Abstract
Vanadium (V) is a transition metal found in air adsorbed onto suspended particles. As a result, urban populations are often exposed to this element as a constituent of particulate matter (PM). One aspect of the myriad toxicities that might arise from these exposures is altered immune responses. Previous reports from the laboratory reported modifications in splenic architecture – with germinal center hyperplasia and a suppressed humoral immune response – in mice that had been exposed to vanadium agents via inhalation. This paper reports a decrease in the presence of the CD11c surface marker on mouse thymic dendritic cells (DC) as a result of host exposure to vanadium (here, in the form of vanadium pentoxide; V2O5) over a period of 4 weeks. All results were obtained using immunohistochemistry and flow cytometry. It is surmised that this decrease might induce a dysfunction, including possible negative selection of T-cells, which could increase the presence of autoreactive clones in the exposed host. Such an outcome could, in turn, increase the risk for development of autoimmune reactions in different organs specifically, and of autoimmune diseases in general in these V-exposed hosts.
Keywords::
Introduction
Vanadium is an element found in suspended particles smaller than 2.5 µm (PST2.5). It is considered an atmospheric pollutant that has (over the last decades) been found in increased concentrations in the atmosphere in cities with heavy motor traffic, such as Mexico City (Fortoul et al., Citation2002). Vanadium is liberated into the atmosphere as a consequence of fossil fuel combustion and metallurgic industrial activities (Hope, Citation1994). Vanadium presence is high in fossil fuels, especially in Mexican petroleum (IPCS, Citation2000; Fortoul et al., Citation2002; Rodriguez-Mercado et al., Citation2006). The pre-eminent compounds liberated after the combustion are oxides, such as vanadium pentoxide (V2O5). This oxide is considered the most toxic vanadium compound in the atmosphere that is adhered to suspended matter (WHO World Health Organization (WHO) Citation1998. Vanadium. In: Environmental Health Criteria 81. Geneva: WHO., Citation1998) and that enters the body primarily via inhalation (Pope et al., Citation1999; Laden et al., Citation2000; Cohen, Citation2004).
Exposure to particulate matter with diameters < 2.5 µm is associated with respiratory disease aggravation and impaired cardiovascular function (Dockery and Pope, Citation1994). Previous reports from our group noted that vanadium can affect other tissues as well, including the blood and nervous systems (Gonzalez-Villalva et al., Citation2006) (Avila-Costa et al., Citation2004). We also have reported that vanadium impairs the immune system, with changes induced in the morphology of the spleen and decreases in humoral immune responses (Piñon-Zarate et al., Citation2008). Taken together, these changes suggested an extensive disorganization of the immune system as a result of vanadium exposure.
Dendritic cells (DC) are professional antigen-presenting cells that also participate in the negative selection of T-lymphocytes and in T-regulatory (Treg) cell induction (Wu and Liu, Citation2007). These cells are regularly distributed into the thymus medulla, and preliminary reports from our group indicated that vanadium inhalation modifies the thymus cortex–medulla relationship in animals exposed to V by inhalation (Fortoul et al., Citation2011). Because of the morphological changes in the thymus that suggests modification in thymocyte selection, we decided to explore the DC expression of CD11c (a thymic-DC biomarker) as well as MHC-II (a biomarker for antigen-presenting cells) to apprise the impact that inhaled vanadium may have upon thymic DC cell distribution.
Materials and methods
Animal selection
A total of 94 CD1 male mice (8-weeks-old, 33 [±2] g) were obtained from the School of Medicine. The mice were housed in groups of 10 in hanging plastic cages maintained in a pathogen-free animal facility (with an average 21°C temperature, 57% humidity, and controlled lighting [i.e., 12:12-h light/dark regimen]). All mice were provided ad libitum access to Purina rat chow and filtered water. The experimental protocol was in accordance with the Animal Act of 1986 for Scientific Procedures.
Mice were separated into two groups: One set of mice (n = 40) was dedicated for use in the immunohistochemistry analysis (i.e., 20 exposed and 20 control), and a second group (n = 54; 27 exposed and 27 control) for use in the flow cytometry analyses.
Exposure protocol
Whole-body inhalation exposure was performed as described in Piñon-Zarate et al. (Citation2008), reproducing our standardized parameters as follows: exposures were performed using a 0.02 M V2O5 (99.99% purity, Sigma, St. Louis, MO) suspension in saline containing 100 µl Tween-20/100 ml of suspension. The aerosol inhalation chamber (an acrylic box measuring 45 cm × 21 cm × 35 cm) with a 3.3 L total volume could house from 20–25 mice/session. A DeVilbiss Ultraneb 99 (Somerset, PA) system was used to nebulize the V2O5 suspension at a constant flow of 10 L/min; according to the manufacturers, ≈ 80% of the aerosolized particles reaching the mice would be expected to have a mass median aerodynamic diameter (MMAD) of 0.5–5.0 µm.
The V2O5 levels in the chamber were quantified as in Fortoul et al. (Citation2002), i.e., a filter was positioned at the external outlet of the ultra-nebulizer during the entire exposure period and samples collected at a flow rate or 10 L/min. After each exposure, the filters were removed and weighed; the element was quantified following the same protocol as with tissue samples. Six filters for each inhalation were evaluated. The final concentration in the chamber was, as expected, 1.4 mg V2O5/m3 (Fortoul et al., Citation2002). As we have reported previously (Gonzalez-Villalva et al., Citation2011), the concentration selected for our experimental model is greater than that in workplaces (ranging from 0.2–0.5 mg/m3) reported by the IARC (Citation2006), but in the range for those noted by the WHO (Citation2000) (i.e., 0.01–60 mg/m3). High vanadium concentrations (> 600 mg/kg) have also been detected in soils in the vicinity of petrochemical plants in Mexico—as a consequence of airborne particulate emissions resulting from high levels of oil/fuel burning (Hernandez and Rodriguez, Citation2011). Thus, we deemed the level of V used here would adequately reflect airborne V exposures that occur routinely among the citizenry of many urban centers, including Mexico City (Fortoul et al. Citation2002), and are also readily encountered in occupational settings.
Immunohistochemistry (IHC)
For the immunohistochemistry analyses, 20 mice were placed in the acrylic box to inhale V2O5 for 1 h/day, twice a week (i.e., on Tuesday and Thursday), for a total of 4 weeks. An additional 20 mice (controls) were exposed only to saline, using the same protocol. Each week, at random, five exposed and five control mice were euthanized by cervical dislocation and their tissues harvested and processed as outlined below.
Thymuses were dissected and then fixed in 4% paraformaldehyde (in phosphate-buffered saline [PBS, pH 7.4]) for 24 h at 4°C. Tissues then underwent a sucrose density gradient from 10–30% at 4°C with changes every 24 h. In a cryostat Leyca CM 1900 (Nussloch, Germany), tissue sections (8-µm thick) were obtained and processed for conventional immunohistochemistry to analyze CD11c expression. Briefly, endogenous peroxidase was blocked with 30% H2O2 for 10 min, followed by three PBS rinses. Sections were then incubated overnight with biotinylated (1:50 dilution) anti-CD11c (Abcam, Catalog item ab11029) in a humidified chamber. The following day, the samples were rinsed three times with PBS, and then incubated for 30 min with streptoavidin peroxidase ready-to-use solution (4plus HRP Universal Detection, Biocare, Concord, CA). Samples were rinsed again with PBS; immunoreactivity was then visualized by a 3-min incubation with diaminobenzidine (DAB) followed by hematoxylin counter-staining (Biocare).
Slides were covered with synthetic mounting resin and observed in a light Olympus CX 31 microscope coupled with a digital camera (Olympus C-7070, Tokyo, Japan). Images were then analyzed using image-analyzer software (Image Pro Plus, Version 6.0, Silver Spring, MD). Five fields from each animal were analyzed, considering as positive the presence of ochre color. Color intensity was reported in pixel units. One-way ANOVA with a Tukey’s post-test was performed to identify differences through exposures time considering results to be statistically significant when p < 0.05 (GraphPad Prism Software, V5, 2007, La Jolla, CA).
Flow cytometry analysis/thymic DC isolation
All exposures were performed on the respective groups as outlined earlier. At the end of the third week of exposures, flow cytometric analyses of CD11c+ and MHC-II+ cells were performed on the 54 mice dedicated for use in this study. For the analyses, there were a total of 27 control and 27 experimental mice. These numbers of mice were required because of the scanty numbers of thymic DC obtained from any one mouse engendered the need to use a method wherein the thymic DC of three mice (within each group) were pooled to obtain enough cells for each test (thereby resulting in the n of 9 [i.e., three groups of three mice each] per group for statistical comparisons of outcomes). The particular (3-week) timepoint was selected based on results collected from previous immunohistochemistry results in preliminary studies in which changes in the thymus were evidenced at week 3 of exposure (see ).
In brief, these mice were euthanized by cervical dislocation, and their thymuses extracted, chopped, and then immersed in PBS at 4°C. The tissue fragments were then digested at 37°C for 60 min in RPMI 1640 containing Type IV collagenase (0.6 mg/ml) and DNase (25 Kuntiz unit/ml) (Baba et al., Citation2009). The resultant tissue suspension was mashed with a syringe plunger to obtain a more dispersed suspension that was, in turn, rinsed twice with PBS at 4°C. These cells were counted in a Neubauer chamber, adjusted to 350 × 106 cells, and then incubated for 15 min at 4°C with anti-MHC-II antibody coupled to magnetic beads 1:9 (Miltenyi Biotec, Bergisch Gladbach, Germany). The beads/column was then rinsed twice with PBS, and the cells were separated via magnetic cell sorting (MACS; Miltenyi). Positive and negative fractions were collected; the positive fraction contained the MCH-II+ thymic cells. A new count was performed on this pool of cells and the population adjusted to 7 × 106/ml in PBS.
Cells were divided into four fractions: cells to be (1) unstained, (2) stained with anti-CD11c APC 1:200 (Biolegend, San Diego, CA; cat. 117310, clone: N418); (3) stained with anti-MHC-II (IA IE) biotinylated 1:300 (BD, San Diego, CA; cat. 223622, clone: 2G9) and latter incubated with streptavidin conjugated with PE-Cy5 1:300 (BD; cat. 554062); or, (4) double-stained with anti-CD11c APC and anti-MHC-II Cy5. Flow cytometry was performed in a FACSCalibur flow cytometer (BD), and the data obtained was analyzed with FlowJo 8.7 software (Tree Star, Inc. Ashland, OR). A minimum of 50,000 events/sample were captured and analyzed.
Results
Immunohistochemistry
CD11c+ cells were observed in both control and exposed animals. The main location of the cells was in the thymic medulla. Comparing control vs exposed mice, the ochre color (diaminobenzidine-developed stain) was more evident in samples from the controls (). Densitometry demonstrated that CD11c expression (ochre color stain) decreased in V-exposed mice; this change became statistically significant during the second week of exposure. The decrease persisted until the end of the experiment ().
Figure 1. Immunohistochemistry staining for CD11c in control and V-exposed mice thymii. CD11c+ ochre color cells (arrows) are observed in thymic medulla (M). The scarcity of these cells in the thymic cortex (Co) is noteworthy. Representative photomicrographs (a) 3-week control; (b) 3-week V-exposed; (c) 4-week control; and (d) 4-week V-exposed. (a) and (c) reveal a higher presence of CD11c+ cells compared to that shown in (b) and (d).
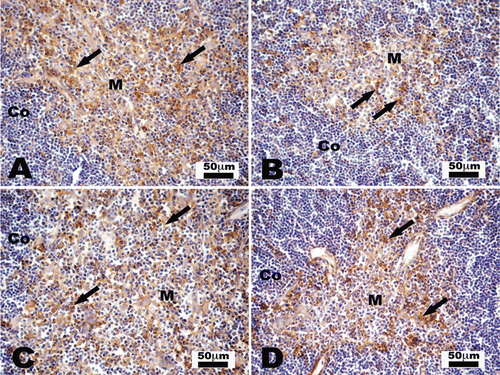
Figure 2. Mean differences in color density when comparing staining results of tissues from V-exposed mice vs those from controls. The reduction in color density is very evident in tissues from exposed mice; this decrease was progressively worsened as the numbers/total amounts of V exposure proceeded. ANOVA (Tukey’s), p < 0.05.
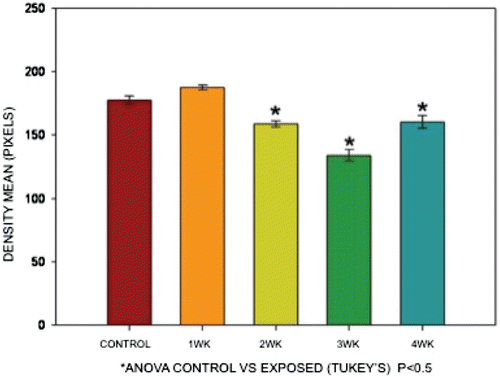
Flow cytometry
Levels of CD11c+ and MHC-II+ cells were significantly decreased in the V-exposed mice as compared with controls. The loss was both in terms of number and in mean fluorescence intensity (MFI) values. The percentages of MFI, MHC-II+, and CD11c+ were significantly higher in controls (p < 0.05) ().
Figure 3. Mean Fluorescence Intensity (MFI) and the percentage of double-positive stained cells in thymic tissues from control and exposed mice. (a) MFI from CD11c+, decreased in exposed mice. ANOVA, p < 0.05. (b) MFI also decreases for MHCII in V-exposed. (c) Percentage of CD11c+ and MHC-II+ double-positive cells showed a significant decrease in exposed mice. ANOVA, p < 0.05.
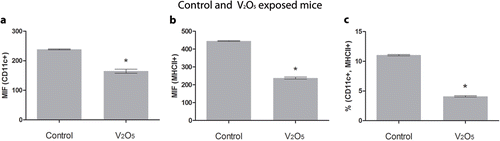
When control dot-plots were compared against those from the V-exposed mice, there was evidence of decreased MFI among CD11c+ cells in the experimental group ( ). The histogram for these mice yielded a shift to the right from that of the controls; this demonstrated a decrease in CD11c expression in the experimental mice. The same results were noted for the MCH-II populations (). Taken together, these results indicated a decrease in the levels of thymic MHC-II+ and CD11c+ cells overall, as well as in the relative expression of these markers on individual cells. It is interesting to note that these changes appeared to be related to the length of exposure as well.
Figure 4. Histograms and dot-plots of CD11c+ and MHC-II+ cells obtained from control and V-exposed mice. (a) Percentage of MFI from a control mouse in CD11c+ and MHC-II+ double-positive cells in 50,000 acquired events. (b) In the V-exposed group the percentage of double-positive cells decreased compared with controls (a). (c) MFI histogram for CD11c+. Basal Isotype control (1), control curve (2) showed a shift to the right compared with the experimental curve (3). The shift to the right indicates that CD11c+ expression is greater in controls compared to V-exposed mice. (D) MFI histogram for MHCII+. Basal Isotype control (1), control curve (2) showed a shift to the right compared with the experimental curve (3). The shift to the right indicates that MHC-II+ expression is greater in controls compared to V-exposed mice.
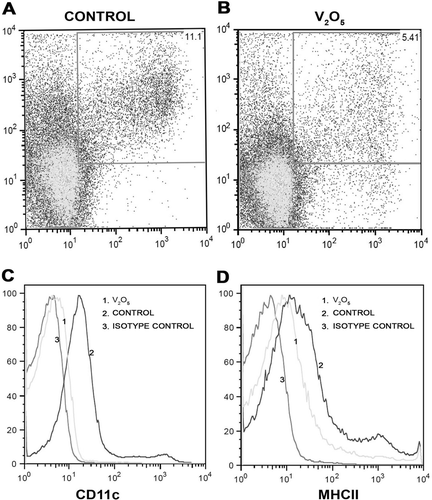
Discussion
The primary route by which particulate matter (PM) gains systemic access is through the respiratory system. Vanadium (V) adsorbed to PM gains access directly into the systemic circulation (Nemmar et al., Citation2004) and exerts effects in a variety of tissues and organs, such as the lung, brain, liver, and spleen (Bonner et al., Citation2000).
We have previously reported that the immune system is compromised in a V-exposed mouse model; in those studies, morphological and functional changes were noted in spleens of mice exposed to V (Fortoul et al., Citation2002; Gonzalez-Villalva et al., Citation2006). Because of the critical participation of the spleen in immune responses/system function, we wondered whether effects would also manifest in another key immune system organ, the thymus. The thymus has a primary role in T-cell selection, and DC within this organ are very active in this selection process (Krueger, Citation2011). In this study, we demonstrated the effect of V inhalation on thymic DC in vivo. Specifically, our study revealed that there was an induced decrease in the number of DC, as well as a decline in the expression of some of their surface markers, i.e., CD11c and MHC-II.
CD11c is a common biomarker for the three thymic DC populations, e.g., the plasmocytoid (pCD), the conventional lymphoid (cCD-lymphoid), and the conventional myeloid (cCD-myeloid) (Li et al., Citation2009). Immunohistochemical analyses showed that the V-exposed mice here had thymic medullae that were thinner than those in the control mice tissues. This finding led us to assume that the number of DC was diminished, as these cells are located in the cortico-medullar and medullar areas (Steinman et al., Citation1996). To verify the reduction, we employed a densitometric analysis of ochre (positive) staining to assess any decreases in CD11c+ cells in the thymus of the exposed mice. We also evaluated whether observed changes were related to the length/degree of total V exposure. We realized that a finding of any exposure-related decreases could be explained by either an outright reduction in the number of positive cells themselves and/or a decrease in the expression of the marker on individual cells. Even with this caveat, the first challenge was to obtain enough thymic DC; we resolved this problem by modifying a method by Baba et al. (Citation2009) and also employing a MACS method. This method allowed DC to be isolated while avoiding a loss of cells due to entrapment in the filters that are commonly employed in other separation techniques. This technique also allowed for the selection of MHC-II+ and CD11c+ cells, an outcome not achieveable by other methods commonly used to separate DC (Varas et al., 2008).
The flow cytometry results confirmed our immunohistochemical findings of decreases in CD11c+ and MHC-II+ DC levels, as well as the decreases in CD11c marker expression/cell. The same outcomes were noted in our IHC analyses. Our results differ from those reported by Chan and Filgueira (Citation2011) that found a discrete effect of V on DC maturation and function with any change in CD11c marker expression. We surmise that the differences compared to our results are because their work was done in vitro while ours was in vivo; specifically, Chan and Filgueira worked with human bone marrow cells (monocytes) that were induced to differentiate into DC before being cultivated with V, while we obtained our cells directly from the thymus of the V-exposed animals.
The decrease in the CD11c expression and the reduced number in DC we report here are in agreement with the findings by Piñon-Zarate et al. (Citation2008) using the same experimental model. Specifically, those authors reported a reduced humoral immune response in animals exposed to V via inhalation. Piñon-Zarate et al. (Citation2008), in a follow-on study, reported spleen germinal center hyperplasia and a reduction in CD19+ B-lymphocytes in V-exposed hosts. In addition, those authors noted that in mice immunized with Hepatitis B surface antigen (HBsAg), there was a decrease in anti-HBsAg antibody production in these exposed animals. DC are critically significant for B-cell responses since these cells directly activate the proliferation of CD40-activated naïve and memory B-cells as well as the differentiation of these cells into antibody-producing cells (Caux et al., Citation1995).
To explain the modification in CD11c presence, we make use of the V potential to induce oxidative stress in our model reported by us and other authors (Gusik et al., Citation2003; Cano-Gutierrez et al., Citation2011). Oxidative stress liberates reactive oxygen (ROS) and nitrogen species (NOS) that may modify pro-inflammatory transcriptional factors and could modify the expression of CD11c. CD11c is an integrin sub-unit and is known also as Axb2, CR4, or leukocyte’s surface antigen p150, 95 (Pribila, Citation2004). This integrin is a heterodimer in which CD11c is the αX chain (ITGAX) that combines with the β2 chain (ITGB2), and constitutes a specific leukocyte integrin (Steinman et al., Citation1996). This integrin can then interact with the cellular matrix (D’Amico et al., Citation1998). A decrease in the presence of the CD11c could modify DC traffic and, in turn, alter thymic function. Another possible consequence of a decrease in CD11c expression could be T-cell dysfunction because this molecule is associated with the presence of CD40 (Pribila et al., 1994) and with MHC-II (Mausberg et al., Citation2009), as noted previously. The reduction in MHC-II+ cells found in our experiments would support this explanation.
Our findings clearly indicate a level of thymic dysfunction after inhalation of vanadium, in particular V2O5. This would be reflected in a host as a disruption in T-cell negative selection that, in turn, would then alter the interaction of CD4 lymphocytes with the MHC-II protein on DC. Such changes could then favor the development of autoimmune responses as suggested by Chang (Citation2010) and indirectly by Zouali (Citation2011), who hypothesized that an epigenetic effect from urban air pollutants led to altered immune cell phenotypes that, in turn, favored development of autoimmunity. Further studies are planned to investigate this topic in greater detail.
Conclusions
Vanadium inhalation modified CD11c and MHC-II expression in thymic dendritic cells (DC). As summarized in , the results presented here support our long-standing hypothesis that air pollutants, especially particulate matter, are capable of inducing changes in the systemic immune response that could be used to explain the increase in a variety of autoimmune-related pathologies—such as lupus, rheumatoid arthritis, and other diseases—that have not yet been clearly shown to be associated with other environmental factors (Fortoul et al., Citation2002, Fortoul and Rojas-Lemus, Citation2007). The master key in this immunological disruption would be the changes in DC interaction with T-cells, reducing the strict surveillance for T-cell selection, allowing autoreactive cells to enter into the systemic circulation, resulting in an increase in the potential for autoimmune disease development.
Figure 5. A schematic description of the proposed effect of vanadium on dendritic cells (DC). The decreases in the expression of CD11c and the MHC-II markers demonstrated in this report would likely result in CD40 reductions and in B-cell proliferation (as suggested by Caux et al., 1994), as well as an increase in the levels/numbers of autoreactive T-cell clones in the host (as suggested by Chang, Citation2010).
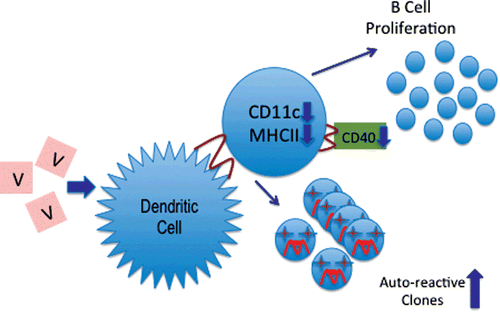
Acknowledgments
The authors are grateful to Diana Millan and Francisco Pasos, for their technical assistance. The first author gives thanks for the support given by the Posgrado en Ciencias Biológicas, UNAM.
Declaration of interest
The authors report no conflicts of interest. The authors alone are responsible for the content and writing of the paper.
References
- Avila-Costa, M. R., Montiel-Flores, E., Colin-Barenque, L., Ordoñez, J. L., Gutierrez, A. L., Niño-Cabrera, H. G., Mussali-Galante, P.Fortoul, T. I. 2004. Nigrostriatal modifications after vanadium inhalation: An immunocytochemical and cytological approach. Neurochem. Res. 29:1365–1369.
- Baba, T., Nakamoto, Y.Mukaida, N. 30632009. Crucial contribution of thymic sirpα+ conventional dendritic cells to central tolerance against blood-borne antigens in a CCR-dependent manner. J. Immunol. 183:3053–3063.
- Bonner, J. C., Rice, A. B., Moomaw, C. R.Morgan, D. L. 2000. Airway fibrosis in rats induced by vanadium pentoxide. Am. J. Physiol. 278:L209–L216.
- Cano-Gutierrez, G., Acevedo-Nava, S., Santamaria, A., Altamirano-Lozano, M., Cano-Rodriguez, M. C.Fortoul, T. I. 2011. Hepatic megalocytosis due to vanadium inhalation: Participation of oxidative stress. Toxicol. Ind. Health (doi 10.1177/0748233711412424).
- Caux, C., Massacrier, C., Vanbervliet, B., Dubois, B., Kooten, C., Durand, I.Banchereau, J. 1995. Activation of human dendritic cells through CD40 cross-linking. J. Exp. Med. 180:1263–1272.
- Chan, E.Filgueira, L. 2011. Effect of vanadium on human dendritic cells. Toxicol. Environ. Chem. 92:355–371.
- Chang, C. 2010. The immune effects of naturally occurring and synthetic nanoparticles. J. Autoimmun. 34:J234–J246.
- Cohen, M. D. 2004. Pulmonary immunotoxicology of select metals: Aluminum, arsenic, cadmium, chromium, copper, manganese, nickel, vanadium, and zinc. J. Immunotoxicol. 1:39–70.
- D’Amico, G., Bianchi, G., Bernasconi, S., Bersani, L., Piemonti, L., Sozzani, S., Mantovani, A.Allavena, P. 1998. Adhesion, trans-endothelial migration, and reverse transmigration of in vitro cultured dendritic cells. Blood 92:207–214.
- Dockery, D. W.Pope, C. A., 3 rd. 1994. Acute respiratory effects of particulate air pollution. Annu. Rev. Publ. Health. 15:107–132.
- Fortoul, T. I.Rojas-Lemus, M. 2007. Vanadium as an air pollutant. In: Vanadium: its impact on health (Fortoul, T. I.Avila Costa, M. R., Eds.). New York: Nova Science Publishers, pp. 1–6.
- Fortoul, T. I., Quan-Torres, A., Sanchez, I., Lopez, I. E., Bizarro, P., Mendoza, M. L., Osorio, L. S., Espejel-Maya, G., Avila-Casado, M. del C., Avila-Costa, M. R., Colin-Barenque, L., Villanueva, D. N.Olaiz-Fernandez, G. 2002. Vanadium in ambient air: Concentrations in lung tissue from autopsies of Mexico City residents in the 1960s and 1990s. Arch. Environ. Health. 57:446–449.
- Fortoul, T. I., Rodriguez-Lara, V., Gonzalez-Villalva, A., Rojas-Lemus, M., Cano-Gutierrez, G., Ustarroz-Cano, M., Colin-Barenque, L., Montaño, L. F., Garcia-Pelaez, I., Bizarro-Nevares, P., Lopez-Valdez, N., Falcon-Rodriguez, C. I., Jimenez-Martinez, R. S., Ruiz Guerrero, M. L., Lopez-Zepeda, L. S., Morales-Rivero, A.Muñiz-Rivera-Cambas, A. 2011. Vanadium inhalation in a mouse model for the understanding or air-suspended particle systemic repercussion. J. Biomed. Biotechnol. 2011: 951043.
- Gonzalez Villalva, A., Fortoul, T. I., Avila-Acosta, M. R., Piñon-Zarate, G., Rodriguez-Lara, V., Martinez-Levy, G., Rojas-Lemus, M., Bizarro-Nevares, P., Diaz-Bech, P., Mussali-Galante, P.Colin Barenque, L. 2006. Thrombocytosis induced in mice after subacute and sub-chronic V2O5 inhalation. Toxicol. Ind. Health. 22:113–116.
- Gonzalez-Villalva, A., Piñón-Zárate, G., De la Peña-Diaz, A., Flores-Garcia, M., Bizarro-Nevares, P., Rendon-Huerta, E., Colin-Barenque, L.Fortoul, T. I. 2011. The effect of vanadium on platelet function. Environ. Toxicol.Pharmacol. 32:447–456.
- Gusik, T. J., Korbut, R.Adame-Gusik, T. 2003. Nitric oxide and superoxide in inflammation and immune regulation. J. Physiol. Pharmacol. 54:469–487.
- Hernandez, H.Rodriguez, R. 2011. Geochemical evidence for the origin of vanadium in an urban environment. Environ. Monit. Assess. doi: 10.1007/s10661-011–2343–9.
- Hope, B. K. 1994. A global biogeochemical budget for vanadium. Sci. Total Environ. 141:1–10.
- IARC (International Agency for Research on Cancer. 2006. Vanadium pentoxide. IARC Monogr. Eval. Carcinog. Risks Hum. 86:227–292.
- IPCS, International Programme on Chemical Safety. 2000. Concise international chemical assessment: document. vanadium pentoxide and other inorganic vanadium compounds. Geneva: World Health Organization (WHO).
- Krueger, A. 2011. A missing link in thymic dendritic cell development. Eur. J. Immunol. 41:2141–2147.
- Laden, F., Neas, L. M., Dockery, D. W.Schwartz, J. 2000. Association of fine particulate matter from different source with daily mortality in six U.S. cities. Environ. Health Perspect. 108:941–947.
- Li, C., Park, J., Foss, D.Goldschneider, I. 2009. Thymus-homing peripheral dendritic cells constitute two of the three major subsets of dendritic cells in the steady-state thymus. J. Exp. Med. 206:607–622.
- Mausberg, A. K., Jander, S.Reichmann, G. 2009. Intra-cerebral granulocyte-macrophage-colony stimulating factor induces functionally competent dendritic cells in the mouse brain. Glia 57:1341–1350.
- Nemmar, A., Hoylaerts, M. F., Hoet, P. H.Nemery, B. 2004. Possible mechanisms of the cardiovascular effects of inhaled particles: Systemic translocation and pro-thrombotic effects. Toxicol. Lett. 149:243–253.
- Piñon-Zarate, G., Rodriguez-Lara, V., Rojas-Lemus, M., Martinez-Pedraza, M., Gonzalez Villalva, A., Mussali-Galante, P.Fortoul, T. 2008. Vanadium pentoxide inhalation pro-vokes germinal center hyperplasia and suppressed humoral immune responses. J. Immunotoxicol. 5:115–122.
- Pope, M., Ashley, M. J.Ferrence, R. 1999. The carcinogenic and toxic effects of tobacco smoke: Are women particularly susceptible? J. Gend. Specif. Med. 2:45–51.
- Pribila, J., Itano, A., Mueller, K.Shimizu, Y. 2004. The α1β1 and α1β1 integrins define a subset of dendritic cells in peripheral lymph nodes with unique adhesive and antigen uptake properties. J. Immunol. 172:282–291.
- Rodriguez-Mercado, J. J.Altamirano-Lozano, M. A. 2006. Vanadium: Contamination, metabolism, and genotoxicity. Rev. Int. Contam. Ambient. 22:173–189.
- Steinman, R. M., Pack, M.Inaba, K. 1996. Dendritic cells in the T-cell areas of lymphoid organs. Immunol. Rev. 156:25–37.
- Varas, A., Hernandez-Lopez, C., Valencia, J., Mattavelli, S., Martinez, V. G., Hidalgo, L., Gutierrez-Frias, C., Zapata, A.G., Sacedon, R. Vicente, A. 2009. Survival and function of human thymic dendritic cells are dependent on autocrine Hedgehog signaling. J. Leukocyte Biol. 83:1476–1483.
- World Health Organization (WHO) 1998. Vanadium. In: Environmental Health Criteria 81. Geneva: WHO.
- World Health Organization (WHO). Regional Office for Europe 2000. Vanadium. In: WHO Regional Publications, European series No. 91. Copenhagen: WHO.
- Wu, L.Liu, Y. J. 2007. Development of dendritic-cell lineages. Immunity 26:741–750.
- Zouali, M.. 2011. Epigenetics in lupus. Ann. N. Y. Acad. Sci. 1217:154–165.