Abstract
Welding fume inhalation affects the immune system of exposed workers. Manganese (Mn) in welding fume may induce immunosuppressive effects. The goal was to determine if Mn in welding fume alters immunity by reducing the number of circulating total leukocytes and specific leukocyte sub-populations. Sprague-Dawley rats were treated by intratracheal instillation (ITI) with either a single dose (2.00 mg/rat) or repeated doses (0.125 or 2.00 mg/rat for 7 weeks) with welding fumes that contained different levels of Mn. Additional rats were treated by ITI once a week for 7 weeks with the two doses of manganese chloride (MnCl2). Bronchoalveolar lavage was performed to assess lung inflammation. Also, whole blood was recovered, and the number of circulating total leukocytes, as well as specific lymphocyte subsets, was determined by flow cytometry. The welding fume highest in Mn content significantly increased lung inflammation, injury, and production of inflammatory cytokines and chemokines compared to all other treatment groups. In addition, the same group expressed significant decreases in the number of circulating CD4+ and CD8+ T-lymphocytes after a single exposure, and significant reductions in the number of circulating total lymphocytes, primarily CD4+ and CD8+ T-lymphocytes, after repeated exposures (compared to control values). Repeated MnCl2 exposure led to a trend of a reduction (but not statistically significant) in circulating total lymphocytes, attributable to the changes in the CD4+ T-lymphocyte population levels. The welding fume with the lower concentration of Mn had no significant effect on the numbers of blood lymphocytes and lymphocyte subsets compared to control values. Evidence from this study indicates that pulmonary exposure to certain welding fumes cause decrements in systemic immune cell populations, specifically circulating T-lymphocytes, and these alterations in immune cell number are not dependent exclusively on Mn, but likely a combination of other metals present in welding fume.
Introduction
Electric arc welding is a common industrial process used to join metals. Arc welding processes generate complex aerosols that are composed of potentially hazardous metals, such as iron (Fe), manganese (Mn), and chromium (Cr). Greater than 400,000 workers are classified as full-time welders in the US (Bureau of Labor Statistics, Citation2007), and several million more workers are regularly exposed to welding aerosols worldwide. The health effects of welders are oftentimes difficult to determine because welders are a heterogeneous workforce employed in a variety of workplace settings that may include confined, poorly ventilated spaces, or well-ventilated indoor and open-air sites. In addition, their exposure may vary due to the vast selection of different materials/processes commonly used in welding. Most long-time welders have experienced some type of pulmonary disorder during their career (as reviewed by Sferlazza and Beckett, Citation1991; Antonini, Citation2003). Respiratory effects have included bronchitis, metal fume fever, reversible lung function decrements, and a possible increase in the incidence of lung cancer. For decades, an area of concern within the occupational health community has been the observation that welding fume inhalation may cause decrements in immune function (as reviewed by Zeidler-Erdely et al., submitted). Worker studies assessing the adverse effects of welding fume exposure on immune function are limited.
Limited information is available about the systemic changes in leukocyte number after welding fume exposure. Kim et al. (Citation2005) observed a significant increase in peripheral leukocyte and neutrophil numbers in non-smoking welders immediately following an acute exposure. The oxidative stress ratio of peripheral blood monocytes (du Plessis et al., Citation2010) and biomarkers of systemic inflammation (Nuernberg et al., Citation2008) have been shown to be significantly elevated in workers exposed to welding fume compared to non-exposed controls. Hanovcova et al. (Citation1998) indicated that exposure to stainless steel welding fume increased the number of peripheral lymphocyte and lymphocyte subsets. However, Tuschl et al. (Citation1997) observed no change in circulating lymphocyte numbers in welders, but did report a significant decrement in the cytotoxic activity of natural killer (NK) cells.
Animal studies provide additional evidence of systemic immune responses. In a study by Rim et al. (Citation2004), gene expression profiling of peripheral blood mononuclear cells collected from rats after 30 days of inhalation to a relatively high concentration of stainless steel welding fume indicated that ~3-times more genes, many of which are associated with immune function and response, were down-regulated as opposed to being increased. Moreover, microarray technology and subsequent pathway analysis indicated a strong immunologic transcriptional response to welding fume in a study comparing lung tumor susceptible and resistant mouse strains (Zeidler-Erdely et al., Citation2010). Another study specifically evaluated the systemic humoral immune response after pulmonary exposure to a highly water-soluble stainless steel welding fume in rats (Anderson et al., Citation2007). In vitro exposure of splenocytes to stainless steel fume reduced IgM activity in response to sheep red blood cells (SRBC). In addition, splenocytes harvested after an in vivo exposure to 20 mg/kg of the stainless steel fume showed a significant decrease in the IgM response to SRBC.
Welding fume is a complex mixture of different metals, many of which may alter the host’s immune system after exposure. Nakata et al. (Citation2006) and Yuan et al. (Citation2006) hypothesized that Mn in welding fume may induce systemic immunosuppressive effects in exposed workers. Smialowicz et al. (Citation1988) indicated that manganese chloride (MnCl2) significantly enhanced systemic natural killer NK cell activity in young mice that was mediated by production of interferon (IFN)-α and -β. Mn is an essential element in most welding electrodes. Mn increases hardness and strength, prevents steel from cracking during manufacture, and improves metallurgical properties (Harris, Citation2002). Nakata et al. (Citation2006) showed that welders who had a high concentration of Mn in their blood displayed significant reductions in total lymphocytes, B-lymphocytes, total T-lymphocytes, and CD8+ T-lymphocytes compared to levels in workers with low blood Mn levels. In contrast, Yuan et al. (Citation2006) observed no differences in number of circulating total lymphocytes and lymphocyte subsets in welders who had elevated blood Mn levels compared to unexposed controls. It was the goal of the current study to develop an animal model to determine if pulmonary exposure to Mn in welding fume alters systemic immunity by causing changes in the number of circulating total lymphocytes and specific lymphocyte sub-populations.
Materials and methods
Experimental design
Male Sprague-Dawley rats were exposed (by intratracheal instillation [ITI]) to multiple doses of welding fumes that contained different levels of Mn. Short- and long-term dosing regimens included an acute, single ITI, or a repeated ITI regimen that lasted 7 weeks (once a week × 7 weeks). Additional sets of rats were treated by ITI once a week for 7 weeks with pure MnCl2. Whole blood was recovered from all treated animals, and the number of circulating total leukocytes, monocytes, polymorphonuclear leukocytes (PMN), and lymphocytes, as well as specific lymphocyte subsets, was determined by flow cytometry. Bronchoalveolar lavage (BAL) was performed only in the repeated dosing regimen study to assess onset/persistence of any lung injury and inflammation.
Welding fume characterization
Bulk samples of different welding fumes were collected by Lincoln Electric Co. (Cleveland, OH). The fumes were generated in a cubical open front fume chamber (volume = 1 m3) by a skilled welder using a manual or semi-automatic technique appropriate to the electrode and collected on 0.2 μm Nuclepore filters (Nuclepore Co., Pleasanton, CA). The fume samples were generated using two different processes: (1) gas metal arc welding using a mild steel E70S-3 electrode (GMA-MS; L-50 carbon steel electrode, Lincoln Electric Co., Cleveland, OH), and (2) shielded manual metal arc welding using a flux-covered stainless steel hard-surfacing electrode (MMA-HS; Wearshield 15CrMn, Lincoln Electric Co., Cleveland, OH). Particle sizes of bulk samples of the two collected fumes were determined using scanning electron microscopy and found to be in the respirable size range with count mean diameters of < 1.0 μm.
A portion of the collected fume samples was digested, and the metals were analyzed by inductively coupled plasma-atomic emission spectroscopy (ICP-AES) by the Division of Applied Research and Technology (DART, Cincinnati, OH), according to NIOSH method 7300 modified for microwave digestion (National Institute for Occupational Safety and Health, Citation1994). The metals that were measured included Ag, Al, As, Ba, Be, Ca, Cd, Co, Cr, Cu, Fe, K, La, Li, Mg, Mn, Mo, Na, Ni, P, Pb, Sb, Se, Sr, Te, Ti, Tl, V, Zn, and Zr. Metal content of blank filters were analyzed for control purposes.
Animals
Male Sprague-Dawley [Hla:(SD) CVF] rats from Hilltop Lab Animals (Scottsdale, PA), weighing 250–300 g and free of viral pathogens, parasites, mycoplasma, Helicobacter, and CAR Bacillus, were used for all exposures. The rats were acclimated for at least 6 days after arrival, and were housed in ventilated polycarbonate cages on Alpha-Dri cellulose chips and hardwood Beta-chips as bedding. All rats were provided HEPA-filtered air, and had ad libitum access to irradiated Harlan Teklad 2918 diet (Frederick, MD) and tap water. The animal facilities were specific pathogen-free, environmentally controlled (20–25°C, 30–40% relative humidity, and 12-h light/dark cycles), and accredited by the Association for Assessment and Accreditation of Laboratory Animal Care International (AAALAC). All animal procedures used during the study were reviewed and approved by the National Institute for Occupational Safety and Health (NIOSH) Animal Care and Use Committee.
Welding fume treatment
Two dosing regimens were used for the study: (1) single treatment—one intratracheal instillation (ITI) of 2.0 mg/rat of GMA-MS or MMA-HS welding fume; (2) repeated treatment—one ITI a week for 7 weeks, with 0.125 or 2.00 mg/rat of GMA-MS or MMA-HS welding fume. Additional sets of rats were treated by ITI once a week for 7 weeks with 0.125 or 2.00 mg/rat of manganese (II) chloride tetrahydrate (MnCl2; Sigma-Aldrich Chemical Co., St. Louis, MO). For the ITI procedure, rats were lightly anesthetized by an intraperitoneal injection of 0.6 ml of a 1% [w/v] solution of sodium methohexital (Brevital, Eli Lilly, Indianapolis, IN) and treated by ITI with the welding fume sample suspended in 300 μl of sterile phosphate-buffered saline (PBS, pH 7.4). Vehicle control animals were treated by ITI with 300 μl of sterile PBS.
Whole blood and bronchoalveolar lavage collection
At 1 day after the final ITI for each dosing regimen, animals were deeply anesthetized with an intraperitoneal injection of sodium pentobarbital (>100 mg/kg body weight [BW]; Sleepaway. Ft. Dodge Animal Health, Wyeth, Madison, NJ). Whole blood was collected via the inferior vena cava and each rat was exsanguinated (by severing abdominal aorta). BAL was performed on the animals used in the repeated dosing regimen at 1 day after the last of the seven weekly ITI treatments to assess lung injury and inflammation.
The lungs were first lavaged with a 1 ml/100 g BW aliquot of calcium-, magnesium-free PBS (pH 7.4). The first fraction of recovered bronchoalveolar lavage fluid (BALF) was centrifuged (500 ×g, 10 min), and the resultant cell-free supernatant analyzed for various biochemical parameters. The lungs were further lavaged with 6 ml aliquots of PBS until 30 ml were collected. These samples also were centrifuged (10 min, 500 × g), and the cell-free BALF discarded. The cell pellets from all washes for each rat were combined, washed, and re-suspended in 1 ml of PBS buffer and evaluated as described below.
Whole blood cell differentiation and quantification
Whole blood cells were differentiated and quantified as previously described (Erdely et al., Citation2011). Briefly, 100 μl of the blood cell suspension was added to a flow tube with 100 μl of 300 μg/ml mouse IgG (Sigma) in FACS buffer. After 10 min incubation, 50 μl of pre-mixed antibodies in FACS buffer were added to the tube and stained for 30 min at room temperature on a shaker. The antibody mixture contained 5 μg/ml of the following antibodies: CD45R-FITC (clone HIS24), CD4-FITC (clone OX35), CD8-PerCP (clone OX-8), and CD3-APC (clone 1F4). All antibodies were purchased from PharMingen (Becton Dickinson, San Diego, CA). Red blood cells were then lysed with 100 μl Caltag Cal-lyse lysing solution (GAS-010, Invitrogen, Carlsbad, CA) for 10 min in the dark. Caltag counting beads (PCB-100, Invitrogen) were added for cell enumeration prior to analysis using a FACSCalibur flow cytometer (Becton Dickinson Biosystems, San Jose, CA). Samples were acquired through a live gate without compensation.
After collecting 3500 counting beads, the data of all cells were exported to the analysis software FlowJo (Treestar, Costa Mesa, CA). Leukocytes were separated by side scattering (SSC) and forward scattering (FSC) into three gates: lymphocytes, monocytes, and eosinophils + neutrophils. The lymphocytes were further classified by FSC/SSC and specific surface markers. CD4 cells were identified by expression of CD3+ and CD4+; CD8 cells were identified as double positive in CD3+ and CD8+ channels. B-Lymphocytes were distinguished from T-lymphocytes by CD45R expression in the lymphocyte gate. Natural killer (NK) cells were identified by expression of NKR-PIA, but not CD3, marker. NK-T cells were identified as double positives in CD3 vs NKR-PIA plots. The NK antibody staining was needed for the calculation of the total CD8+ population.
Lung injury and inflammation
Lung cell differentiation
Total cell numbers recovered by BAL were determined using Coulter Multisizer II and AccuComp software (Coulter Electronics, Hialeah, FL). Cells were prepared on cytospin slides (5 × 104 cells) using a Cytospin 3 centrifuge (Shandon Life Sciences International, Cheshire, UK). Cells (200/rat) were identified after labeling with Leukostat stain (Fisher Scientific, Pittsburgh, PA) as alveolar macrophages, polymorphonuclear leukocytes (PMN), and lymphocytes.
Biochemical parameters of lung injury
Using the acellular first fraction of BALF, albumin content (index to quantify increased permeability of bronchoalveolar-capillary barrier) and lactate dehydrogenase (LDH) activity (indicator of general cytotoxicity) were measured. Albumin content was determined colorimetrically at 628 nm (based on albumin binding to bromcresol green) using an albumin BCG diagnostic kit (Sigma). LDH activity was determined by measuring oxidation of lactate to pyruvate coupled with the formation of NADH at 340 nm. Measurements were performed with a COBAS MIRA auto-analyzer (Roche Diagnostic Systems, Montclair, NJ).
BALF cytokine and chemokine determination
Levels of cytokines, tumor necrosis factor (TNF)-α, interleukin (IL)-1β, -2, -6, and -10, and the chemokines monocyte chemotactic protein-1 (MCP-1) and macrophage inflammatory protein-2 (MIP-2) were assayed in the first fraction of BALF. The selection of the cytokines and/or chemokines was based on their role in lung inflammatory and immune responses after particulate exposure. Cyto-/chemokine protein concentrations were determined using enzyme-linked immunoabsorbent assay (ELISA) kits (Biosource International, Inc., Camarillo, CA). Results of the colorimetric assay were obtained with a Spectramax 250 plate spectrophotometer using Softmax Pro 2.6 software (Molecular Devices Corp., Sunnyvale, CA).
Serum and BALF antibody quantification
The concentrations of rat immunoglobulins (IgA, IgG, and IgM) and complement factor 3 (C3) were determined in the serum and/or first fraction BALF by ELISA (GenWay Biotech, Inc., San Diego, CA) according to manufacturer’s instructions. The Spectramax 250 plate reader was used to measure absorbance values for each immunoasssay.
Statistical analysis
All data are presented as mean [± SE]. Analyses were performed using JMP® 9 Statistical Discovery Software (SAS, Cary, NC). Treatments were compared by a one-way Analysis of Variance (ANOVA) followed by a post-hoc Student’s t-test. Differences were considered statistically significant at p < 0.05.
Results
Fume characterization
Metal composition of the two welding fume samples () was determined in a previous study (Antonini et al., Citation2010). Briefly, the GMA-MS fume was relatively insoluble in water and composed of Fe and Mn. The MMA-HS fume was significantly more water-soluble, and composed of predominately Mn, Fe, and Cr and alkali metals, potassium (K), and sodium (Na). The percentage of total Mn (50.90%) was more than double in the MMA-HS fume compared to the Mn (21.70%) in GMA-MS.
Table 1. Metal composition and solubility properties of welding fume samples.
Systemic immune cell response; single welding fume treatment
Total leukocytes, monocytes, PMN, and lymphocytes, as well as lymphocyte subsets were determined in whole blood collected 1 day after a single 2.00 mg dose of the two different welding fumes (). A single intratracheal treatment with the two fumes had no significant effect on the number of total circulating leukocytes, monocytes, and lymphocytes. Blood PMN levels were significantly elevated 1 day after a single treatment with both the GMA-MS and MMA-HS welding fumes, compared to control values. Among the lymphocyte subsets, CD4+ and CD8+ T-lymphocyte levels were significantly reduced below control values 1 day after a single treatment with the MMA-HS fume, but not the GMA-MS welding fume. B-Lymphocyte numbers in the blood were unchanged after a single treatment with either of the two fumes.
Table 2. Immune cell differential of whole blood after a single welding fume treatment.
Local lung response; repeated welding fume treatment
Multiple parameters of lung injury and inflammation were measured in the recovered BALF 1 day after 7 weeks of treatment with two doses of the welding fumes and MnCl2 groups (). In terms of lung injury, repeated lung treatment with each welding fume and the MnCl2 groups at the two doses significantly increased both LDH and albumin, compared to control host values. Treatment with the 2.00 mg dose of the MMA-HS and MnCl2 groups caused significant increases in both LDH and albumin compared to values seen in the other welding fume groups.
Table 3. Lung injury and inflammation in BALF after repeated welding fume treatments.
In the assessment of lung inflammation, repeated treatment with all of the welding fume and MnCl2 groups caused significant elevations in the number of PMN and lymphocytes recovered from the lungs by BAL. Both BALF PMN and lymphocyte numbers were significantly increased after repeated treatment with 2.00 mg of the MMA-HS and MnCl2 groups compared to values seen in the other welding fume groups (). Both MnCl2 groups and only the 2.00 mg dose for the two fume groups caused a significant increase (compared to control) in BALF alveolar macrophage number and MIP-2 levels. BALF MIP-2, MCP-1, TNFα, and IL-6 levels were significantly elevated in the 2.00 mg MMA-HS and MnCl2 groups compared to values noted with rats in all the other groups. Treatment with the welding fumes and MnCl2 had no significant effect on BALF IL-1β, IL-2, or IL-10 levels. Both doses of the MMA-HS and MnCl2 groups caused a significant increase in BALF IgG levels after repeated treatment compared to control.
Systemic immune cell response; repeated welding fume and MnCl2 treatment
Total leukocytes, monocytes, PMN, and lymphocytes were determined in whole blood collected 1 day after 7 weeks of treatment with two doses of the different welding fumes and MnCl2 groups (). Repeated welding fume treatment had no statistically significant effect on the number of circulating total leukocytes and monocytes ( and ). Both doses of MMA-HS and the 2.00 mg dose of MnCl2 resulted in significantly increased circulating PMN compared to saline control (), indicating the capacity of these groups to induce systemic inflammation. Intratracheal treatment for 7 weeks with 2.00 mg of the MMA-HS fume caused a significant decrease in circulating total lymphocytes compared to saline control values (). No effect on total lymphocyte number was found in the other fume or MnCl2 groups (compared to control).
Figure 1. Systemic response; repeated treatment. Total (a) leukocytes, (b) monocytes, (c) PMN, and (d) lymphocytes in whole blood at 1 day after repeated intratracheal instillations of 0.125 or 2.0 mg of gas metal arc-mild steel (GMA-MS) and manual metal arc–hard-surfacing (MMA-HS) welding fume or MnCl2 once a week for 7 weeks. Control animals were treated with sterile saline. Values are means ± SE (n = 5–6). * Value significantly different from saline control group for a particular cell type (p < 0.05).
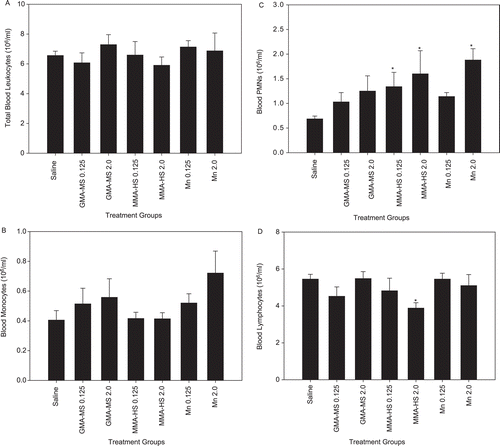
Subsets of lymphocytes were also measured in whole blood after repeated treatment with the two different welding fumes and MnCl2 groups (). The number of circulating B-lymphocytes was unaffected by welding fume and MnCl2 treatment (). T-Lymphocyte, CD4+, and CD8+ cell levels were significantly decreased after repeated treatment with 2.00 mg of MMA-HS fume as compared to values noted in the saline control hosts (). None of the other welding fume or MnCl2 groups demonstrated a significant effect from treatments on T lymphocytes, CD4+, and CD8+ cell levels relative to those associated with the saline controls.
Figure 2. Systemic response; repeated treatment. (a) B-lymphocytes, (b) T-lymphocytes, (c) CD4+ lymphocytes, and (d) CD8+ lymphocytes in whole blood at 1 day after repeated intratracheal instillations of 0.125 or 2.0 mg of gas metal arc-mild steel (GMA-MS) and manual metal arc-hard-surfacing (MMA-HS) welding fume or MnCl2 once a week for 7 weeks. Control animals were treated with sterile saline. Values are means ± SE (n = 5–6). * Value significantly different from saline control group for a particular cell type (p < 0.05).
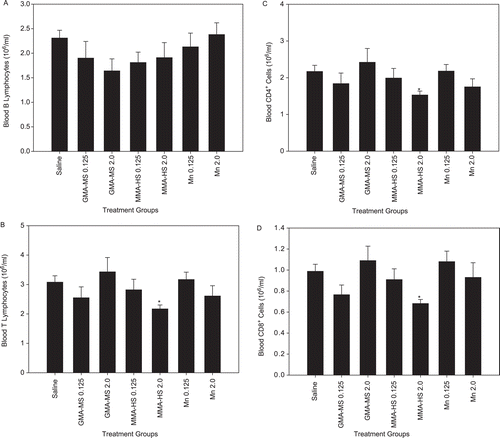
Different immunoglobulins were measured in the serum after repeated welding fume treatment. None of the welding fume treatments had a statistically significant effect on serum concentrations of IgG, IgA, IgM, or C3 compared to control (data not shown).
Discussion
In agreement with several worker studies, systemic PMN numbers were increased in most cases after welding fume treatment in the current animal study. In terms of circulating lymphocyte number, the high Mn, MMA-HS fume had the greatest effect on the systemic immune T-lymphocyte differential, whereas neither dose of the low Mn, GMA-MS fume caused a significant change in the number of circulating total T-lymphocytes or T-lymphocyte sub-populations. Significant reductions in circulating total T-lymphocytes and lymphocyte subsets were observed after treatment with 2.00 mg of MMA-HS fume. The response was similar, regardless of whether the animals were treated with a single or multiple treatments with 2.00 mg of MMA-HS fume, indicating that the effect was acute and persisted over time. This reduction in the number of circulating lymphocytes may be attributed partly to increased translocation of the cells to the alveolar space () or lung-associated lymph nodes. Previously, Anderson et al. (Citation2007) observed a significant increase in the total number of both B- and T-lymphocytes in the lung-associated lymph nodes after lung treatment with welding fume. Interestingly, no changes in the number of whole blood B-lymphocytes were observed after treatment with the MMA-HS fume.
The effect on blood T-lymphocytes observed in the current study was mostly supported by Nakata et al. (Citation2006), who showed that the number of total lymphocytes, total T-lymphocytes, and CD8+ T-lymphocytes were significantly lower in welders that had higher blood Mn concentrations compared to workers with lower blood Mn levels. In contrast to the Nakata et al. (Citation2006) study, Yuan et al. (Citation2006) observed no significant difference in the number of peripheral T-lymphocytes and T-lymphocyte subsets when comparing Mn-exposed welders with non-exposed controls. Even though mean blood Mn levels for the exposed welder group in both studies were comparable, i.e., 1.7 mg/dl (Nakata et al., Citation2006) vs 4.8 mg/dl (Yuan et al., Citation2006), the average workplace Mn exposure concentration for the Nakata et al. (35.7 mg/m3 in one factory and 4.80 mg/m3 in the second factory) study was substantially higher compared to the Mn levels measured in the Yuan et al. study (i.e., 0.138 mg/m3) study. The difference in airborne Mn exposure concentration, and ultimately the pulmonary exposure of the worker, and not blood Mn level, may better explain the varied systemic cell response observed in the two studies. This observation provides additional evidence that blood Mn concentrations may be a poor correlate for workplace exposure to Mn (Ellingsen et al, Citation2003; Lu et al., Citation2005; Dorman et al., Citation2008). Another possible explanation of the observed difference from the Nakata et al. (Citation2006) and Yuan et al. (Citation2006) studies was that Yuan et al. (Citation2006) used the percentage of lymphocytes for each subset, whereas Nakata et al. (Citation2006) utilized the absolute counts of the lymphocyte subset.
The effect of welding fume exposure on circulating immunoglobulin levels has been demonstrated to be variable in worker studies. Borska et al. (Citation2003) observed significant reductions in serum IgG, IgA, and IgM and a significant increase in serum C3 in welders compared to a non-exposed control group, whereas Boshnakova et al. (Citation1989) observed only a significant decrease in serum IgG in welders, but no changes in serum IgA and IgM. Tuschl et al. (Citation1997) observed no differences in circulating IgG, IgA, and IgM when comparing welders and non-welders. In the current animal study, none of the welding fume treatments caused significant changes in the serum concentrations of IgG, IgA, IgM, or C3. In agreement, Nakata et al. (Citation2006) also observed no change in circulating levels of IgG, IgA, and IgM when comparing groups of workers exposed to varying concentrations of Mn in welding fume. On the other hand, IgG was significantly increased in the BALF after treatment with both doses of the MMA-HS fume in the current study. This increase in IgG in the BALF was likely the result of translocation of IgG from the circulation to the airspaces as another serum protein, albumin, was also significantly elevated in the BALF after treatment with the MMA-HS fume.
Even at relatively high repeated doses (~2-fold higher than Mn amount in the MMA-HS fume), pure MnCl2 did not cause a significant decrease in circulating T-lymphocytes in the current study, although there was a trend for reduced numbers of total T-lymphocytes, primarily CD4+ T-lymphocytes. Thus, the influence of other metals present in welding fume on the immune system cannot be ignored, and these other metals may account for the observation that MnCl2 only showed a trend for decreased T-lymphocytes as compared to the total MMA-HS fume. It has been hypothesized that Fe, the primary component of most welding fume, may pre-dispose welders and other metal workers to infectious pneumonia (Palmer et al., Citation2003, 2006). It has been proposed that free Fe may serve as a nutrient for infectious micro-organisms and promote their growth and viability (Ghio and Cohen, Citation2005; Weinberg, Citation2009). However, a previous animal study has shown that ITI of iron oxide (Fe2O3) had no effect on local immune defense responses, and did not alter the clearance of a bacterial pathogen (Antonini and Roberts, Citation2007).
The immunosuppressive effects of Cr have been well-documented (Cohen et al., Citation1998; Cohen, Citation2004). Measureable levels of soluble Cr were observed in the MMA-HS fume used in the current study. Previous studies have shown that lung treatment with pure soluble Cr at concentrations found in welding fume or stainless steel welding fume that contain soluble Cr before pulmonary infection with a bacterial pathogen, Listeria monocytogenes, significantly increased bacterial lung burden when compared to control (Antonini et al., Citation2004, Citation2007; Antonini and Roberts, Citation2007). Importantly, the amount of Cr (8.5%) in the MMA-HS fume used in the current study was substantially less compared to the amount of Cr in the fumes, i.e., 29% and 20%, used in, respectively, Antonini et al. (Citation2004) and Antonini et al. (Citation2007). Even though Cr levels were much lower and Mn levels were substantially higher in the MMA-HS fume used in the current study, the systemic T-lymphocyte and T-lymphocyte subset response induced by the MMA-HS fume likely was not based solely on the influence of one metal, but a combination of metals. It could be hypothesized that the effect on systemic T-lymphocyte populations may be the result of an additive or synergistic interaction between the Cr and Mn in the welding fume. A similar observation was made in an earlier study that showed oxidant-induced lung injury caused by welding fume was not dependent exclusively on a single metal or the solubility of the metal (Taylor et al., Citation2003).
In summary, an animal model was established that examined the effect of pulmonary exposure to welding fumes that contained different levels of Mn on the systemic immune cell profile. The welding fume with the highest concentration of Mn caused significant decreases in the number of circulating T-lymphocytes and specific T-lymphocyte subsets after acute and repeated lung treatment. None of the welding fume treatments used in the study caused significant changes in serum concentrations of IgG, IgA, IgM, or C3. Importantly, a high repeated pulmonary dose of MnCl2 did not cause a significant decrease in circulating T-lymphocytes and lymphocyte subsets, indicating that the immunomodulatory effects observed in welders are likely due to pulmonary exposure to a combination of metals.
Notice of correction:
This paper published online on 28 Februrary 2012 was incorrectly titled as a REVIEW ARTICLE. It has been corrected to a RESEARCH ARTICLE.
Disclaimer
The findings and conclusions of this paper have not been formally disseminated by NIOSH and should not be construed to represent any agency determination or policy.
Declaration of interest
The authors report no conflicts of interest. The authors alone are responsible for the content and writing of the paper.
References
- Anderson, S. E., Meade, B. J., Butterworth, L. F., and Munson, A. E. 2007. The humoral immune response of mice exposed to manual metal arc stainless steel-welding fumes. J. Immunotoxicol. 4:15–23.
- Antonini, J. M. 2003. Health effects of welding. Crit. Rev. Toxicol. 33:61–203.
- Antonini, J. M., and Roberts, J. R. 2007. Chromium in stainless steel welding fume suppresses lung defense responses against bacterial infection in rats. J. Immunotoxicol. 4:117–127.
- Antonini, J. M., Roberts, J. R., Chapman, R., Soukup, J. M., Ghio, A. J., and Sriram, K. 2010. Pulmonary toxicity and extra-pulmonary tissue distribution of metals after repeated exposure to different welding fumes. Inhal. Toxicol. 22:805–816.
- Antonini, J. M., Stone, S., Roberts, J. R., Chen, B., Schwegler-Berry, D., Afshari, A., and Frazer, D. G. 2007. Effect of short-term stainless steel welding fume inhalation exposure on lung inflammation, injury, and defense responses in rats. Toxicol. Appl. Pharmacol. 223:234–245.
- Antonini, J. M., Taylor, M. D., Millecchia, L., Bebout, A. R., and Roberts, J. R. 2004. Suppres-sion in lung defense responses after bacterial infection in rats pretreated with different welding fumes. Toxicol. Appl. Pharmacol. 200:206–218.
- Borska, L., Fiala, Z., Andrys, C., Krejsek, J., and Tejrat, J. 2003. Health risks of occupational exposure in welding processes. II. Immunological effects. Acta Med. (Hradec Kralove) 46:31–35.
- Boshnakova, E., Divanyan, S., Zlatarov, I., Marovsky, S. V., Kisyova, K., Zanev, D., Karev, G., and Marinova, T. Z. 1989. Immunological screening of welders. J. Hyg. Epidemiol. Microbiol. Immunol. 33:379–382.
- BLS. Bureau of Labor Statistics. 2007. Occupational Employment Statistics: Occupational Employment and Wages, 2007, Welders, Cutter, Solders, and Brazers, Washington, D.C.: U.S. Department of Labor. http://www.bls.gov/oes/current/oes514121.htm.
- Cohen, M. D. 2004. Pulmonary immunotoxicology of select metals: Aluminum, arsenic, cad-mium, chromium, copper, manganese, nickel, vanadium, and zinc. J. Immunotoxicol. 1:39–69.
- Cohen, M. D., Zelikoff, J. T., Chen, L. C., and Schlesinger, R. B. 1998. Immunotoxicological effects of inhaled chromium: Role of particle solubility and co-exposure to ozone. Toxicol. Appl. Pharmacol. 152:30–40.
- Dorman, D. C., Struve, M. F., Norris, A., and Higgins, A. J. 2008. Metabolomic analyses of body fluids after subchronic manganese inhalation in rhesus monkeys. Toxicol. Sci. 106:46–54.
- du Plessis, L., Laubscher, P., Jooste, J., du Plessis, J., Franken, A., van Aarde, N., and Eloff, F. 2010. Flow cytometric analysis of the oxidative status in human peripheral blood mononuclear cells of workers exposed to welding fumes. J. Occup. Environ. Hyg. 7:367–374.
- Ellingsen, D. G., Hetland, S. M., and Thomassen, Y. 2003. Manganese air exposure assessment and biological monitoring in the manganese alloy production industry. J. Environ. Monit. 5:84–90.
- Erdely, A., Liston, A., Salmen-Muniz, R., Hulderman, T., Young, S. H., Zeidler-Erdely, P. C., Castranova, V., and Simeonova, P. P. 2011. Identification of systemic markers from a pulmonary carbon nanotube exposure. J. Occup. Environ. Med. 53:S80–S86.
- Ghio, A. J., and Cohen, M. D. 2005. Disruption of iron homeostasis as a mechanism of biologic effect by ambient air pollution particles. Inhal. Toxicol. 17:709–716.
- Hanovcova, I., Chylkova, V., Tejral, J., Andrys, C., Prochazkova, J., Turkova, M., Srb, V., Ettlerova, E., and Kokes, J. 1998. Long-term monitoring of the immune reactivity of stainless steel welders. Centre Eur. Publ. Health 6:51–56.
- Harris, M. K. 2002. Fume and gas generation. In: Welding Health and Safety: A Field Guide for OEHS Professionals. Fairfax, VA: American Industrial Hygiene Association, pp. 214.
- Kim, J. Y., Chen, J. C., Boyce, P. D., and Christiani, D.C. 2005 Exposure to welding fumes is associated with acute systemic inflammatory responses. Occup. Environ. Med. 62:157–163.
- Lu, L., Zhang, L. L., Li, G. J., Guo, W., Liang, W., and Zheng, W. 2005. Alteration of serum concentrations of manganese, iron, ferritin, and transferrin receptor following exposure to welding fumes among career welders. Neurotoxicology 26:257–265.
- Nakata, A., Araki, S., Park, S. H., Park, J. T., Kim, D. S., Park, H. C., and Yokoyama, K. 2006. Decreases in CD8+ T-, naïve (CD45+CD45RA+) T-, and B (CD19+)-lymphocytes by exposure to manganese fume. Ind. Health 44:592–597.
- National Institute for Occupational Safety and Health (NIOSH). 1994. Elements (ICP): Method 7300. In: NIOSH Manual of Analytical Methods (U.S. Department of Health and Human Services, Ed.), 4th Edition, Issue 2, Publication No. 98-119, Washington, DC: NIOSH. Pages 1–8.
- Nuernberg, A. M., Boyce, P. D., Cavallari, J. M., Fang, S. C., Eisen, E. A., and Christiani, D. C. 2008. Urinary 8-isoprostane and 8-OHdG concentrations in boilermakers with welding exposure. J. Occup. Environ. Med. 50:182–189.
- Palmer, K. T., McNeill-Love, R., Poole, J. R., Coggon, D., Frew, A. J., Linaker, C. H., and Shute, J. K. 2006. Inflammatory responses to occupational inhalation of metal fume. Eur. Respir. J. 27:366–373.
- Palmer, K. T., Poole, J., Ayres, J. G., Mann, J., Burge, P. S., and Coggon, D. 2003. Exposure to metal fume and infectious disease. Am. J. Epidemiol. 157:227–233.
- Rim, K. T., Park, K. K., Sung, J. H., Chung, Y. H., Han, J. H., Cho, K. S., Kim, K. J., and Yu, I. J. 2004. Gene-expression profiling using suppression-subtractive hybridization and cDNA microarray in rat mononuclear cells in response to welding-fume exposure. Toxicol. Ind. Health 20:77–88.
- Sferlazza, S. J., and Beckett, W. S. 1991. The respiratory health of welders. Am. Rev. Respir. Dis. 143:1134–1148.
- Smialowicz, R. J., Riddle, M. M., Rogers, R. R., Luebke, R. W., and Burleson G. R. 1988. Immunopharmcol. Immunotoxicol. 10:93–107.
- Taylor, M. D., Roberts, J. R., Leonard, S. S., Shi, X., and Antonini, J. M. 2003. Effects of welding fumes of differing composition and solubility on free radical production and acute lung injury and inflammation in rats. Toxicol. Sci. 75:181–191.
- Tuschl, H., Weber, E., and Kovac, R. 1997. Investigations on immune parameters in welders. J. Appl. Toxicol. 17:377–383.
- Weinberg, E. D. 2009. Iron availability and infection. Biochim. Biophysc. Acta 1790:600–605.
- Yuan, H., He, S., He, M., Niu, Q., Wang, L., and Wang, S. 2006. A comprehensive study on neurobehavior, neurotransmitters, and lymphocyte subsets alteration of Chinese manganese welding workers. Life Sci. 78:1324–1328.
- Zeidler-Erdely, P. C., Erdely, A., and Antonini, J. M. 2011. Immunotoxicology of arc welding fumes: A review of worker and experimental animal studies. J. Immunotoxicol. (submitted).
- Zeidler-Erdely, P. C., Kashon, M. L., Li, S., and Antonini, J. M. 2010. Response of the mouse lung transcriptome to welding fume: Effects of stainless and mild steel welding fumes on lung gene expression in A/J and C57BL/6J mice. Resp. Res 11:(70):1–18.