2498x + 1.8589; R2 = 0.9639]; LPS ED50 = 58 mg/kg TAD = 2.07 mg/kg/day [y = 0.179x2 − 0.219x + 2.1205; R2 = 0.8576]).](/cms/asset/3daed999-88fa-400c-bb23-7f2bd31189f4/iimt_a_643418_f0003_b.gif)
Figure 4. Splenic natural killer (NK) cell activity. Splenic NK activity in adult female B6C3F1 mice was measured using a standard chromium release method following oral exposure to DE-71 for 28 days. Data are presented as mean (± SEM). Sample size for all treatments was five. LU = lytic units. This experiment was conducted twice. Data from a single experiment are shown, as results were representative of both experiments. * Indicates statistical difference from controls (p ≤ 0.05). The calculated ED50 for this response was 20 mg/kg TAD (0.71 mg/kg/day; y = −0.1412x2 − 0.0145x + 1.4478; R2 = 0.9228). Additionally, a significant decreasing relationship was observed with increasing DE-71 dose (Tau b = −0.57; p < 0.05). TAD = Total Administered Dose over the course of 28 days (daily doses are 1/28 of the TAD).
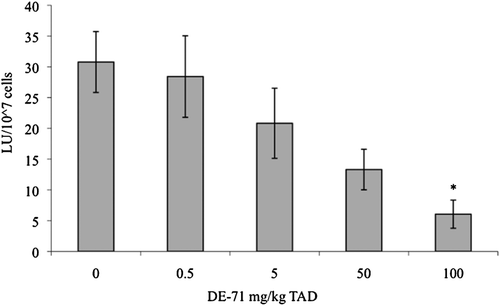
Figure 5. SRBC-specific IgM titers. SRBC-specific IgM titers in adult female B6C3F1 mice were measured by ELISA following oral exposure to DE-71 for 28 days. Data are presented as mean (± SEM). This experiment was conducted once. Sample size for all treatments was five, with the exception of 0.5 mg/kg TAD samples (n = 4). TAD = Total Administered Dose (daily doses are 1/28 of the TAD).
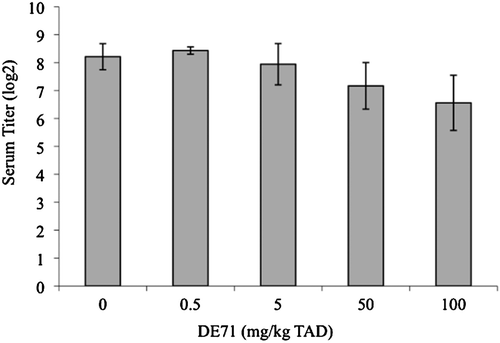
Lymphocyte immunophenotypes
The numbers of thymic CD4/CD8 cells were not altered by DE-71 treatment (data not shown). In the spleen, the only change observed in CD4±CD8± cell numbers was in CD4+CD8+ cells. Compared to controls, numbers of CD4+CD8+ cells were significantly decreased by 50%, 57%, and 51%, respectively, by 0.5, 5, and 100 mg/kg TAD (). Absolute numbers of splenic B-cells (CD19+CD21− and CD19+CD21+) and MHC-II+ cells were not changed following DE-71 exposure (). Numbers of CD19−CD21+ cells that most likely represent follicular dendritic cells were also not altered by DE-71 treatment (data not shown). The CD4+:CD8+ ratio was not altered in either the spleen or thymus (data not shown).
Table 4. Splenic lymphocyte sub-populations in adult B6C3F1 mice following oral exposure with DE-71 for 28 days.
Discussion
This is the first study to examine the effects of a PBDE technical mixture (DE-71) on traditional National Toxicology Program tiered immunotoxicity test end-points (hematology, histology, organ weights, T-cell sub-populations, NK cell activity, lymphocyte proliferation, and SBRC-specific IgM production) following the 28-day oral exposure experimental design described by the USEPA Health Effects Test Guidelines for Immunotoxicity (OPPTS 870.7800). While a few rodent studies have previously assessed immune modulation following exposure to DE-71 (Fowles et al., Citation1994; Kodavanti et al., Citation2010), Bromkal 70-5DE (containing 60% penta-BDE and 40% hexa-BDE; Darnerud and Thuvander, Citation1999), BDE47 and BDE85 (Fernlöf et al., Citation1997), and deca-BDE (Teshima et al., Citation2008; Wantanabe et al., Citation2008, Citation2010a, Citationb), this study demonstrated that exposure to DE-71 led to decreased numbers of peripheral blood monocytes and splenic CD4+CD8+ T-cells and suggested that immune function may be modulated at environmentally relevant concentrations.
The current study identified changes in the distribution of immunological cells and in cell function, but did not detect alterations in total T3 or T4 serum levels. Alterations in circulating levels of thyroid hormones are generally considered a sensitive toxicological endpoint following PBDE exposure in humans and experimental models (Fowles et al., Citation1994; Zhou et al., Citation2001; Darnerud et al., Citation2007; Turyk et al., Citation2008; Dallaire et al., Citation2009). To our knowledge, an acute oral exposure to 0.8 mg/kg DE-71 in female C57Bl/6 mice was the lowest concentration ever reported to modulate thyroid hormones. This concentration was associated with a decrease in total and free T4 serum levels, but immune function was not altered by any of the acute exposures (0.8–500 mg/kg DE-71; Fowles et al., Citation1994). However, Fowles et al. (Citation1994) did observe decreased SRBC-specific IgM production and decreased thymus mass when the mice were given 1000 mg/kg DE-71 TAD (71.4 mg/kg/d) for 14 days. Total and free T4 were more sensitive endpoints as they were decreased by 250 mg/kg DE-71 TAD (17.9 mg/kg/day) (Fowles et al., Citation1994).
In the current study, T- and B-cell proliferation and NK cell activity were significantly different from control mice at a 100 mg/kg TAD. These effects were observed at a total DE-71 concentration that is 100-fold less than the immune effects previously reported by Fowles et al. (Citation1994). Strikingly, the LOAEL (0.5 mg/kg TAD; 0.018 mg/kg/day) that we report (as indicated by both decreased peripheral blood monocyte numbers and decreased numbers of splenic CD4+/CD8+ cells) is 2000-fold lower than previously reported immune effects. In addition, in the current study, immune parameters (changes in cell numbers and function) were affected while thyroid hormone levels were not altered, suggesting that in B6C3F1 mice given PBDE, immune function is a more sensitive end-point than thyroid hormone levels in PBDE-exposed C57BL/6 mice. Although these differences may be strain-related, they are more likely related to duration and frequency of exposure. The current data suggest that lower daily doses for a longer duration result in immune alterations that were not previously detected.
Although peripheral blood monocytes were significantly decreased beginning at the 0.5 mg/kg treatment, it is unclear if this would lead to deficits in disease resistance. Monocytes are a relatively small fraction of immune cells in peripheral circulating blood, but are important in innate immune surveillance. There were no concomitant decreases in the numbers of splenic MHC-II+ cells in the current study. The MHC-II marker, however, is found on dendritic cells and B-cells, as well as on macrophages (monocytes that have infiltrated tissue). Further studies should assess the numbers of macrophages in the spleen using a more specific macrophage cell surface marker such as F4/80, and examine macrophage function (e.g., phagocytosis, nitric oxide production).
Both CD4+ and CD8+ splenic sub-populations were decreased with the 0.5, 5, and 100 mg/kg DE-71 TAD; however, there was no concomitant effect on this lymphocyte sub-population in the thymus. The classic pathway of T-cell maturation is that T-cells mature in the thymus from CD4+CD8+ cells to either CD4+CD8− or CD4−CD8+ cells, which then migrate to the periphery and secondary immune organs. The presence of extra-thymic CD4+CD8+ T-cells has been largely disregarded (CitationZuckermann, 1998). Early studies indicate that mice exhibit a sub-set of intraepithelial lymphocytes (IEL) that exhibit both CD4+ and CD8+ that appear to be mature T-cells rather than immature cells (reviewed in Zuckerman, 1999). In humans, CD4+CD8+ T-cells seem to be important in tumor regulation (Desfrancois et al., Citation2009, Citation2010). Additionally, CD4+/CD8+, a distinct macrophage sub-set, has been identified in rats that exhibit cytotoxic tumor killing capacity (Baba et al., Citation2006, Citation2008). CD4 is constitutively expressed on macrophages and monocytes from humans and rats (Jeffries et al., Citation1985; Wood et al., Citation1985; Baba et al., Citation2006), but this has not been confirmed in mice (Baba et al., Citation2006). Furthermore, increased numbers of CD4+CD8+ T-cells have been noted in lymph and bronchoalveolar lavage fluid from mice following exposure to viruses (Periwal and Cebra, Citation1999; Hillemeyer et al., Citation2002) and studies suggest that CD4 expression on CD8 T-cells enhances the response to viral and alloantigens in mice (Kitchen et al., Citation2005).
Although some information exists regarding extra-thymic CD4+/CD8+ cells, their origin, function, and importance are just beginning to come to light and much is still unknown. The observed decrease in splenic CD4+CD8+ cells following DE-71 treatment indicates a cell sub-population was modulated. However, the importance of this finding in light of the scarcity of information on this cell population is unclear. It is possible that since CD4+CD8+ macrophages have been identified in rats, this decrease might be associated with deficits in peripheral blood monocytes; however, to our knowledge no CD4+CD8+ macrophages have been identified in mice (Baba et al., Citation2006). Increases in susceptibility to RSV following GD10-PND21 exposure to deca-BDE (Wantanabe et al., Citation2010b) suggest that the decreases in splenic CD4+CD8+ cells could be linked to increased viral susceptibility. Perhaps the decrease in CD4+CD8+ cells (assumed to be T-cells) indicates deficiencies in protection from viral pathogenesis. However, caution is warranted in making that assumption since in 5-week-old female BABL/c mice, no increase in RSV titer was observed following a 28-day exposure to a TAD of ~ 52,800 mg/kg DBDE (Watanabe et al., Citation2010a). As the current study assessed DE-71, a penta-DBE mixture rather than deca-BDE, additional studies are required to better characterize this CD4+CD8+ population in the spleen and assess tumor and viral host resistance to confirm potential links.
The LOAEL (0.5 mg/kg TAD; 0.018 mg/kg/day, 500 ppb TAD) in this study was established by both decreased peripheral blood monocyte numbers and decreased numbers of splenic CD4+CD8+ cells. As this dose was the lowest DE-71 concentration tested, the NOAEL could not be determined. Our LOAEL is similar to the upper ranges of ΣPBDE serum levels reported in Schecter et al. (Citation2005) and Windham et al. (Citation2010) (366 and 855 ppb, respectively). Using the mean human serum values presented in to calculate a margin of exposure (MOE), which is the ratio of the NOAEL (or LOAEL in the absence of a NOAEL) in an animal study to exposure levels estimated in the human population, we obtain a range of 0.9–12.3. Calculation of the MOE with the NOAEL, were it available, would further decrease the MOE. MOE values less than 100 may serve as an alert for further testing (Faustman and Omenn, Citation2001). Estimated serum levels of BDE-47 based on the liver:serum ratio of 11.4:1 for B6C3F1 mice reported by Sanders et al. (Citation2006) indicate that treatment concentrations fall within ranges reported in humans (). Taken together, this suggests a possible risk of immunotoxicity at current human exposures. Data on the mechanism of the observed effects along with utilization of a parallelogram approach (Selgrade et al., Citation1995) would decrease uncertainty and provide information needed to better assess human health risk.
Table 5. Estimated mean serum BDE-47 concentration for the B6C3F1 mice in the current study in relation to geometric mean reported in the 2003/2004 National Health and Nutrition Examination Survey (NHANES).
This study comprises the first assessment of traditional immunotoxicity measures as defined by the USEPA Health Effects Test Guidelines for Immunotoxicity (OPPTS 870.7800) and suggests that DE-71 can modulate immune function at environmentally relevant concentrations. Numbers of peripheral blood monocytes and splenic CD4+CD8+ T-cells were decreased at the lowest dose tested. These effects occurred at concentrations where thyroid hormones were not modulated. In fact, no changes in thyroid hormones were noted at any DE-71 concentration tested in the current study. Based on these findings and reported PBDE concentrations in humans and wildlife, continued investigation is warranted to determine the effects and mechanisms of PBDEs on immunity and health parameters at low exposure concentrations to better predict risk.
Acknowledgements
The authors would like to thank Dr. Gary Gilkeson, Ms. Jackie EuDaly and Ms. Rachel Sayre for their technical assistance. The authors would like to thank the following reviewers for their critical review of the manuscript: Dr. Malcolm Meaburn, Mr. John Bemiss and Mr. Tod Leighfield. We acknowledge the Medical University of South Carolina (MUSC) and the Hollings Cancer Center for their support of the Flow Cytometry & Cell Sorting Shared Resource Facility. This publication does not constitute an endorsement of any commercial product or intend to be an opinion beyond scientific or other results obtained by the National Oceanic and Atmospheric Administration (NOAA). No reference shall be made to NOAA, or this publication furnished by NOAA, to any advertising or sales promotion that would indicate or imply that NOAA recommends or endorses any proprietary product mentioned herein, or which has as its purpose an interest to cause the advertised product to be used or purchased because of this publication.
Declaration of interest
The authors report no conflicts of interest. The project was funded by the National Oceanic and Atmospheric Administration (NOAA)/National Ocean Service/Center for Coastal Environmental Health and Biomolecular Research.
References
- Agency for Toxic Substances and Disease Registry (ATSDR). 2004. Toxicological Profile for Polybrominated biphenyls and polybrominated diphenylethers. U.S. Department of Health and Human Services, Public Health Service Agency for Toxic Substances and Disease Registry.
- Athanasiadou M., Cuadra, S. N., Marsh G., Bergman A., and Jakobsson, K. 2007. Polybrominated diphenyl ethers (PBDEs) and bioaccumulative hydroxylated PBDE metabolites in young humans from Managua, Nicaragua. Environ. Health Perspect. 116:400–440.
- Baba T., Ishizu A., Suzuki A., Tomaru U., Ikeda H., Yoshiki T., and Kasahara M. 2006. CD4+/CD8+ macrophages infiltrating at inflammatory sites: A population of monocytes/macro-phages with cytotoxic phenotype. Immunobiology 107:2004–2012.
- Baba T., Iwasaki, S., Maruoka T., Suzuki, A., Tomaru, U., Ikeda H., Yoshiki, T., Kasahara M., and Ishizu A. 2008. Rat CD4+CD8+ macrophages kill tumor cells through an NKG2D- and granzyme/perforin-dependent mechanism. J. Immunol. 180:2999–3006.
- Bernert, J. T., Wayman Turner, E., Patterson, D. J. Jr., and Needham L. L. 2007. Calculation of serum “total lipid” concentrations for the adjustment of persistent organohalogen toxicant measurements in human samples. Chemosphere 68:824–831.
- Birnbaum L. S., and Staskal D. F. 2004. Brominated flame retardants: Cause for concern? Environ. Health Perspect. 112:9–17.
- Bondy G. S., Lefebvre D. E., Aziz, S., Cherry, W., Coady L., Maclellan E., Armstrong C., Barker M., Cooke G., Gaertner D., Arnold D.L., Mehta R., and Rowsel, P. R. 2011. Toxicologic and immunologic effects of perinatal exposure to the brominated diphenyl ether (BDE) mixture DE-71 in the Sprague-Dawley rat. Environ Toxicol. doi: 10.1002/tox.20713. [Epub ahead of print]
- Bryant, J., Day R., Whiteside T. L., and Herberman R. B. 1992. Calculation of lytic units for the expression of cell-mediated cytotoxicity. J. Immunol. Meth. 146:91–103.
- Codru N., Schymura M. J., Negoita S., Rej R., and Carpenter, D. O. 2007. Diabetes in relation to serum levels of polychlorinated biphenyls and chlorinated pesticides in adult native Americans. Environ. Health Perspect. 115:1442–1447.
- Costa L. G., Giordano, G., Tagliaferri S., Caglieri, A., and Mutti, A. 2008. Polybrominated diphenyl ether (PBDE) flame retardants: Environmental contamination, human body burden and potential adverse health effects. Acta Biomed. 79:172–183.
- Dallaire R., Dewailly, É, Pereg, D., Dery S., and Ayotte, P. 2009. Thyroid function and plasma concentrations of polyhalogenated compounds in Inuit adults. Environ. Health Perspect. 117:1380–1386.
- Darnerud, P. O. 2008. Brominated flame retardants as possible endocrine disrupters. Int. J. Androl. 31:152–160.
- Darnerud, P. O., Aune M., Lotta Larsson L., and Hallgren S. 2007. Plasma PBDE and thyroxine levels in rats exposed to Bromkal or BDE-47. Chemosphere 67:S386–S392
- Darnerud, P. O., and Thuvander A. 1999. Effects of polybrominated diphenyl ether (PBDE) and polychlorinated biphenyl (PCB) on some immunological parameters after oral exposure in rats and mice. Toxicol. Environ. Chem. 70:229–242.
- de Wit C. A. 2002. An overview of brominated flame retardants in the environment. Chemosphere 46:583–624.
- Dean J.H. (Ed.).1994. Immunotoxicology and immunopharmacology: Target Organ Toxicology Series. 2nd Edition. Boca Raton, FL: CRC Press.
- Desfrancois J., Derre L., Corvaisier M., Le Mevel B., Catros, V., Joereau F., and Gervois N. 2009. Increased frequency of nonconventional double positive CD4/CD8 αβ T-cells in human breast pleural effusions. Int. J. Cancer 125:374–380.
- Desfrancois, J., Moreau-Aubry A., Vignard V., Godet Y., Khammari A., Dreno B., Jotereau, F., and Gervois N. 2010. Double positive CD4/CD8 αβ T-cells: A new tumor-reactive population in human melanomas. PLoS ONE. 5:e8437.
- DeWitt, J. C., Copeland, C. B., and Luebke R. W. 2005. Immune responses in Sprague-Dawley rats exposed to dibutyltin dichloride in drinking water as adults. J. Immunotoxicol. 2:151–160.
- Duke, S. S. Schook, L. B., and Holsapple, M. P. 1985. Effects of N-nitrodimethylamine on tumor susceptibility. J. Leukocyte Biol. 37:383–394.
- Fair, P., Mitchum G., Hulsey T., Adams J., Zolman E., McFee W., Wirth E., and Bossart, G. 2007. Polybrominated diphenyl ethers (PBDEs) in blubber of free-ranging bottlenose dolphins (Tursiops truncatus) from two southeast Atlantic estuarine areas. Arch. Environ. Contam. Toxicol. 53:483–494.
- Faustman, E. M., and Omenn, G. S.. 2001. Risk assessment. In: Casarett and Doull’s Toxicology: The Basic Science of Poisons, 6th Edition. (Klaassen, C.D., Ed.), New York: McGraw-Hill Companies, Inc., pp.83–104.
- Fernie K. J., Shutt J. L., Mayne G., Hoffman D., Letcher, R. J., Drouillard K. G., and Ritchie I. J. 2005. Exposure to polybrominated diphenyl ethers (PBDEs): Changes in thyroid, Vitamin A, glutathione homeostasis, and oxidative stress in American kestrels (Falco sparverius). Toxicol. Sci. 88:375–383.
- Fernlöf G., Gadhasson I., Pödra K., Darnerud P. O., and Thuvander A. 1997. Lack of effects of some individual polybrominated diphenyl ether (PBDE) and polychlorinated biphenyl (PCB) congeners on human lymphocyte functions in vitro. Toxicol. Lett. 90:189–197.
- Fowles J. R., Fairbrother A., Baecher-Steppan L., and Kerkvliet N. I. 1994. Immunologic and endocrine effects of the flame-retardant pentabromodiphenyl ether (DE-71) in C57BL/6J mice. Toxicology 86:49–61.
- Fraser A., Webster, T., and McClean M. 2009. Diet contributes significantly to the body burden of PBDEs in the general U.S. population. Environ. Health Perspect. 117:1520–1525.
- Hakk H., and Letcher R. J. 2003. Metabolism in the toxicokinetics and fate of brominated flame retardants - a review. Environ. Int. 29:801–828.
- Hale, R. C., La Guardia, M. J., Harvey E., and Mainor, T. M. 2002. Potential role of fire retardant-treated polyurethane foam as a source of brominated diphenyl ethers to the U.S. environment. Chemosphere 46:729–735.
- Harper N., Howie L., Connor K., Dickerson R., and Safe S. 1993. Immunosuppressive effects of highly chlorinated biphenyls and diphenyl ethers on T-cell dependent and independent antigens in mice. Toxicology 85:123–135.
- Hillemeyer P., White M. D., and Pascual, D. W. 2002. Development of a transient CD4+CD8+ T-cell subset in the cervical lymph nodes following intratracheal instillation with an adenovirus vector. Cell. Immunol. 215:173–185.
- Holsapple, M. P., White, K. L., McCay J. A., Bradley S. G., and Munson A. E. 1988. An immunotoxicological evaluation of 4,4′-thiobis-(6-t-butyl-m-cresol) in female B6C3F1 mice. 2. Humoral and cell-mediated immunity, macrophage function, and host resistance. Fundam. Appl. Toxicol. 10:701–716.
- Jeffries, W. A., Green J. R., and Williams A. F. 1985. Authentic T-helper CD4 (W3/25) antigen on rat peritoneal macrophages. J. Exp. Med. 162:117–127.
- Jerne N. K., and Nordin A.A. 1963. Plaque formation in agar by single antibody-producing cells. Science 140:405.
- Johnson-Restrepo B., Kannan, K., Rapaport D. P., and Rodan B. D. 2005. Polybrominated diphenyl ethers and polychlorinated biphenyls in human adipose tissue from NY. Environ. Sci. Technol. 39:5177–5182.
- Kannan K., Yun S. H., Rudd, R. J., and Behr M. 2010. High concentrations of persistent organic pollutants including PCBs, DDT, PBDEs, and PFOS in little brown bats with white-nose syndrome in NY, USA. Chemosphere 80:613–618.
- Kitchen S. G., Whitmire J. K., Jones, N. R., Galic Z., Kitchen C. M. R., Ahmed R., and Zack, J. A. 2005. The CD4 molecule on CD8+ T-lymphocytes directly enhances the immune response to viral and cellular antigens. Proc. Natl. Acad. Sci. USA 102:3794–3799.
- Kodavanti, P. R. S., Coburn C. G., Moser V. C., MacPhail R. C., Fenton S. E., Stoker T. E., Rayner, J. L., Kannan K., and Birnbaum L. S. 2010. Developmental exposure to a commercial PBDE mixture, DE-71: Neurobehavioral, hormonal, and reproductive effects. Toxicol. Sci. 116:297–312.
- La Guardia, M. J., Hale R. C., and Harvey E. 2006. Detailed polybrominated diphenyl ether (PBDE) congener composition of the widely used penta-, octa-, and deca-PBDE technical flame-retardant mixtures. Environ. Sci. Technol. 40:6247–6254.
- Luster M. I., Munson A. E., Thomas P. T., Holsapple M. P., Fenters J. D., White K. L. Jr., Lauer, L. D., Germolec D. R., Rosenthal, G. J., and Dean J. H. 1988. Development of a testing battery to assess chemical-induced immunotoxicity: National Toxicology Program’s guidelines for immunotoxicity evaluation in mice. Fundam. Appl. Toxicol. 10:2–19.
- Luster M. I., Portier C., Pait, D. G., White K. L. Jr., Gennings, C., Munson A. E., and Rosenthal, G. J. 1992. Risk assessment in immunotoxicology. I. Sensitivity and predictability of immune tests. Fundam. Appl. Toxicol. 18:200–210.
- Mercado-Feliciano M., and Bigsby R. 2008. The polybrominated diphenyl ether mixture DE-71 is mildly estrogenic. Environ. Health. Persp. 116:605–611.
- Noren, K., and Meironyte D. 2000. Certain organochlorine and organobromine contaminants in Swedish human milk in perspective of past 20–30 years. Chemosphere 40:1111–1123.
- Olsen G. W., Burris J. M., Ehresman D. J., Foelich J. W., Seacat A. M., Butenhoff J. L., and Zobel L. R. 2007. Half-life of serum elimination of perfluorooctanesulfonate, perfluorohexanesulfonate, and perfluuorooctanoate in retired fluorochemical production workers. Environ. Health Perspect. 115:1298–1305.
- Peden-Adams M. M., Keller J. M., EuDaly J. G., Berger J., Gilkeson G. S., and Keil, D. E. 2008. Suppression of humoral immunity in mice following exposure to perfluorooctane sulfonate. Toxicol. Sci. 104:144–154.
- Periwal, S. B., and Cebra J.J. 1999. Respiratory mucosal immunization with reovirus serotype 1/L stimulates virus-specific humoral and cellular immune responses, including double-positive (CD4+/CD8+) T-cells. J. Virol. 73:7633–7640.
- Reimers, T. J., Cowan R. G., Davidson, H. P., and Colby E. D. 1981. Validation of radioimmunoassay for tri-iodothyronine, thyroxine, and hydrocortisone (cortisol) in canine, feline, and equine sera. Am. J. Vet. Res. 42:2016–2021.
- Sanders, J. M., Chen L. J., Lebetkin E. H., and Burka L. T. 2006. Metabolism and disposition of 2,2′,4,4′-tetra-bromodiphenyl ether following administration of single or multiple doses to rats and mice. Xenobiotica 36:103–117.
- Schecter A., Päpke O., Tung K. C., Joseph J., Harris T. R., and Dahlgren J. 2005. Polybrominated diphenyl ether flame retardants in the U.S. population: Current levels, temporal trends, and comparison with dioxins, dibenzofurans, and polychlorinated biphenyls. J. Occup. Environ. Med. 47:199–211.
- Selgrade M., Cooper, K. D., Devlin, R. B., van Loveren H., Baigini, R. E., and Luster M. I. 1995. Immunotoxicity - bridging the gap between animal research and human health effects. Fundam. Appl. Toxicol. 24:13–21.
- Shaw S. D., and Kannan K. 2009. Polybrominated diphenyl ethers in marine ecosystems of the American continents: Foresight from current knowledge. Rev. Environ. Health 24:157–229.
- Sjödin A., Jones R. S., Focant, J. F., Lapeza C., Wang R. Y., and McGahee E. E. III, 2004. Retrospective time-trend study of polybrominated diphenyl ether and polybrominated and polychlorinated biphenyl levels in human serum from the United States. Environ. Health Perspect. 112:654–658.
- Sjödin A., Lee-Yang Wong, L. Y., Jones, R. S., Park, A., Zhang Y., Hodge C., DiPietro E., McClure C., Turner W., Needham L. L., and Patterson D. G. Jr., 2008. Serum concentrations of polybrominated diphenyl ethers (PBDEs) and polybrominated biphenyl (PBB) in the United States population: 2003–2004. Environ. Sci. Technol. 42:1377–1384.
- Sjödin, A., Patterson D. G., and Bergman A.. 2003. A review on human exposure to brominated flame retardants - particularly polybrominated diphenyl ethers Environ. Intl. 29:829–839.
- Stapleton H., Dodder N. G., Kucklick J. R., Reddy C. M., Schantz M. M., Becker P. R., Gulland F., Porter B. J., and Wise S. A. 2006. Determination of HBCD, PBDEs and MeO-BDEs in California sea lions (Zalophus californianus) stranded between 1993 and 2003. Mar. Poll. Bull. 52:522–531.
- Stoker T. E., Laws, S. C., Crofton K. M., Hedge J. M., Ferrell J. M., and Cooper, R. L. 2004. Assessment of DE-71, a commercial poly-brominated diphenyl ether (PBDE) mixture, in the EDSP male and female pubertal protocols. Toxicol. Sci. 78:144–155.
- Swarthout R. F., Keller J. M., Peden-Adams, M., Landry A. M., Fair P. A., and Kucklick, J. R. 2010. Organohalogen contaminants in blood of Kemp’s Ridley (Lepidochelys kempii) and green sea turtles (Chelonia mydas) from the Gulf of Mexico. Chemosphere 78:731–741.
- Temple L., Kawabata T. T., Munson A. E., and White, K. L. 1993. Comparison of ELISA and plaque-forming cell assays for measuring the humoral immune response to SRBC in rats and mice treated with benzo[a]pyrene and cyclophosphamide. Fundam. Appl. Toxicol. 21:412–419.
- Teshima R., Nakamura R., Nakamura R., Hachisuka A., Sawada J., and Shibutani, M. 2008. Effects of exposure to decabromodiphenyl ether on the development of the immune system in rats. J. Health Sci. 54:382–389.
- Thuvander A., and Darnerud, P. O. 1999. Effects of polybrominated diphenyl ethers (PBDs) and polychlorinated biphenyl (PCB) on some immunological parameters after exposure in rats and mice. Toxicol. Environ. Chem. 70:229–242.
- Turyk M. E., Persky, V. W., Imm P., Knobeloch L., Chatterton R., and Anderson H. A. 2008. Hormone disruption by PBDEs in adult male sport fish consumers. Environ. Health Perspect. 116:1635–1641.
- Vonderheide A. P., Mueller K. E., Meija J., and Welsh G. L. 2008. Polybrominated diphenyl ethers: Causes for concern and knowledge gaps regarding environmental distribution, fate and toxicity. Sci. Total Environ. 400:425–436.
- Watanabe W., Shimizua T., Hinoa A., and Kurokawa K. 2008. Effects of decabrominated diphenyl ether (DBDE) on developmental immunotoxicity in offspring mice. Environ. Toxicol. Pharmacol. 26:315–319.
- Watanabe W., Shimizu T., Sawamura R., Hino A., Konno K., Hirose A., and Kurokawa M. 2010b. Effects of tetrabromobisphenol A, a brominated flame retardant, on the immune response to respiratory syncytial virus infection in mice. Int. Immunopharmacol. 10:393–397.
- Watanabe W., Shimizu T., Sawamura R., Hino A., Konno, K., and Kurokawa, M. 2010a. Functional disorder of primary immunity responding to respiratory syncytial virus infection in offspring mice exposed to a flame retardant, decabrominated diphenyl ether, perinatally. J. Med. Virol. 82:1075–1082.
- Windham G. C., Pinney S. M., Sjödin A., Lum, R., Jones R. S., Biro L. M., Hiatt R. A., Kushi L. H., and Needham F. 2010. Body burdens of brominated flame retardants and other persistent organo-halogenated compounds and their descriptors in U.S. girls. Environ. Res. 110:251–257.
- Williams, A. L., and DeSesso J. M. 2010. The potential of selected brominated flame retardants to affect neurological development. J. Toxicol. Environ. Health B 13:411–448.
- Wood, G. S., Warner N. L., and Warnke R. A. 1985. Anti-Leu-3/T4 antibodies react with cells of monocyte/macrophage and Langerhans lineage. J. Immunol. 131:212–216.
- Zhou T., Ross D. G., DeVito, M. J., and Crofton K. M. 2001. Effects of short-term in vivo exposure to polybrominated diphenyl ethers on thyroid hormones and hepatic enzyme activities in weanling rats. Toxicol. Sci. 61:76–82.
- Zuckermann F. A. 1999. Extrathymic CD4/CD8 double positive T-cells. Vet. Immunol. Immunopathol. 72:55–66.