Abstract
Arc welding processes generate complex aerosols composed of potentially hazardous metal fumes and gases. Millions of workers worldwide are exposed to welding aerosols daily. A health effect of welding that is of concern to the occupational health community is the development of immune system dysfunction. Increased severity, frequency, and duration of upper and lower respiratory tract infections have been reported among welders. Specifically, multiple studies have observed an excess mortality from pneumonia in welders and workers exposed to metal fumes. Although several welder cohort and experimental animal studies investigating the adverse effects of welding fume exposure on immune function have been performed, the potential mechanisms responsible for these effects are limited. The objective of this report was to review both human and animal studies that have examined the effect of welding fume pulmonary exposure on local and systemic immune responses.
Abbreviations: | ||
Mn, | = | manganese |
Cr6+, | = | hexavalent chromium |
Ni, | = | nickel |
CO, | = | carbon monoxide |
O3, | = | ozone |
MMA, | = | shielded manual metal arc welding |
GMA, | = | gas metal arc welding |
FCA, | = | flux-cored arc welding |
MIG, | = | metal inert gas welding |
TIG, | = | tungsten inert gas welding |
NO, | = | nitrogen oxide |
CO2, | = | carbon dioxide |
Cr, | = | chromium |
Fe, | = | iron |
MS, | = | mild steel |
SS, | = | stainless steel |
Al, | = | aluminium |
ACGIH, | = | American Conference of Governmental Industrial Hygienists |
TLV-TWA, | = | Threshold Limit Value-Time Weighted Average |
CRP, | = | C reactive protein |
NK, | = | natural killer cells |
IgA, | = | immunoglobulin A |
IgG, | = | immunoglobulin G |
IgM, | = | immunoglobulin M |
ROS, | = | reactive oxygen species |
BALF, | = | bronchoalveolar lavage fluid |
ITI, | = | intratracheal instillation |
AM, | = | alveolar macrophage |
PMN, | = | polymorphonculear leukocyte |
Mmp12, | = | matrix metalloproteinase-12 |
Cd5l, | = | Cd 5 molecule-like |
Ccl7, | = | chemokine (C-C motif) ligand 7 |
Cxcl5, | = | C-X-C motif chemokine 5 |
Spp1, | = | secreted phosphoprotein 1 |
Trem2, | = | triggering receptor expressed on myeloid cells 2 |
IgG-2a, | = | gamma-2a immunoglobulin heavy chain |
Igh-1a, | = | immunoglobulin heavy chain-1a |
Igh, | = | immunoglobulin heavy locus |
Ccl3 | = | and Ccl4, chemokine (C-C motif) ligands 3 and 4 |
TH1, | = | T-helper type 1; TH2, T-helper type 2 |
IFN-γ, | = | interferon-γ |
TNF, | = | tumor necrosis factor |
IL, | = | interleukin |
MCP-1, | = | monocyte chemotactic protein-1 |
MIP-2, | = | macrophage inflammatory protein-2 |
SRBC, | = | sheep red blood cells |
LPS, | = | lipopolysaccharide |
Fe2O3, | = | iron oxide |
Introduction
A scientific panel reported increased severity, frequency, and duration of upper and lower respiratory tract infections among welders (Lockey et al., Citation1988). An increased mortality from pneumonia has been observed in welders and metal fume workers (Coggon et al., Citation1994; Hoffmaster et al., Citation2006; Avashia et al., Citation2007). Evidence suggests that ferrous and other metal fumes inhaled in the workplace may predispose workers to pulmonary infection (Palmer et al., Citation2003). Unfortunately, elucidation of the mechanisms that cause these observations is lacking. The objective of this manuscript was to review both human and animal studies that have examined the effect of welding fume pulmonary exposure on local and systemic immune responses. Following a summary of what is known about welding processes and the physico-chemical characteristics of commonly used welding particles, the review will delve into the health effects from occupational exposure of humans followed by a summary of what is known about immunotoxic effects/mechanisms of effect gleaned from animal model studies.
Welding processes
Arc welding is a common industrial process used to join metals. Arc temperatures above 4000°C heat the base metal pieces to be joined and the consumable electrode wire or rod that is continuously fed into the weld. Most of the generated fume is derived from the consumable electrode, which is partially volatilized in the welding process (Palmer and Eaton, Citation2001). Vaporized metals react with air, producing metal oxide particles that are primarily of respirable size. Arc welding processes generate complex aerosols that are composed of hazardous metal fumes (e.g., manganese (Mn), hexavalent chromium (Cr6+), nickel (Ni)), and gases (e.g., carbon monoxide (CO), ozone (O3)).
The American Welding Society has identified more than 17 metal joining processes that are used commercially (The James F. Lincoln Arc Welding Foundation. Citation2000). Seven processes are classified under arc welding that together account for the greatest amount of welding and filler metal deposited commercially. Each arc welding method has its own metallurgical and operational advantages, and may present its own potential health and safety hazard. The most common types of welding are shielded-manual metal arc (MMA), gas metal arc (GMA), flux-cored arc (FCA), gas tungsten arc, and submerged arc welding (). The composition and the rate of generation of welding fumes are characteristic of the individual welding processes, and may be affected by the welding current, shielding gases, and the technique and skill of the welder. The choice of a particular technology depends on many factors, including the thickness and type of base metal to be welded, the size and strength of the weld desired, the speed or volume of welding, the position of the material to be welded (i.e., vertical or horizontal), and cost (Martin et al., Citation1997). Exposure to diverse aerosols generated from different welding processes, combined with the complexity of workplace settings, could increase the risk of exposure and the associated adverse health effects.
Table 1. Common arc welding processes and descriptions.
Welding fume characterization
Particle morphology and size
Electron microscopic analyses () have revealed that most particles generated during arc welding processes are arranged as chain-line agglomerates of smaller, spherical primary particles (Zimmer and Biswas, Citation2001; Jenkins et al., Citation2005; Antonini et al., Citation2006). Particle formation during arc welding first includes nucleation, a process by which high temperature metal vapors are transformed into primary particles, followed by coagulation, a dynamic aerosol growth process where smaller primary particles collide to form larger agglomerates (Zimmer, Citation2002). The primary particles generated during arc welding have been observed to be in the ultrafine size range, <0.10 µm (Sowards et al., Citation2010). Modest changes in welding process parameters (e.g., voltage) can affect the number of ultrafine welding particles formed (Antonini et al., Citation2011a). The agglomerates are formed after collisions between primary particle–primary particle, primary particle–agglomerate, and agglomerate–agglomerate (Zimmer, Citation2002). After these collisions, the agglomerates are believed held together by van der Waals, electrostatic, and magnetic forces. The mass median aerodynamic diameter of most arc welding fume is in the fine size range between 0.20–0.50 µm after reaching the welder’s breathing zone.
Figure 1. Scanning electron micrograph of SS welding fume collected onto a filter during welding using a flux-cored electrode. Note the chain-like agglomerates of much smaller, spherical primary particles. Welding fume samples were collected onto 47-mm Nuclepore polycarbonate filters (Whatman, Clinton, PA). The filters were cut into equal sections and mounted onto aluminum stubs with silver paste. The deposited welding particles were viewed using a JEOL 6400 scanning electron microscope (JEOL, Inc., Tokyo, Japan).
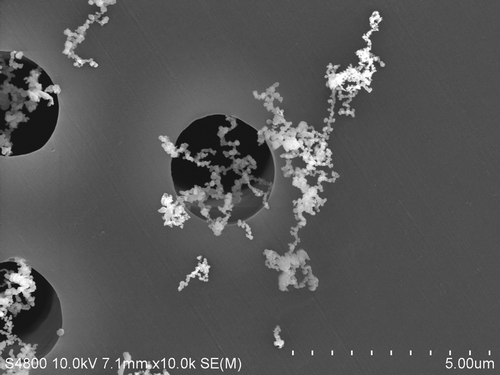
Gas and fume chemical properties
A variety of gases are formed during the welding process that may affect the health of exposed workers (). Weld shielding gases can intensify the ultraviolet radiation produced in the arc, leading to the photochemical formation of O3 and nitrogen oxides (NO, NO2). Carbon dioxide (CO2) may be reduced and converted to the chemically more stable, but potentially fatal gas, CO. The oxidation of vapors from degreasing agents (e.g., trichloroethylene), which are sometimes used to clean the base metals prior to welding, can produce the pulmonary irritant gas, phosgene.
Table 2. Potential health hazards associated with welding.
In addition to gases, arc welding produces complex metal oxide particles that are volatilized from the consumption of the welding electrode or the flux material added within or on the outside of an electrode (Zimmer and Biswas, Citation2001). Shielding gases; base metal composition and coating; paint; the presence of degreasing, cleaning, or anti-slag chemicals; and welding process parameters may all influence the chemical composition of the resulting fume. Most welding jobs use mild or carbon and low alloy steels. Mild steel (MS) electrodes are composed predominately of iron (Fe) with low and varying amounts of Mn. Welding using stainless steel (SS), aluminum (Al), Ni, and other alloys accounts for < 10% of all welding performed (Beckett, Citation1996). SS electrodes contain significant amounts of Cr, in addition to Fe, Mn, and Ni. Depending on the welding metals and processes used, oxides and salts of many other elements may be present in the fume ().
Based on the selection of the welding process and materials, the resulting fume may be chemically distinct. Fumes generated during GMA welding processes generally are water-insoluble and closely mimic the metal composition of the welding wire that is consumed during the process. Due to the presence of fluxes, fume formed during MMA welding has been observed to be physically and chemically more complex than fumes generated during GMA welding (Zimmer and Biswas, Citation2001; Jenkins and Eagar, Citation2005; Jenkins et al., Citation2005). Because of the presence of alkali metals (e.g., potassium and sodium) in the fluxes, the fumes from MMA welding processes are very water-soluble (Antonini et al., Citation1999).
Recent studies indicate that a metal oxidation state is highly dependent on welding process and shield gas composition (Keane et al., Citation2009, Citation2010). Mn species, including Mn2+, Mn3+, and Mn4+, have been observed in the generated fume from multiple processes, and the proportions of the different Mn species were dependent on the welding process and settings (Minni et al., Citation1984; Keane et al., Citation2010). Similarly, the concentration of Cr6+ can vary significantly depending on the welding process and shield gas type (Keane et al., Citation2009). These differences in metal solubility and valence states in fumes generated from different welding processes have been shown to influence the bioavailability of the metals after deposition in the respiratory tract and the eventual translocation of the metals to the circulation and other organ systems in animal models.
Using neutron-activation, Lam et al. (Citation1979) demonstrated that the metal constituents of SS welding fumes generated from MMA and GMA processes were cleared in different phases. In the initial phase, intact welding particles cleared from the body within days up to a week by mucociliary and lung macrophage clearance. The clearance rates of each element of the SS fume were similar during this initial phase, indicating that the eliminated particles were transported in their entirety, without separation of the constituents. At the later phase, various elements of the specific fumes cleared at much different rates with biological half-lives of several weeks, indicative of tissue solubilization of the deposited particle. Previous studies by our group indicate that SS welding fumes cleared from the lungs of rats at a slower rate compared to MS fumes (Antonini et al., Citation1996, 2011b). In addition, specific metals in the different welding fumes cleared from the lungs at varying rates after exposure. The more toxic metals (e.g., Mn, Cr) present in some welding fumes were found to clear more quickly and completely from the lungs compared to Fe, likely increasing their translocation from the respiratory system to other organs (Antonini et al., Citation2010).
Welding exposure and health effects
An estimated 430,000 workers are employed full-time in welding operations in the United States (U.S. Bureau of Labor Statistics, 2010), and millions more worldwide are exposed to welding aerosols daily. Welders are part of an heterogeneous workforce employed in a variety of workplace settings; these may include well-ventilated indoor and open-air sites or confined poorly ventilated spaces (e.g., ship hulls, pipelines, building crawl spaces). Because of this, a wide range of exposure concentrations have been measured in workplaces where welding occurs. Personal exposure measurements of ≈200 workers from numerous occupational settings throughout the US indicated that total welding particulate levels ranged from 1.02–37.30 mg/m3 in 1995 and 0.10–18.00 mg/m3 in 1996 (Susi et al., Citation2000). In the assessment of a construction site, Meeker et al. (Citation2007) observed total welding particulate concentrations to range from 2.65–11.6 mg/m3 in settings without local exhaust ventilation, and 3.15–5.44 mg/m3 where local exhaust ventilation was used. Airborne fume concentration levels of individual metals in eight welding companies that mostly performed GMA-MS welding were measured by the Workplace Safety and Health Branch in Manitoba, Canada (Korczynski, Citation2000). The personal exposures to Fe and Mn ranged from 0.04–16.29 mg/m3 and 0.01–4.93 mg/m3, respectively. Studies have reported that workplace levels of individual metals in welding fumes have exceeded airborne exposure limits. Korczynski observed that 19% of welders studied were exposed to levels of Fe that exceeded the American Conference of Governmental Industrial Hygienists (ACGIH) Threshold Limit Value-Time Weighted Average (TLV-TWA) of 5 mg/m3. Moreover, 62% of the welders studied were reportedly exposed to levels of Mn that exceeded the ACGIH TLV-TWA of 0.2 mg/m3. In the assessment of nine construction sites among different welding trades, it was observed that the probability of exceeding the ACGIH TLV-TWA for Mn was sufficiently high to cause health concerns among the construction trades (Rappaport et al., Citation1999).
Hundreds of studies have evaluated the health effects associated with welding fume inhalation. However, these effects are sometimes difficult to assess because of differences in worker populations, work area ventilation, welding processes and materials used, and other occupational exposures besides welding fumes. It has been reported that most career welders experience some type of respiratory disorder during their time of employment (Sferlazza and Beckett, Citation1991; Martin et al., Citation1997; Antonini, Citation2003). Pulmonary effects have included metal fume fever, bronchitis, siderosis, reversible lung function decrements, increased susceptibility to infection, and an increased risk of lung cancer. In addition, a variety of non-pulmonary effects (e.g., neurological, cardio-vascular, and reproductive) have been observed in workers exposed to welding fumes. The occupational-related health effects of greatest concern are the potential development of neurological effects, lung cancer, and decrements in immune function and defense.
Welding fume exposure may be associated with the development of neurological dysfunction similar to Parkinson’s disease. Much of this concern has been attributed to Mn in the generated welding fume. Mn intoxication following chronic human exposure in other occupational settings, such as mining, smelting, ferroalloy, and dry battery industries, has been documented to cause a Parkinson’s disease-like syndrome called ‘manganism’ (Roels et al., Citation1992; Mergler and Baldwin, Citation1997, Lucchini et al., Citation1999). Neurobehavioral changes have been reported in exposed welders (Bowler et al., Citation2007a, b; Ellingsen et al., Citation2008), and case reports show Mn accumulates in dopaminergic brain regions of welders exposed to high concentrations of welding fume (Sadek et al., Citation2003; Josephs et al., Citation2005). In addition, some studies describe a potential link between welding and Parkinsonism, and suggest the possibility of an early-onset Parkinsonism among welders (Racette et al., Citation2001, 2005), whereas other studies do not (Goldman et al., Citation2005; Stampfer, Citation2009; Tanner et al., Citation2009). Recent animal studies demonstrated that repeated exposure to Mn-containing welding fumes modulated molecular factors associated with synaptic transmission, oxidative stress, neuro-inflammation, and mitochondrial dysfunction in dopaminergic targets in rat brains, as well as induced transcriptional changes in a variety of PARK genes (Sriram et al., Citation2010a, b).
Despite inadequate evidence in humans and laboratory animals, welding fume has been classified as ‘possibly carcinogenic’ by the International Agency for Research on Cancer due to the presence of Cr6+ and Ni in SS fumes (IARC, 1990). Multiple epidemiology studies have reported an elevated risk for the development of lung cancer among welders (Hansen et al., Citation1996; Moulin, Citation1997; Becker, Citation1999), whereas others have not (Steenland et al., Citation1991; Hansen et al., Citation1996; Danielsen et al., Citation2000). Moreover, epidemiological studies have been unable to correlate the development of lung cancer solely with exposure to Cr-containing SS welding fume as compared to non-Cr-containing MS fume (Moulin et al., Citation1993; Langard, 1994; Moulin, Citation1997). However, in vitro genotoxicity studies demonstrated that Cr-containing SS, but not MS, welding fumes are mutagenic and induce DNA damage (Hedenstedt et al., Citation1977; Maxild et al., Citation1978). Recent animal studies with MMA- and GMA-generated fumes indicate these to be weak lung tumor initiators in A/J mice, a lung tumor susceptible mouse strain. However, a chronic lung inflammatory response to these fumes was found, which may suggest that long-term exposure could increase lung tumor incidence and/or multiplicity in the A/J model (Solano-Lopez et al., Citation2006; Zeidler-Erdely et al., Citation2008, 2011a, b).
Human immunotoxicity studies
Data from the national analyses of occupational mortality for England and Wales have consistently demonstrated that welders have an increased mortality from pneumonia (Registrar General Citation1958, 1971, 1981). In 1994, Coggon et al. (Citation1994) confirmed these observations using 1979–1980 and 1982–1990 data from the UK that showed an excess of pneumococcal and unspecified lobar pneumonia in men below retirement age of 65 years. Lobar pneumonia, a disease with acute progression, is usually the result of infection by Streptococcus pneumoniae and affects a large and continuous area of a lung lobe. Since a similar mortality pattern was found in other metal fume-exposed workers such as molders and coremakers, Coggon et al. concluded that the primary causative agent could be the metallic fume components or possibly O3 or NO. Studies from the US associating increased mortality from pneumonia due to welding fume exposure are few, but most support an increased risk in welders of pneumonias other than bronchopneumonia (Puntoni et al., Citation1979; Beaumont and Weiss, Citation1980; Polednak, Citation1981; McMillan and Pethybridge, 1983; Milham, Citation1983; Newhouse et al., Citation1985).
In 2003, a hospital-based case-control study in males (mean age of 46 years) by Palmer et al. (Citation2003) determined that inhalation of metal fumes renders the worker susceptible to infectious pneumonia, primarily of the lobar type, and that this was a reversible phenomenon after exposure ceased. Further, the study identified pneumonia of pneumococcal as well as Legionella, Mycoplasma, and Haemophilus influenza origin in the subjects. Also, ferrous metal (with or without other metals/alloys) was found to be associated with a higher risk of pneumonia than non-ferrous exposures. Importantly, the study eliminated other workplace hazards such as coal dust, wood dust, cement dust, and asbestos as contributing to the increased risk in the metal dust-exposed subjects (i.e., welders, molders, coremakers, and die casters) (Palmer et al., Citation2003). These results led Palmer et al. to postulate that ferrous metal fumes may cause oxidative damage to lung host defense or that they may provide an enriched free Fe environment for micro-organisms to temporarily prosper such as that which occurs with sickle cell disease (Barrett-Connor, Citation1971). Moreover, Ghio and Cohen (Citation2005) have theorized that the immunomodulatory effect of particulate matter, such as welding fume, may involve a disruption of Fe homeostasis in cells and tissues. It is possible that other metals may compete with Fe so that lung phagocytes become Fe-insufficient and unable to function properly, while simultaneously allowing for greater free Fe to remain available for pathogens (Cohen et al., Citation2010). A subsequent study by Palmer et al. (Citation2006) revealed that, surprisingly, despite high Fe loads and a low unsaturated Fe-binding capacity in sputum, no overt inflammatory response was found in blood or sputum of welders with a median employment of 10 years. However, an increase in blood eosinophil and basophils in welders vs non-welders, with some correlation to exposure level, was observed. Also found were trends for increased C reactive protein (CRP), neutrophil oxidative burst, sputum IgA, and decreased sputum eosinophils (Palmer et al., Citation2006).
Supportive evidence for metal particulate-induced epithelial damage or macrophage activation causing weak systemic effects via cytokine signaling, however, could not be found in the airways of the patients. Taken together, the findings of this study were difficult for the authors to resolve given the strong evidence for pro-inflammatory lung effects of a variety of metal-rich particulates such as diesel exhaust, residual oil fly ash, particulate matter, zinc oxide, and coal dust (Blanc et al., Citation1991; Carter et al., Citation1997; Salvi et al., Citation2000; Vallyathan et al., Citation2000; Ulrich et al., Citation2002). The authors speculated that the high transitional metal levels in the lung must somehow impair lung host defense and provide a temporary window for infection. Alternatively, apoptosis, via Fe-catalyzed oxidative stress, may impair immune cell activation, which may also lead to increased worker susceptibility (Palmer et al., Citation2006). In fact, apoptosis is a mechanism by which S. pneumonia, the organism presumably at most infective risk to metal fume workers, acts to augment its own pathogenicity (Hakansson et al., 1996).
Case studies of rapidly progressing, fatal pneumonia have been reported in four healthy male welders residing in Texas and Louisiana (Miller et al., Citation1997; Avashia et al., Citation2007). All four cases exhibited symptoms of Bacillus anthracis respiratory disease, and the welders succumbed to the illness 5–8 days after their initial onset of symptoms. The causative agent was determined to be Bacillus cereus, an organism occasionally associated with food-borne illness and merely considered a contaminant in clinical laboratory specimens. In healthy individuals, B. cereus poses no health concern, but could cause a variety of infections in those who are immunocompromised or possess an underlying illness (Drobniewski, Citation1993). It was later discovered that three of the four B. cereus isolates obtained from the patients and/or workplace contained B. anthracis toxin genes, an unusual and unexpected finding (Miller et al., Citation1997; Hoffmaster et al., Citation2004; Avashia et al., Citation2007). In all cases, including another similar non-fatal case involving the same organism, the men were only linked by their metal-working profession (Hoffmaster et al., Citation2004). This association further raises the possibility that exposure to metal fumes may lead to periods of increased susceptibility, even to ubiquitous organisms such as B. cereus.
Immunological screening studies in welders are limited and, in general, report moderate changes for various immune-related parameters. In a cohort of 11 welders and nine grinders (mean age = 31 years; work experience = 8 years), Borska et al. (2003) reported decreased levels of IgG, IgA, and IgM as well as acute phase proteins transferrin, α1-antitrypsin, β2-macroglobulin, haptoglobulin, and ceruloplasmin in the serum. Increased levels of phagocytic cells, C3 complement component, neopterin, β2-macroglobulin, and orosomucoid were also found. Others have reported similar findings of alterations in certain parameters of humoral immunity including decreased IgG, IgA, and increased C3 complement component (Boshnakova et al., Citation1989, Nielsen et al., Citation1993; Hanovcova et al., 1998). In contrast, Tuschl et al. (Citation1997) observed no effect on lymphocytic sub-populations, mixed lymphocyte reactions, leukocytes phagocytosis, or immunoglobulin levels in an examination of 30 welders who worked in a plant where levels of welding fumes typically exceeded Austrian Working Limits of 5 mg/m3. However, the authors reported that welding fumes reduced the cytotoxic activity of lymphokine-activated killer cells which are primarily natural killer (NK) cells. They suggested that the decreased immunocompetent state might subject the welders to an increased risk of infection because NK cells are a main defense mechanism against viruses (Tuschl et al., Citation1997). In a cohort of 74 healthy welders, Boshnakova et al. (Citation1989) found evidence for impaired T- and B-lymphocytes and decreased serum IgG and total E-rosette-forming cells. The authors suggested a link to complex occupational factors, such as the heavy metal Mn, noise, and vibration, as possible causes for the immune suppression.
Other studies have reported effects on lymphocytes in welders. For example, a study examining the effects of Mn exposure in welders (work experience = 6–36 years) found a significant inverse correlation between blood Mn concentrations and CD8+, CD3+, CD4+, naïve T, and total lymphocytes after adjusting for age and smoking (Nakata et al., Citation2006). In addition, B (CD19+)-lymphocyte numbers were also significantly decreased in high Mn-exposed welders. In a 3-year study by Hanovcova et al. (1998), increased lymphocyte counts, T-lymphocyte percentages, leukocyte metabolism, and decreased phagocytosis were found in SS welders (n = 22–53) compared to a standard laboratory reference value/non-exposed persons from the same plant. Exposure to metal fumes has been shown to induce systemic inflammation and oxidative stress parameters in the serum of welders, which could potentially lead to immune effects (Comhair and Erzurum, Citation2002; Han et al., Citation2005; Kim et al., Citation2005; Fang et al., Citation2009). A recent study by du Plessis et al. (2010) measured the oxidative status of peripheral blood mononuclear cells in welders (n = 15, mean age = 32 years, work experience = 0.25–29 years) from a South African manufacturing factory using a GMA welding process. Using flow cytometry, increased levels of reactive oxygen species (ROS) and lipid peroxidation, along with a decrease in intracellular glutathione were found. These data further support a role for an altered oxidative status in the immune suppression that occurs in workers exposed to metal fumes.
Animal immunotoxicity studies
Numerous animal studies have examined the toxic effects of welding fumes generated from MMA and GMA welding using different pulmonary exposure routes, such as pharyngeal aspiration, intratracheal instillation (ITI), and inhalation (Taylor et al., Citation2003; Sung et al., Citation2004; Yu et al., Citation2004; Antonini et al., Citation2007). Overall, analysis of the bronchoalveolar lavage fluid (BALF) has shown significant cytotoxicity, air–blood barrier damage, and an inflammatory cytokine response after welding fume exposure via these routes. In addition, a cellular influx consisting of alveolar macrophages (AM), neutrophils, lymphocytes, and, to a lesser extent, eosinophils was reported. Studies in both rats and mice that directly compared different types of welding fumes showed that SS fumes induced more lung toxicity as compared to MS fumes, an effect likely attributable to the greater proportion of toxic metals, such as Cr (Taylor et al., Citation2003; Zeidler-Erdely et al., Citation2008; Erdely et al., Citation2011b).
Results of lung gene expression studies in both mice and rats indicate a strong immunologic transcriptional response to welding fume (Oh et al., Citation2009, 2011; Yang et al., Citation2009; Zeidler-Erdely et al., Citation2010). A 30-day inhalation exposure to MMA-SS fume in rats resulted in marked changes in several immune-related genes, including matrix metalloproteinase-12 (Mmp12), Cd 5 molecule-like (Cd5l), chemokine (C-C motif) ligand 7 (Ccl7), C-X-C motif chemokine 5 (Cxcl5), and secreted phosphoprotein 1 (Spp1). In addition, triggering receptor expressed on myeloid cells 2 (Trem2), γ-2a immunoglobulin heavy chain (IgG-2a), immunoglobulin heavy chain-1a (Igh-1a), and immunoglobulin heavy locus (Igh) were increased after an exposure and recovery protocol. A second 30-day MMA-SS fume inhalation exposure verified that this gene sub-set was predominant in the response and that the lung showed a lack of adaptation to a repeated exposure (Yang et al., Citation2009; Oh et al., Citation2011). In mice, many of these same genes were altered after GMA welding fume aspiration and inhalation exposure (Zeidler-Erdely et al., Citation2010, 2011c), which suggests a consistency across rodent species and a general response to welding fume-induced lung injury. While only portions of a GMA-SS fume inhalation mouse study (40 mg/m3 for 10 days at 3 h/day with 110–120 µg measured total deposition) have been published (Erdely et al., Citation2011a; Zeidler-Erdely et al., Citation2011c), the preliminary analysis indicates a robust immunologic response through 28 days post-exposure. The functional analysis of pulmonary gene expression changes indicated ‘immune cell trafficking’, ‘infectious disease’, ‘immunological disease’, and ‘anti-microbial response’ as highly significant categories of response. In addition, significant canonical pathways including ‘communication between innate and adaptive immune cells’, ‘dendritic cell maturation’, ‘role of pattern recognition receptor in recognition of bacteria and viruses’, and ‘crosstalk between dendritic cells and NK cells’ suggest prominent immune-mediated signaling. Further, aspirated GMA-SS welding fume (85 μg × 4 doses) in mice also increased lung gene expression of chemotactic and immunomodulatory genes such as chemokine (C-C motif) ligands 3 and 4 (Ccl3 and Ccl4), immunoglobulin M (IgM), and Mmp12 through16 weeks post-exposure. Overall, the study concluded that sustained lung inflammation was associated with alterations in immunomodulatory genes, indicating that a delayed resolution of inflammation was linked to ongoing immune signaling in mice (Zeidler-Erdely et al., Citation2010).
Several animal studies have examined the immunosuppressive effects of welding fume and specifically analyzed the ability of the rat lung to clear a bacterial infection to Listeria monocytogenes after welding fume exposure. A preliminary study compared three fumes, GMA-SS, GMA-MS, and MMA-SS, administered by ITI (2.00 mg/rat) 3 days before pulmonary inoculation, and the subsequent effects on the lung host defense response in rats (Antonini et al., Citation2004). In this study, only the MMA-SS fume resulted in significant weight loss and a reduction in pulmonary clearance of L. monocytogenes after infection. However, bacterial challenge 3 days after welding fume treatment also resulted in enhanced pulmonary polymorphonuclear leukocytes (PMN) influx, ROS production, and BALF NOx production. Interestingly, these factors have been suggested to be mechanisms by which bacterial clearance was enhanced after silica exposure (Antonini et al., Citation2000), but findings with MMA-SS fume suggest only a minor role for these parameters in the effective clearance of a bacterial challenge.
Pre-exposure to MMA-SS fume (2.00 mg/rat) before pulmonary L. monocytogenes challenge has also been shown to alter the induction of several immune-related cytokines. For instance, pre-treatment with MMA-SS fume prevented any L. monocytogenes-induced increases in interleukin (IL)-2 and also augmented IL-10 production after infection (Antonini et al., Citation2004). IL-10 is an anti-inflammatory cytokine produced by various cell types of the innate and adaptive immune response. IL-10 reduces tissue injury of exacerbated immune responses and the expression of pro-inflammatory cytokines (Ouyang et al., Citation2011). While protective, the increased IL-10 may also be a mechanism of immune evasion of bacterial and viral infections (Ouyang et al., Citation2011). IL-2 induces responses that are both inflammatory-generating T-helper type 1 (TH1) and TH2 effector cells, and anti-inflammatory-increasing the survival and functionality of regulatory T-lymphocytes and inhibiting of TH17 differentiation (Hoyer et al., Citation2008). Interestingly, IL-2-deficient mice have increased TH1 responsiveness and develop autoimmunity and inflammation, a consequence that may be linked to reduced regulatory T-lymphocytes (Hoyer et al., Citation2008). These studies indicate that IL-2 plays a homeostatic role in an inflammatory immune response. The reduced IL-2 response with MMA-SS fume treatment alone and before L. monocytogenes challenge suggests altered T-cell activity.
The majority of the inflammatory effects produced by MMA-SS welding fume can be attributed to the soluble, Cr-abundant component (Taylor et al., Citation2003; Antonini and Roberts, Citation2007). The immunosuppressive effects of Cr are well documented (Cohen, Citation2004). For example, a study that examined pre-treatment with the total MMA-SS fume and individual metal components before infection showed that soluble Cr in the fume, as opposed to the Ni or Fe, resulted in reduced bacterial clearance and excessive lung injury in rats (Antonini and Roberts, Citation2007). In contrast to the total fume, there was no effect on IL-10 with soluble Cr alone, which suggests IL-10 may not play a major role. IL-2 levels were reduced by soluble Cr treatment alone and pre-treatment before bacterial infection, suggesting an altered T-lymphocyte activation; this result is consistent with MMA-SS fume exposure (Antonini et al., Citation2004; Antonini and Roberts, Citation2007). Pre-exposure with soluble Cr greatly enhanced infection-induced IL-12 production in the BALF (Antonini and Roberts, Citation2007). IL-12 aids in the development of cell mediated immunity by increasing interferon-γ (IFNγ) production, differentiating T-lymphocytes into TH1 effector cells, and enhancing the cytotoxicity of NK and cytotoxic T-lymphocytes (Gee et al., Citation2009). While this result suggested an enhanced ability for bacterial lung clearance, this was not the case in the study by Antonini and Roberts (Citation2007).
The development of the robotic welder at the National Institute for Occupational Safety and Health (Antonini et al., Citation2006) has allowed the effects of inhalation of GMA-SS and GMA-MS welding fumes with L. monocytogenes challenge to be studied in rats. Unlike in the studies that used ITI, direct inhalation (15 mg or 40 mg/m3 × 3 h/day × 10 days) of both of these welding fumes resulted in reduced body weight and bacterial clearance after infection (Antonini et al., Citation2007, 2009). Following GMA-SS fume exposure, the reduced bacterial clearance was accompanied by marked enhancement of tumor necrosis factor (TNF)-α, IL-6, IL-10, IL-12, monocyte chemotactic protein-1 (MCP-1), and macrophage inflammatory protein-2 (MIP-2) and a significant reduction in IL-2 after infection compared to air controls (Antonini et al., Citation2007). The reduction in IL-2 and increased IL-12 was consistent with MMA-SS fume and/or soluble Cr. Interestingly, and in contrast to MMA-SS fume and soluble Cr, GMA-SS fume inhalation reduced ROS production from AM measured via chemiluminesence. Attenuated AM activity has also been found in conjunction with reduced bacterial clearance after diesel and O3 exposure (Van Loveren et al., Citation1988; Cohen et al., Citation2002; Yin et al., Citation2002). Inhalation exposure to GMA-MS fume (40 mg/m3 × 3 h/day × 10 days) did not result in an overt lung inflammatory response, but significantly reduced pulmonary bacterial clearance. In addition, pre-exposure to GMA-MS welding fume only enhanced TNFα and MIP-2 following infection (Antonini et al., Citation2009). Although differences were not significant, IL-2 and IL-12 responses were similar compared to SS welding exposures.
Findings from studies with L. monocytogenes challenge were inconsistent. The differences between aspiration and ITI of GMA fumes may be the result of AM function and cellular influx. After ITI exposure, there was an immediate and abundant influx of PMN that was delayed or absent with inhalation (Taylor et al., Citation2003; Antonini et al., Citation2007, 2009). The delayed response after inhalation to GMA fumes was attributed to inhibitory effects on AM chemotactic signaling (Antonini et al., Citation2007, 2009). The lack of an initial PMN influx could diminish the effectiveness of the lung to clear bacteria post-exposure. The question then becomes, why does MMA-SS fume exposure, which resulted in a significant early PMN influx, result in reduced bacterial clearance? Perhaps the answer lies between the effectiveness of local and extravasating leukocytes and the altered pulmonary cytokine response after L. monocytogenes challenge. As discussed later, circulating leukocytes exhibit a diminished response to a secondary stimulus after MMA-SS fume exposure (unpublished observations). Cohen et al. (Citation1998) also showed that AM harvested from rats exposed to Cr had a reduced response ex vivo when stimulated with lipopolysaccharide (LPS). These studies indicate reduced effectiveness of local and circulating leukocytes after exposure.
GMA-SS fume-exposed rats had a greater post-infection inflammatory response (), larger reductions in body weight, and poorer bacterial clearance () than GMA-MS fume-exposed rats. While only qualitatively comparable because of different exposure routes, the fold increase of bacteria colony forming units due to MMA-SS fume exposure was 1.6–1.8 (Antonini et al., Citation2004; Antonini and Roberts, Citation2007). Therefore, there is a graded response in reduced immune function for the different types of welding fume that corresponds to the level of general cytotoxicity and inflammation (SS > MS). The specific mechanisms responsible for reduced immune competence after GMA-MS fume exposure are unknown. Possibly, Fe, the primary metal component of the fume, promotes general bacteria viability. While plausible, exposure to iron oxide (Fe2O3) had no effect on bacterial clearance in rats (Antonini and Roberts, Citation2007). In comparison, both SS fumes resulted in a further reduced capacity to manage a bacterial challenge compared to GMA-MS fumes. These effects are likely linked to the differences in metal composition between the fumes. Cr in the SS fumes is the most suspect because of its immunosuppressive actions (Cohen, Citation2004). Bacterial challenge after SS exposure in rats resulted in alterations of several cytokines linked to innate and adaptive immunity not significantly altered with MS exposure. In addition, the inability of these SS-exposed hosts to efficiently respond to and clear L. monocytogenes (as compared to infected shams) caused an augmented pulmonary toxicity, likely exacerbating the situation.
Table 3. Enhanced cytokine response to GMA-SS welding fume compared to GMA-MS.
In addition to the localized effects, a few animal studies provide evidence of systemic immunosuppression. One study evaluated the local and systemic humoral immune response following aspiration of multiple doses (5–20 mg/kg body weight every 5 days × 4 doses) of MMA-SS fume in mice (Anderson et al., Citation2007). In vitro exposure of splenocytes to MMA-SS fume reduced IgM activity in response to sheep red blood cells (SRBC), which was the result of the soluble fume fraction. After an in vivo exposure to this fume, splenocytes also showed a significant decrease in the IgM response to SRBC, but only at the highest dose of 20 mg/kg. Localized immunosuppression was determined in B-lymphocytes harvested from lung-associated lymph nodes. There was a dose response of decreased IgM for the total and soluble fraction of MMA-SS fume, but not the insoluble fraction. The total number of B- and T-lymphocytes in the lung-associated lymph nodes increased, indicating the effect was the result of reduced function.
Recently, Antonini et al. (submitted companion paper) examined the effect of ITI pulmonary treatment of two doses of welding fumes that contained different Mn levels on the systemic immune cell profile in rats. Systemic PMN were increased, in most cases, after single or repeated ITI exposure to either a high (2.00 mg/rat) or a low (0.125 mg/rat) Mn welding fume. In terms of circulating lymphocyte number, the high Mn welding fume caused the greatest effect on the systemic immune T-lymphocyte differential, whereas neither dose of the low Mn fume significantly changed the number of circulating total T-lymphocyte or T-lymphocyte sub-populations. Significant reductions in circulating total T-lymphocytes and sub-sets were observed after ITI treatment with a 2.00 mg/rat dose of the high Mn fume. The response was similar, regardless of whether the animals were exposed to 2.00 mg/rat of the high Mn fume via single or repeated ITI treatments, indicating that the effect was acute and persisted over time. Changes in the number of whole blood B-lymphocytes or in circulating levels of IgG, IgA, and IgM were not found after treatment with either welding fume. Importantly, a high repeated pulmonary dose (2.00 mg/rat ITI, once a week for 7 weeks) of pure manganese chloride (MnCl2) did not cause a significant decrease in circulating T-lymphocytes and lymphocyte sub-sets. This indicated that the immunomodulatory effects from the parent welding fumes were likely due to pulmonary exposure to a combination of several fume-associated metals, and not solely dependent on Mn.
Unpublished experiments from our group have revealed a reduced capacity of circulating leukocytes to respond to a second stimulus after welding fume exposure. Rats were exposed to MMA-SS and sacrificed 24 h post-exposure. Whole blood was collected and incubated in sterile media with or without LPS. After 24-h incubation, supernatants were collected for cytokine analysis. Despite the increased total number of circulating leukocytes at 24 h post-exposure, LPS-induced inflammatory proteins were significantly less in MMA-SS fume-exposed rats (unpublished observations). Ongoing studies are analyzing whole blood cell gene expression by microarray after exposure and ex vivo stimulation to assess mechanisms related to this depressed activity.
Rim et al. (Citation2004) used suppression-subtractive hybridization and a cDNA microarray to determine expression changes in rat mononuclear cells after 30 days of host exposure to MMA-SS fume by inhalation (108 mg/m3 × 2 h/day × 30 days). The overall finding of this study was that MMA-SS fume down-regulated ~3-times more genes than were increased. The result was suggested to be in response to the toxicity of the prolonged exposure to MMA-SS fume. Together, these studies addressing systemic immunosuppression strongly suggest a reduced capacity to fight off an infection after welding fume exposure, in particular MMA-SS fume, both locally and systemically.
A recent study showed that mice exposed by inhalation of GMA-SS fume (40 mg/m3 for 3 h/day for 10 days—daily deposition of 11.8 µg/day) resulted in local and systemic immune-related responses (Erdely et al., Citation2011a). This study analyzed whole blood cells, aorta, and lung by microarray, and used pathway analysis to identify consistent mechanisms of effect across tissue types. Interestingly, the major functional categories in the vasculature and blood cells were related to infectious mechanism/disease (). Systemically, the response to GMA-SS fume appeared to be as if the particle was an infection, a response that was initiated by the lung. The major finding of the study was a prominent Type I IFN response mediated by IRF7-dependent signaling that was consistent across tissue and time. Type I IFN receptor knockout mice are resistant to the effects of L. monocytogenes (Auerbuch et al., Citation2004), which may provide an additional mechanism by which GMA-SS fume elicited enhanced adverse response to infection compared to GMA-MS fume (, ).
Table 4. Functional analysis of extra-pulmonary effects following GMA-SS inhalation.
Figure 2. Comparison of the response to L. monocytogenes following gas metal arc (GMA)-mild steel (MS) or -stainless steel (SS) welding fume pre-exposure. Rats were exposed by inhalation to either GMA-MS or GMA-SS fume at 40 mg/m3 for 3 h/day for 3 days and then challenged with L. monocytogenes infection. At 3 days post-infection, colony-forming units (CFU) increased significantly and body weight decreased with exposure to either fume (adapted from Antonini et al., Citation2007, 2009). When represented as fold change from respective air-exposed L. monocytogenes challenged sham, there was a greater effect following GMA-SS welding fume exposure compared to GMA-MS; *p < 0.05 MS vs SS.
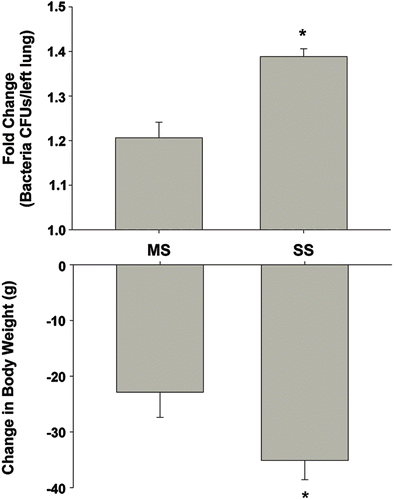
Summary
The majority of epidemiological studies suggest a reduced ability of workers exposed to metal fumes to ward off a pulmonary infection, thus allowing a window of opportunity for pulmonary infection shortly after or during a metal fume exposure (). These studies are well supported by infectivity studies in animal models (). Mechanistic studies suggest a diminished functionality of local and circulating immune cells. The reduced functionality of immune competent cells was associated with altered immune-related cytokine and antibody production as well as impaired resolution of local inflammation. The reduced capacity to eliminate infectious agents results in enhanced pulmonary injury, exacerbating the initial exposure effects and perhaps providing the potential to extend the window of immunosuppression.
Table 5. Human studies related to welding fume exposure and immune function.
Table 6. Animal studies related to welding fume exposure and immune function.
Disclaimer
The findings and conclusions of this paper have not been formally disseminated by NIOSH and should not be construed to represent any agency determination or policy.
Declaration of interest
The authors report no conflicts of interest. The authors alone are responsible for the content and writing of the paper.
References
- Anderson, S. E., Meade, B. J., Butterworth, L. F.Munson, A. E. 2007. The humoral immune response of mice exposed to manual metal arc stainless steel-welding fumes. J Immunotoxicol 4:15–23.
- Antonini, J. M. 2003. Health effects of welding. Crit Rev Toxicol 33:61–103.
- Antonini, J. M.Roberts, J. R. 2007. Chromium in stainless steel welding fume suppresses lung defense responses against bacterial infection in rats. J Immunotoxicol 4:117–127.
- Antonini, J. M., Afshari, A. A., Stone, S., Chen, B., Schwegler-Berry, D., Fletcher, W. G., Goldsmith, W. T., Vandestouwe, K. H., McKinney, W., Castranova, V.Frazer, D. G. 2006. Design, construction, and characterization of a novel robotic welding fume generator and inhalation exposure system for laboratory animals. J Occup Environ Hyg 3:194–203; quiz D45.
- Antonini, J. M., Keane, M., Chen, B. T., Stone, S., Roberts, J. R., Schwegler-Berry, D., Andrews, R. N., Frazer, D. G.Sriram, K. 2011a. Alterations in welding process voltage affect the generation of ultrafine particles, fume composition, and pulmonary toxicity. Nanotoxicology 5:700–710.
- Antonini, J. M., Krishna Murthy, G. G., Rogers, R. A., Albert, R., Ulrich, G. D.Brain, J. D. 1996. Pneumotoxicity and pulmonary clearance of different welding fumes after intratracheal instillation in the rat. Toxicol Appl Pharmacol 140:188–199.
- Antonini, J. M., Lawryk, N. J., Murthy, G. G.Brain, J. D. 1999. Effect of welding fume solubility on lung macrophage viability and function in vitro. J Toxicol Environ Health Part A 58:343–363.
- Antonini, J. M., Roberts, J. R., Chapman, R. S., Soukup, J. M., Ghio, A. J., and Sriram, K. 2010. Pulmonary toxicity and extrapulmonary tissue distribution of metals after repeated exposure to different welding fumes. Inhal Toxicol 22:805–816.
- Antonini, J. M., Roberts, J. R., Stone, S., Chen, B. T., Schwegler-Berry, D., Chapman, R., Zeidler-Erdely, P. C., Andrews, R. N., and Frazer, D. G. 2011. Persistence of deposited metals in the lungs after stainless steel and mild steel welding fume inhalation in rats. Arch Toxicol 85:487–498.
- Antonini, J. M., Roberts, J. R., Stone, S., Chen, B. T., Schwegler-Berry, D., and Frazer, D. G. 2009. Short-term inhalation exposure to mild steel welding fume had no effect on lung inflammation and injury but did alter defense responses to bacteria in rats. Inhal Toxicol 21:182–192.
- Antonini, J. M., Stone, S., Roberts, J. R., Chen, B., Schwegler-Berry, D., Afshari, A. A., and Frazer, D. G. 2007. Effect of short-term stainless steel welding fume inhalation exposure on lung inflammation, injury, and defense responses in rats. Toxicol Appl Pharmacol 223:234–245.
- Antonini, J. M., Taylor, M. D., Millecchia, L., Bebout, A. R., and Roberts, J. R. 2004. Suppression in lung defense responses after bacterial infection in rats pretreated with different welding fumes. Toxicol Appl Pharmacol 200:206–218.
- Antonini, J. M., Yang, H. M., Ma, J. Y., Roberts, J. R., Barger, M. W., Butterworth, L., Charron, T. G., and Castranova, V. 2000. Subchronic silica exposure enhances respiratory defense mechanisms and the pulmonary clearance of Listeria monocytogenes in rats. Inhal Toxicol 12:1017–1036.
- Auerbuch, V., Brockstedt, D. G., Meyer-Morse, N., O’Riordan, M., and Portnoy, D. A. 2004. Mice lacking the type I interferon receptor are resistant to Listeria monocytogenes. J Exp Med 200:527–533.
- Avashia, S. B., Riggins, W. S., Lindley, C., Hoffmaster, A., Drumgoole, R., Nekomoto, T., Jackson, P. J., Hill, K. K., Williams, K., Lehman, L., Libal, M. C., Wilkins, P. P., Alexander, J., Tvaryanas, A., and Betz, T. 2007. Fatal pneumonia among metalworkers due to inhalation exposure to Bacillus cereus containing Bacillus anthracis toxin genes. Clin Infect Dis 44:414–416.
- Barrett-Connor, E. 1971. Bacterial infection and sickle cell anemia. An analysis of 250 infections in 166 patients and a review of the literature. Medicine (Baltimore) 50:97–112.
- Beaumont, J. J., and Weiss, N. S. 1980. Mortality of welders, shipfitters, and other metal trades workers in boilermakers Local No. 104, AFL-CIO. Am J Epidemiol 112:775–786.
- Becker, N. 1999. Cancer mortality among arc welders exposed to fumes containing chromium and nickel. Results of a third follow-up: 1989-1995. J Occup Environ Med 41:294–303.
- Beckett, W. S. 1996. Welding. In: Occupational and Environmental Respiratory Disease (Harber, P., Schenker, M. B., and Balmes, J. R., Eds.). St. Louis, MO: Mosby, pp. 704–717.
- Blanc, P., Wong, H., Bernstein, M. S., Boushey, H. A. 1991. An experimental human model of metal fume fever. Ann Intern Med 114:930–936.
- Borská, L., Fiala, Z., Ctirad, A., Krejsek, J., and Tejral, J. 2003. Health risk of occupational exposure in welding processes II. Immunological effects. Acta Medica (Hradec Kralove) 46:31–35.
- Boshnakova, E., Divanyan, H., Zlatarov, I., Marovsky, S., Kisyova, K., Zanev, D., Karev, G., and Marinova, T. 1989. Immunological screening of welders. J Hyg Epidemiol Microbiol Immunol 33:379–382.
- Bowler, R. M., Nakagawa, S., Drezgic, M., Roels, H. A., Park, R. M., Diamond, E., Mergler, D., Bouchard, M., Bowler, R. P., and Koller, W. 2007a. Sequelae of fume exposure in confined space welding: a neurological and neuropsychological case series. Neurotoxicology 28:298–311.
- Bowler, R. M., Roels, H. A., Nakagawa, S., Drezgic, M., Diamond, E., Park, R., Koller, W., Bowler, R. P., Mergler, D., Bouchard, M., Smith, D., Gwiazda, R., and Doty, R. L. 2007b. Dose-effect relationships between manganese exposure and neurological, neuropsychological and pulmonary function in confined space bridge welders. Occup Environ Med 64:167–177.
- Carter, J. D., Ghio, A. J., Samet, J. M., and Devlin, R. B. 1997. Cytokine production by human airway epithelial cells after exposure to an air pollution particle is metal-dependent. Toxicol Appl Pharmacol 146:180–188.
- Coggon, D., Inskip, H., Winter, P., and Pannett, B. 1994. Lobar pneumonia: An occupational disease in welders. Lancet 344:41–43.
- Cohen, M. D. 2004. Pulmonary immunotoxicology of select metals: aluminum, arsenic, cadmium, chromium, copper, manganese, nickel, vanadium, and zinc. J Immunotoxicol 1:39–69.
- Cohen, M. D., Sisco, M., Baker, K., Li, Y., Lawrence, D., van Loveren, H., Zelikoff, J. T., and Schlesinger, R. B. 2002. Effects of inhaled ozone on pulmonary immune cells critical to antibacterial responses in situ. Inhal Toxicol 14:599–619.
- Cohen, M. D., Sisco, M., Prophete, C., Yoshida, K., Chen, L. C., Zelikoff, J. T., Smee, J., Holder, A. A., Stonehuerner, J., Crans, D. C., and Ghio, A. J. 2010. Effects of metal compounds with distinct physicochemical properties on iron homeostasis and antibacterial activity in the lungs: Chromium and vanadium. Inhal Toxicol 22:169–178.
- Cohen, M. D., Zelikoff, J. T., Chen, L. C., and Schlesinger, R. B. 1998. Immunotoxicologic effects of inhaled chromium: role of particle solubility and co-exposure to ozone. Toxicol Appl Pharmacol 152:30–40.
- Comhair, S. A., and Erzurum, S. C. 2002. Antioxidant responses to oxidant-mediated lung diseases. Am J Physiol Lung Cell Mol Physiol 283:L246–L255.
- Danielsen, T. E., Langård, S., and Andersen, A. 2000. Incidence of cancer among welders and other shipyard workers with information on previous work history. J Occup Environ Med 42:101–109.
- Drobniewski, F. A. 1993. Bacillus cereus and related species. Clin Microbiol Rev 6:324–338.
- duPlessis, L., Laubscher, P., Jooste, J., du Plessis, J., Franken, A., van Aarde, N., and Eloff, F. 2010. Flow cytometric analysis of the oxidative status in human peripheral blood mononuclear cells of workers exposed to welding fumes. J Occup Environ Hyg 7:367–374.
- Ellingsen, D. G., Konstantinov, R., Bast-Pettersen, R., Merkurjeva, L., Chashchin, M., Thomassen, Y., and Chashchin, V. 2008. A neurobehavioral study of current and former welders exposed to manganese. Neurotoxicology 29:48–59.
- Erdely, A., Hulderman, T., Liston, A. L., Salmen-Muniz, R., Stone, S., Chen, B. T., Frazer, D. G., Li, S., Kashon, M. L., Antonini, J. M., Simeonova, P. P. Zeidler-Erdely, P. C. 2011a. Interferon signaling, systemic inflammation and atherosclerosis following welding fume inhalation exposure: From the lung to the blood to the vasculature. The Toxicologist 120.
- Erdely, A., Salmen-Muniz, R., Liston, A., Hulderman, T., Zeidler-Erdely, P. C., Antonini, J. M., and Simeonova, P. P. 2011b. Relationship between pulmonary and systemic markers of exposure to multiple types of welding particulate matter. Toxicology 287:153–159.
- Fang, S. C., Cavallari, J. M., Eisen, E. A., Chen, J. C., Mittleman, M. A., and Christiani, D. C. 2009. Vascular function, inflammation, and variations in cardiac autonomic responses to particulate matter among welders. Am J Epidemiol 169:848–856.
- Gee, K., Guzzo, C., Che Mat, N. F., Ma, W., and Kumar, A. 2009. The IL-12 family of cytokines in infection, inflammation and autoimmune disorders. Inflamm Allergy Drug Targets 8:40–52.
- Ghio, A. J., and Cohen, M. D. 2005. Disruption of iron homeostasis as a mechanism of biologic effect by ambient air pollution particles. Inhal Toxicol 17:709–716.
- Goldman, S. M., Tanner, C. M., Olanow, C. W., Watts, R. L., Field, R. D., and Langston, J. W. 2005. Occupation and parkinsonism in three movement disorders clinics. Neurology 65:1430–1435.
- Håkansson, A., Carlstedt, I., Davies, J., Mossberg, A. K., Sabharwal, H., and Svanborg, C. 1996. Aspects on the interaction of Streptococcus pneumoniae and Haemophilus influenzae with human respiratory tract mucosa. Am J Respir Crit Care Med 154:S187–S191.
- Han, S. G., Kim, Y., Kashon, M. L., Pack, D. L., Castranova, V., and Vallyathan, V. 2005. Correlates of oxidative stress and free-radical activity in serum from asymptomatic shipyard welders. Am J Respir Crit Care Med 172:1541–1548.
- Hanovcová, I., Chýlková, V., Tejral, J., Andrýs, C., Procházková, J., Turková, M., Srb, V., Ettlerová, E., and Kokes, J. 1998. Long-term monitoring of the immune reactivity of stainless steel welders. Cent Eur J Public Health 6:51–56.
- Hansen, K. S., Lauritsen, J. M., and Skytthe, A. 1996. Cancer incidence among mild steel and stainless steel welders and other metal workers. Am J Ind Med 30:373–382.
- Hedenstedt, A., Jenssen, D., Lidestein, B.-M, Ramel, C., Rannug, U., and Stern, R. M. 1977. Mutagenicity of fume particles from stainless steel welding. Scand J Work Environ Health 3:203–211.
- Hoffmaster, A. R., Hill, K. K., Gee, J. E., Marston, C. K., De, B. K., Popovic, T., Sue, D., Wilkins, P. P., Avashia, S. B., Drumgoole, R., Helma, C. H., Ticknor, L. O., Okinaka, R. T., and Jackson, P. J. 2006. Characterization of Bacillus cereus isolates associated with fatal pneumonias: Strains are closely related to Bacillus anthracis and harbor B. anthracis virulence genes. J Clin Microbiol 44:3352–3360.
- Hoffmaster, A. R., Ravel, J., Rasko, D. A., Chapman, G. D., Chute, M. D., Marston, C. K., De, B. K., Sacchi, C. T., Fitzgerald, C., Mayer, L. W., Maiden, M. C., Priest, F. G., Barker, M., Jiang, L., Cer, R. Z., Rilstone, J., Peterson, S. N., Weyant, R. S., Galloway, D. R., Read, T. D., Popovic, T., and Fraser, C. M. 2004. Identification of anthrax toxin genes in a Bacillus cereus associated with an illness resembling inhalation anthrax. Proc Natl Acad Sci USA 101:8449–8454.
- Hoyer, K. K., Dooms, H., Barron, L., and Abbas, A. K. 2008. Interleukin-2 in the development and control of inflammatory disease. Immunol Rev 226:19–28.
- IARC. International Agency for Research on Cancer. 1990. IARC Monographs on the Evaluation of Carcinogenic Risks to Humans 49. Geneva: WHO Press.
- Jenkins, N., Pierce, W. M. Eager, T. W. 2005. Particle size distribution of gas metal and flux cored arc welding fumes. Welding J. 84:156s–163s.
- Jenkins, N. T. Eagar, T. W. 2005. Chemical analysis of welding fume particles. Welding J. 84:87s–93s.
- Josephs, K. A., Ahlskog, J. E., Klos, K. J., Kumar, N., Fealey, R. D., Trenerry, M. R., and Cowl, C. T. 2005. Neurologic manifestations in welders with pallidal MRI T1 hyperintensity. Neurology 64:2033–2039.
- Keane, M., Stone, S., and Chen, B. 2010. Welding fumes from stainless steel gas metal arc processes contain multiple manganese chemical species. J Environ Monit 12:1133–1140.
- Keane, M., Stone, S., Chen, B., Slaven, J., Schwegler-Berry, D., and Antonini, J. 2009. Hexavalent chromium content in stainless steel welding fumes is dependent on the welding process and shield gas type. J Environ Monit 11:418–424.
- Kim, J. Y., Chen, J. C., Boyce, P. D., and Christiani, D. C. 2005. Exposure to welding fumes is associated with acute systemic inflammatory responses. Occup Environ Med 62:157–163.
- Korczynski, R. E. 2000. Occupational health concerns in the welding industry. Appl Occup Environ Hyg 15:936–945.
- Lam, H. F., Hewitt, P. J. Hicks, R. 1979. A study of pulmonary deposition, and the elimination of some constituent metals from welding fume in laboratory animals. Ann. Occup. Hyg. 21:363–373.
- Langård, S. 1994. Nickel-related cancer in welders. Sci Total Environ 148:303–309.
- Lockey, J. E., Schenker, M. B., Howden, D. G., Desmeules, M. J., Saracci, R., Sprince, N. L., and Harber, P. I. 1988. Current issues in occupational lung disease. Am Rev Respir Dis 138:1047–1050.
- Lucchini, R., Apostoli, P., Perrone, C., Placidi, D., Albini, E., Migliorati, P., Mergler, D., Sassine, M. P., Palmi, S., and Alessio, L. 1999. Long-term exposure to “low levels” of manganese oxides and neurofunctional changes in ferroalloy workers. Neurotoxicology 20:287–297.
- Martin, C. J., Guidotti, T. L. Langãrd, S. 1997. Respiratory hazards of welding. Clin Pulm Med 4:194–204.
- Maxild, J., Andersen, M., and Kiel, P. 1978. Mutagenicity of fume particles from metal arc welding on stainless steel in the Salmonella/microsome test. Mutat Res 56:235–243.
- McMillan, G. H., and Pethybridge, R. J. 1983. The health of welders in naval dockyards: Proportional mortality study of welders and two control groups. J Soc Occup Med 33:75–84.
- Meeker, J. D., Susi, P., and Flynn, M. R. 2007. Manganese and welding fume exposure and control in construction. J Occup Environ Hyg 4:943–951.
- Mergler, D., and Baldwin, M. 1997. Early manifestations of manganese neurotoxicity in humans: An update. Environ Res 73:92–100.
- Milham, S. 1983. Occupational mortality in Washingotn State 1950–1979. Cincinatti. OH: DHHS [NIOSH] Publication #83–116.
- Miller, J. M., Hair, J. G., Hebert, M., Hebert, L., Roberts, F. J. Jr., Weyant, R. S. 1997. Fulminating bacteremia and pneumonia due to Bacillus cereus. J Clin Microbiol 35:504–507.
- Minni, E., Gustafsson, T. E., Koponen, M., Kalliomaki, P.-L. 1984. A study of the chemical structure of particles in the welding fumes of mild and stainless steel. J Aerosol Sci 15:57–68.
- Moulin, J. J. 1997. A meta-analysis of epidemiologic studies of lung cancer in welders. Scand J Work Environ Health 23:104–113.
- Moulin, J. J., Wild, P., Haguenoer, J. M., Faucon, D., De Gaudemaris, R., Mur, J. M., Mereau, M., Gary, Y., Toamain, J. P., Birembaut, Y., Blanc, M., Debiolles, M. P., Jegaden, D., Laterriere, B., Leonard, M., Marini, F., Massardier, C., Moulin, M., Reure, M., Rigal, L., Robert, G. Viossat, M. 1993. A mortality study among mild steel and stainless steel welders. Br J Ind Med 50:234–243.
- Nakata, A., Araki, S., Park, S. H., Park, J. T., Kim, D. S., Park, H. C., and Yokoyama, K. 2006. Decreases in CD8+ T, naive (CD4+CD45RA+) T, and B (CD19+) lymphocytes by exposure to manganese fume. Ind Health 44:592–597.
- Newhouse, M. L., Oakes, D., and Woolley, A. J. 1985. Mortality of welders and other craftsmen at a shipyard in NE England. Br J Ind Med 42:406–410.
- Nielsen, J., Dahlqvist, M., Welinder, H., Thomassen, Y., Alexandersson, R., and Skerfving, S. 1993. Small airways function in aluminium and stainless steel welders. Int Arch Occup Environ Health 65:101–105.
- Oh, J. H., Yang, M. J., Heo, J. D., Yang, Y. S., Park, H. J., Park, S. M., Kwon, M. S., Song, C. W., Yoon, S. Yu, I. J. 2011. Inflammatory response in rat lungs with recurrent exposure to welding fumes: A transcriptomic approach. Toxicol. Ind. Health. On-line 5 July 2011; DOI: 10.1177/0748233711410906.
- Oh, J. H., Yang, M. J., Yang, Y. S., Park, H. J., Heo, S. H., Lee, E. H., Song, C. W., and Yoon, S. 2009. Microarray-based analysis of the lung recovery process after stainless-steel welding fume exposure in Sprague-Dawley rats. Inhal Toxicol 21:347–373.
- Ouyang, W., Rutz, S., Crellin, N. K., Valdez, P. A., and Hymowitz, S. G. 2011. Regulation and functions of the IL-10 family of cytokines in inflammation and disease. Annu Rev Immunol 29:71–109.
- Palmer, K. T., McNeill Love, R. M., McNeill-Love, R., Poole, J. R., Coggon, D., Frew, A. J., Linaker, C. H., and Shute, J. K. 2006. Inflammatory responses to the occupational inhalation of metal fume. Eur Respir J 27:366–373.
- Palmer, K. T., Poole, J., Ayres, J. G., Mann, J., Burge, P. S., and Coggon, D. 2003. Exposure to metal fume and infectious pneumonia. Am J Epidemiol 157:227–233.
- Palmer, W. G. Eaton, J. C. 2001. Effects of Welding on Health, XI. Miami, FL: American Welding Society.
- Polednak, A. P. 1981. Mortality among welders, including a group exposed to nickel oxides. Arch Environ Health 36:235–242.
- Puntoni, R., Vercelli, M., Merlo, F., Valerio, F., and Santi, L. 1979. Mortality among shipyard workers in Genoa, Italy. Ann N Y Acad Sci 330:353–377.
- Racette, B. A., McGee-Minnich, L., Moerlein, S. M., Mink, J. W., Videen, T. O., and Perlmutter, J. S. 2001. Welding-related parkinsonism: Clinical features, treatment, and pathophysiology. Neurology 56:8–13.
- Racette, B. A., Tabbal, S. D., Jennings, D., Good, L., Perlmutter, J. S., and Evanoff, B. 2005. Prevalence of parkinsonism and relationship to exposure in a large sample of Alabama welders. Neurology 64:230–235.
- Rappaport, S. M., Weaver, M., Taylor, D., Kupper, L., and Susi, P. 1999. Application of mixed models to assess exposures monitored by construction workers during hot processes. Ann Occup Hyg 43:457–469.
- Registrar General. 1958. Decennial supplement England and Wales, 1951. Occupational Mortality, Part II, 1. London, UK: Registrar General.
- Registrar General. 1971. Decennial supplement England and Wales, 1961: Occupational mortality tables. London, UK: Registrar General.
- Registrar General. 1981. Decennial supplement England and Wales, 1971, Series DS, No. 1. London, UK: Registrar General.
- Rim, K. T., Park, K. K., Sung, J. H., Chung, Y. H., Han, J. H., Cho, K. S., Kim, K. J., and Yu, I. J. 2004. Gene-expression profiling using suppression-subtractive hybridization and cDNA microarray in rat mononuclear cells in response to welding-fume exposure. Toxicol Ind Health 20:77–88.
- Roels, H. A., Ghyselen, P., Buchet, J. P., Ceulemans, E., and Lauwerys, R. R. 1992. Assessment of the permissible exposure level to manganese in workers exposed to manganese dioxide dust. Br J Ind Med 49:25–34.
- Sadek, A. H., Rauch, R., and Schulz, P. E. 2003. Parkinsonism due to manganism in a welder. Int J Toxicol 22:393–401.
- Salvi, S. S., Nordenhall, C., Blomberg, A., Rudell, B., Pourazar, J., Kelly, F. J., Wilson, S., Sandström, T., Holgate, S. T., and Frew, A. J. 2000. Acute exposure to diesel exhaust increases IL-8 and GRO-α production in healthy human airways. Am J Respir Crit Care Med 161:550–557.
- Sferlazza, S. J., and Beckett, W. S. 1991. The respiratory health of welders. Am Rev Respir Dis 143:1134–1148.
- Solano-Lopez, C., Zeidler-Erdely, P. C., Hubbs, A. F., Reynolds, S. H., Roberts, J. R., Taylor, M. D., Young, S. H., Castranova, V., and Antonini, J. M. 2006. Welding fume exposure and associated inflammatory and hyperplastic changes in the lungs of tumor-susceptible A/J mice. Toxicol Pathol 34:364–372.
- Sowards, J. W., Ramierz, A. J., Dickinson, D. W.Lippold, J. C. 2010. Characterization of welding fume from SMAW electrodes. Welding J. 89:89s–90s.
- Sriram, K., Lin, G. X., Jefferson, A. M., Roberts, J. R., Chapman, R. S., Chen, B. T., Soukup, J. M., Ghio, A. J., and Antonini, J. M. 2010a. Dopaminergic neurotoxicity following pulmonary exposure to manganese-containing welding fumes. Arch Toxicol 84:521–540.
- Sriram, K., Lin, G. X., Jefferson, A. M., Roberts, J. R., Wirth, O., Hayashi, Y., Krajnak, K. M., Soukup, J. M., Ghio, A. J., Reynolds, S. H., Castranova, V., Munson, A. E., and Antonini, J. M. 2010b. Mitochondrial dysfunction and loss of Parkinson’s disease-linked proteins contribute to neurotoxicity of manganese-containing welding fumes. FASEB J 24:4989–5002.
- Stampfer, M. J. 2009. Welding occupations and mortality from Parkinson’s disease and other neurodegenerative diseases among United States men, 1985-1999. J Occup Environ Hyg 6:267–272.
- Steenland, K., Beaumont, J., and Elliot, L. 1991. Lung cancer in mild steel welders. Am J Epidemiol 133:220–229.
- Sung, J. H., Choi, B. G., Maeng, S. H., Kim, S. J., Chung, Y. H., Han, J. H., Song, K. S., Lee, Y. H., Cho, Y. B., Cho, M. H., Kim, K. J., Hyun, J. S., and Yu, I. J. 2004. Recovery from welding-fume-exposure-induced lung fibrosis and pulmonary function changes in Sprague Dawley rats. Toxicol Sci 82:608–613.
- Susi, P., Goldberg, M., Barnes, P., and Stafford, E. 2000. The use of a task-based exposure assessment model (T-BEAM) for assessment of metal fume exposures during welding and thermal cutting. Appl Occup Environ Hyg 15:26–38.
- Tanner, C. M., Ross, G. W., Jewell, S. A., Hauser, R. A., Jankovic, J., Factor, S. A., Bressman, S., Deligtisch, A., Marras, C., Lyons, K. E., Bhudhikanok, G. S., Roucoux, D. F., Meng, C., Abbott, R. D., and Langston, J. W. 2009. Occupation and risk of parkinsonism: a multicenter case-control study. Arch Neurol 66:1106–1113.
- Taylor, M. D., Roberts, J. R., Leonard, S. S., Shi, X., and Antonini, J. M. 2003. Effects of welding fumes of differing composition and solubility on free radical production and acute lung injury and inflammation in rats. Toxicol Sci 75:181–191.
- The James F. Lincoln Arc Welding Foundation. 2000. The Procedure Handbook of Arc Welding, 14th ed. Cleveland, OH: The James F. Lincoln Arc Welding Foundation.
- Tuschl, H., Weber, E., and Kovac, R. 1997. Investigations on immune parameters in welders. J Appl Toxicol 17:377–383.
- Ulrich, M. M., Alink, G. M., Kumarathasan, P., Vincent, R., Boere, A. J., and Cassee, F. R. 2002. Health effects and time course of particulate matter on the cardiopulmonary system in rats with lung inflammation. J Toxicol Environ Health Part A 65:1571–1595.
- U. S. Bureau of Labor Statistics. 2010. ‘51–4121 Welders, Cutters, Solderers, and Brazers’, Occupational Employment and Wages, May 2010 [online].Available online at: http://www.bls.gov/oes/current/oes514121.htm, accessed 2011.
- Vallyathan, V., Goins, M., Lapp, L. N., Pack, D., Leonard, S., Shi, X., and Castranova, V. 2000. Changes in bronchoalveolar lavage indices associated with radiographic classification in coal miners. Am J Respir Crit Care Med 162:958–965.
- Van Loveren, H., Rombout, P. J., Wagenaar, S. S., Walvoort, H. C., and Vos, J. G. 1988. Effects of ozone on the defense to a respiratory Listeria monocytogenes infection in the rat. Suppression of macrophage function and cellular immunity and aggravation of histopathology in lung and liver during infection. Toxicol Appl Pharmacol 94:374–393.
- Yang, M. J., Yang, Y. S., Sung, J. H., Kim, J. S., Cho, K. H., Lim, C. W., Chung, Y. H., Kim, H. Y., Yang, J. S., Yu, I. J., and Song, C. W. 2009. Recurrent exposure to welding fumes induces insufficient recovery from inflammation. Inhal Toxicol 21:337–346.
- Yin, X. J., Schafer, R., Ma, J. Y., Antonini, J. M., Weissman, D. D., Siegel, P. D., Barger, M. W., Roberts, J. R., and Ma, J. K. 2002. Alteration of pulmonary immunity to Listeria monocytogenes by diesel exhaust particles (DEPs). I. Effects of DEPs on early pulmonary responses. Environ Health Perspect 110:1105–1111.
- Yu, I. J., Song, K. S., Maeng, S. H., Kim, S. J., Sung, J. H., Han, J. H., Chung, Y. H., Cho, M. H., Chung, K. H., Han, K. T., Hyun, J. S., and Kim, K. J. 2004. Inflammatory and genotoxic responses during 30-day welding-fume exposure period. Toxicol Lett 154:105–115.
- Zeidler-Erdely, P. C., Battelli, L. A., Salmen-Muniz, R., Li, Z., Erdely, A., Kashon, M. L., Simeonova, P. P., and Antonini, J. M. 2011a. Lung tumor production and tissue metal distribution after exposure to manual metal ARC-stainless steel welding fume in A/J and C57BL/6J mice. J Toxicol Environ Health Part A 74:728–736.
- Zeidler-Erdely, P. C., Battelli, L. A., Stone, S., Chen, B. T., Frazer, D. G., Young, S. H., Erdely, A., Kashon, M. L., Andrews, R., and Antonini, J. M. 2011b. Short-term inhalation of stainless steel welding fume causes sustained lung toxicity but no tumorigenesis in lung tumor susceptible A/J mice. Inhal Toxicol 23:112–120.
- Zeidler-Erdely, P. C., Erdely, A., Stone, S., Kashon, M. L., Li, S. Antonini, J. M. 2011c. Molecular pathways of pulmonary injury following aspiration and inhalation of stainless steel welding fume. The Toxicologist 120:2322.
- Zeidler-Erdely, P. C., Kashon, M. L., Battelli, L. A., Young, S. H., Erdely, A., Roberts, J. R., Reynolds, S. H., and Antonini, J. M. 2008. Pulmonary inflammation and tumor induction in lung tumor susceptible A/J and resistant C57BL/6J mice exposed to welding fume. Part Fibre Toxicol 5:12.
- Zeidler-Erdely, P. C., Kashon, M. L., Li, S., and Antonini, J. M. 2010. Response of the mouse lung transcriptome to welding fume: Effects of stainless and mild steel fumes on lung gene expression in A/J and C57BL/6J mice. Respir Res 11:70.
- Zimmer, A. T. 2002. The influence of metallurgy on the formation of welding aerosols. J Environ Monit 4:628–632.
- Zimmer, A. T. Biswas, P. 2001. Characterization of the aerosols resulting from arc welding processes. J Aerosol Sci 32:993–1008.