Abstract
Warfarin is a Vitamin K (VK) antagonist that affects Vitamin K-dependent (VKD) processes, including blood coagulation, as well as processes unrelated to hemostasis such as bone growth, calcification, and growth of some cell types. In addition, warfarin exerts influence on some non-VKD-related activities, including anti-tumor and immunomodulating activity. With respect to the latter, both immune stimulating and suppressive effects have been noted in different experimental systems. To explore the in vivo immunomodulatory potential of warfarin on one type of activity (i.e., cytokine production) in two different immune cell populations (i.e., mononuclear or polymorphonuclear cells), effects of subchronic oral warfarin intake in rats on pro-inflammatory cytokine (i.e., TNFα, IL-6) production by peripheral blood mononuclear and polymorphonuclear cells (granulocytes) was examined. Differential effects of warfarin intake on TNFα and IL-6 were noted, depending on the type of peripheral blood leukocytes and on the cytokine examined. Specifically, a lack of effect on TNFα and a priming of IL-6 production by mononuclear cells along with a decrease in TNFα and a lack of effect on IL-6 in polymorphonuclear cells were seen in warfarin-exposed hosts. The cell- and cytokine-dependent effects from subchronic oral warfarin intake on peripheral blood leukocytes demonstrated in this study could, possibly, differentially affect reactions mediated by these cells. Ultimately, the observed effects in rats might have implications for those humans who are on long-term/prolonged warfarin therapy.
Introduction
Warfarin (4-OH coumarin) and its analogs are Vitamin K (VK) antagonists that inhibit the Vitamin K-dependent (VKD) step in the complete synthesis of a number of blood coagulation factors required for normal blood coagulation (Shearer, Citation1990). Impairment of production of these essential VKD blood clotting factors by coumarin anti-coagulants results in an increase in clotting time up to the point where no clotting occurs. Anti-coagulant effects of warfarin and its congeners that, along with small blood vessels damage, generally result in death due to internal hemorrhage, provides the basis for their use as anti-coagulant rodenticides (Lund, Citation1988). Anti-coagulants of the 4-hydroxy-coumarin type are also used in prophylactic medicine to prevent thromboembolic diseases in at-risk patients (Furie, Citation2000).
Because of its importance as a drug and as a rodenticide, the mechanisms of anti-coagulant action of warfarin have been extensively studied. Warfarin inhibits Vitamin K epoxide reductase (VKOR), in turn, giving rise to a rapid depletion of hydroquinone (K1H2), a co-factor for the γ-glutamyl carboxylase enzyme that mediates carboxylation of glutamyl (Gla) residues on precursors of several VKD proteins involved in coagulation. A reduced supply of this co-factor form of VK results in an accumulation of under-carboxylated (inactive) forms of Factors II (FII, prothrombin), VII (FVII), IX (FIX), and X (FX) (Furie, Citation2000).
Inhibition of VKOR by warfarin also affects the catalytic rate of VKD proteins required for biological processes other than hemostasis, including proteins required for the regulation of bone growth and calcification (bone Gla protein, BGP/osteocalcin, and matrix Gla protein, MGP) (Price, Citation1988; Becker, Citation2007). The effects of warfarin on BGP and MGP are considered responsible for ‘warfarin embryopathy’, a developmental defect resulting from warfarin consumption during pregnancy, as well as for bone mass loss in patients on long-term anti-coagulant therapy (WHO, 1995). Suppression of MGP is a possible determinant in arterial calcification induced by warfarin consumption in rats (Howe and Webster, Citation2000) and humans (Becker, Citation2007). As warfarin also affects VKD proteins involved in growth of vascular smooth muscle cells (Nakano et al., Citation1997) and mesangial cells (Yanagita et al., Citation1999), this implies broader effects of this anti-coagulant.
Indeed, warfarin effects on processes unrelated to Vitamin K have been demonstrated and include anti-tumor and immunomodulating activities. Inhibition of proliferation of some tumor cells by warfarin, as well as potentiation of natural killer (NK) cell activity in patients on warfarin therapy (Bobek et al., Citation2005), formed the basis for its use as an adjuvant anti-tumor therapy in humans (Bobek and Kovarik, Citation2004; Nakchbandi et al., Citation2006). Concerning warfarin action on immune system, conflicting data have been reported. Early studies in humans showed an inhibition of the development of skin indurations in delayed-hypersensitivity tests by warfarin at doses that induce an anti-coagulant effect (Edwards and Rickles, Citation1978). In contrast, warfarin therapy resulted in an enhancement of lectin (PHA)-induced proliferation of peripheral blood mononuclear cells in some patients (Berkarda et al., Citation1983). Similar data were obtained in mice wherein both stimulatory (Berkarda et al., Citation1978) and suppressive (Eichbaum et al., Citation1979; Perez et al., Citation1994; Kurohara et al., Citation2008) effects of warfarin on the immune system were noted.
Our earlier data showed a pro-inflammatory activity of warfarin that gained access to the skin of rats. This included an increased capacity of epidermal cells to stimulate T-cell activation and/or proliferation (Kataranovski et al., Citation2005) and to produce tumor necrosis factor (TNF)-α (Popov et al., Citation2011), as well as T-cell tropism for warfarin-treated skin (Kataranovski et al., Citation2007a; Popov et al., Citation2011). Peripheral blood granulocyte priming for respiratory burst activity and increased adhesion (Kataranovski et al., Citation2003, Citation2008) were indicative of a percutaneous pro-inflammatory potential resulting from acute warfarin administration as well. However, a concomitant decrease in skin-draining lymph node responses to contact allergen (Kataranovski et al., Citation2003) illustrated the potential suppressive effects of warfarin. These and the above-cited human and animal data imply that there are a variety of effects of warfarin on immune cells and their functions. Differential effects of warfarin might rely on differences in the type of cells and/or immune activity examined. There are some indications from in vitro data that warfarin might even exert differential effects on the same type of cell (Kater et al., Citation2002).
In the view of these findings, the aim of the present study was to obtain information concerning immunomodulatory effects of warfarin by examining the same type of activity (i.e., cytokine production) in different cell populations (i.e., mononuclear or polymorphonuclear cells). To achieve this aim, the effect of subchronic oral warfarin intake in rats on pro-inflammatory cytokine (TNFα and interleukin [IL]-6) production by peripheral blood mononuclear and polymorphonuclear cells (granulocytes) was examined.
Materials and methods
Chemicals
Warfarin sodium was purchased from Serva Fein Biochemica (Heidelberg, Germany). 3-(4,5-Dimethyl-thiazol-2-yl)-2,5 diphenyltetrazolium bromide (MTT), lipopolysaccharide (LPS, Escherichia coli Type 0111:B4) were purchased from Sigma (St. Louis, MO). LPS was dissolved in culture medium under sterile conditions. MTT was dissolved in phosphate-buffered saline (PBS, pH 7.4) and was sterile filtered (Minisart, pore size 0.20 µm; Sartorius Stedim biotech, Goettingen, Germany) before use. Dextrane T500 was purchased from Pharmacia (Fine Chemicals AB, Uppsala, Sweden) and dissolved in non-pyrogenic sterile physiological saline (6% [w/v]). RPMI 1640 medium (PAA Laboratories, Pasching, Austria) supplemented with 2 mM glutamine, 20 µg/ml gentamycin (Galenika a.d., Zemun, Serbia), and 5% (v/v) heat-inactivated fetal calf serum (PAA Laboratories) was used in cell culture experiments.
Animals and warfarin treatment
Male Dark Agouti (DA) rats (12–16-weeks-old, 200–230 g), conventionally housed at the Institute for Biological Research ‘Siniša Stanković’ (IBISS, Belgrade, Serbia), were used in these experiments. Housing was in a facility maintained at 20–24°C, with 60% relative humidity and a 12-h light/dark cycle. All rats had ad libitum access to standard rodent chow and filtered water (the latter prior to the exposures, see below). All animal protocols/treatments were carried out in adherence to the guidelines of/and with the approval of the Institute Ethical Committee.
At least two independent experiments were conducted with rats randomly assigned to each treatment group (at 4–6 rats/timepoint/treatment, two rats/cage). Warfarin sodium was prepared in the rat drinking water at 3.5 mg/L and was given to rats ad libitum for 30 days (with replacement by freshly-prepared solution twice a week). Control rats were given drinking water only (warfarin intake duration = 0 days). Seven, 15, and 30 days following the start of warfarin consumption, the rats were euthanized by an over-anesthetization of thiopental sodium (Rotex-medica, Tritau, Germany) given by intraperitoneal (IP) injection (40 mg/kg body weight), and blood was withdrawn from the abdominal artery into heparinized tubes (for leukocyte isolation) or in tubes which contained 3.8% (w/v) sodium citrate (final blood to citrate ratio 5:1) for PT and PTT measurements.
Clinical biochemistry
Prothrombin (PT) and partial thromboplastin time (PTT) were determined in blood samples diluted in citrate buffer (5:1) by a one-stage method using citrate plasma and Thromborel S reagents (Behring Diagnostics GmbH, Marburg, Germany), with Siemens equipment. Kaolin-activated PTT (APTT) was determined by a one-stage method using Pathromtin SL reagent (Behring Diagnostics).
Peripheral blood leukocyte counts
Total leukocyte counts were determined using an improved Neubauer hemocytometer (Neubauer, Wertheim, Germany). Differential leukocyte counts were determined by examining at least 300 cells in air-dried whole-blood smears that had been stained according to a standard May Grünwald-Giemsa (MGG) protocol used in our laboratory (Kataranovski et al., Citation2008).
Histology
Lungs, liver, kidneys, and skin were taken 30 days following the start of warfarin intake. Each tissue sample was fixed in 4% buffered formalin (pH 6.9), embedded in paraffin wax, sectioned at 5 μm, and stained with hematoxylin/eosin (H&E) solution. Samples were then examined by a certified histopathologist in a blinded manner using a Coolscope digital light microscope (Nikon Co, Tokyo, Japan).
Peripheral blood mononuclear and polymorphonuclear cells isolation
Cells were isolated from heparinized blood by dextran sedimentation/centrifugation over OptiPrep (Nycomed AS, Oslo, Norway) density separation medium. Mononuclear cells were harvested from the band formed at the interface of plasma and OptiPrep medium. Polymorphonuclear cells (granulocytes) were obtained from the pellet fraction following lysis of erythrocytes with isotonic 0.84% (w/v) NH4Cl solution. Trypan blue dye exclusion revealed low numbers of dead cells as a result of this procedure. The purity of cells, determined morphologically after MGG staining, was ≈ 100% and > 95% for mononuclear cells and granulocytes, respectively.
A quantitative colorimetric assay described for human granulocytes (Oez et al., Citation1990), the MTT assay (which is based on reduction of tetrazolium salt MTT to formazan by mitochondrial dehydrogenases) was used to assess granulocyte metabolic viability. Briefly, granulocytes were added to wells of a 96-well plate (0.25 × 106 cells/well) and incubated with 500 µg/ml of MTT (added immediately or following 24 h of culture) for 3 h at 37°C. Formazan produced by the cells was then dissolved by overnight incubation following addition of 100 µl of 10% sodium dodecyl sulfate (SDS)/0.01 N HCl solution. The absorbance in each well was then measured at OD 540/650 nm using an ELISA 96-well plate reader (GDV EC, Rome, Italy). Cell survival following 24 h in culture (i.e., survival index) was calculated as the ratio of MTT reduction by cultured granulocytes vsthat by freshly-isolated cells.
Peripheral blood mononuclear and granulocyte cell culture to assess cytokine formation
For assessments of cytokine production, 5 × 105 cells/well (in 96-well plate) were placed in culture for 48 h in wells that had received the addition of 200 µl of medium only (spontaneous production) or with 100 ng LPS/ml (stimulated production).
Cytokine determination by ELISA
Tumor necrosis factor (TNF)-α and interleukin (IL)-6 concentrations in granulocyte- or mononuclear-conditioned medium were evaluated using enzyme-linked immunosorbent assays (ELISA) for rat TNFα (eBioscience Inc., San Diego, CA) and rat IL-6 (R&D systems, Minneapolis, MN), according to manufacturer instructions. Cytokine titer was calculated by the extrapolation from a standard curve constructed with known amounts of kit-provided recombinant cytokines. The limit of detection for the TNFα and IL-6 kits were 16 and 21 pg/ml, respectively.
Reverse transcription—real-time polymerase chain reaction (RT-PCR)
Total RNA was isolated from the mononuclear cells and granulocytes immediately after isolation with an RNA Isolator (Metabion, Martinsried, Germany) following the manufacturer’s instructions. The isolated RNA was reverse-transcribed using random hexamer primers and MMLV (Moloney Murine Leukemia Virus) reverse transcriptase, according to the manufacturer’s instructions (Fermentas, Vilnius, Lithuania). Prepared cDNAs were amplified by using Power SYBR® Green PCR Master Mix (Applied Biosystems, Foster City, CA) according to the recommendations of the manufacturer in a total volume of 20 µl in an ABI PRISM 7000 Sequence Detection System (Applied Biosystems). Thermocycler conditions comprised an initial step at 50°C for 5 min, followed by a step at 95°C for 10 min, and subsequent 2-step PCR program at 95°C for 15 s and 60°C for 60 s for 40 cycles. The PCR primers were as follows: β-actin forward 5′-CCCTGGCTCCTAGCACCAT-3′, backward 5′-GAGCCACCAATCCACACAGA-3′; TNFα forward 5′-TCGAGTGACAAGCCCGTAGC-3′, backward: 5′-CTCAGCCACTCCAGCTGCTC-3′; IL-6 forward 5′-GCCCTTCAGGAACAGCTATGA-3′, reverse 5′-TGTCAACAACATCAGTCCCAAG-3′. Accumulation of PCR products was detected in real time and the results were analyzed with 7500 System Software (Applied Biosystems) and calculated as 2–ΔCt, where ΔCt was the difference between Ct values of a specific gene and the endogenous control (β-actin).
Data display and statistical analysis
Results are expressed as mean ± SD. All statistical analyses were performed using STATISTICA 7.0 software (StatSoft Inc., Tulsa, OK). Statistical significance was defined by a Mann-Whitney U-test (cell numbers, cytokine production) and t-test (cytokine gene expression). p-values < 0.05 were considered significant.
Results
Relevance of dose selection for use in the study
The average warfarin dose that rats consumed here was 0.4 mg/kg. This value was derived from the warfarin concentration in water (i.e., 3.5 mg/L) and the average water volume consumed per rat (i.e., 25 ml/day) each day. In this way, the rats introduced 0.0875 mg into a body mass of 215 g (average weight) each day; this equates to 0.4 mg/kg. According to the formula for calculation of Human Equivalent Dose, HED (Reagan-Shaw et al., Citation2007), the HED (in mg/kg) is equal to the Animal dose (mg/kg) multiplied by the [Animal Km (i.e., 6)/the Human Km (i.e., 37)]. As such, the dose that the rats consumed daily corresponded to 0.06 mg/kg, a value that is on par with a level of 3.6 mg/60 kg that literature reports have indicated have been a warfarin dose for humans (actually ranged from 2–5 mg per average weight person) (Hirsh et al., Citation1998).
Anti-coagulant effects of oral warfarin intake
Rats were given warfarin in drinking water at 3.5 mg/L. Every individual rat consumed 20–30 ml of warfarin solution or water daily; there were no noted changes in liquid consumption during the entire period of intake. In this way, the rats consumed 250 [± 20] µM warfarin daily, a value that corresponds to 360 [± 50] µg warfarin/kg, a dose shown previously to modulate leukocyte activity in an acute exposure regimen (Kataranovski et al., Citation2003, Citation2008). Increases in the mean PT and PTT were noted as early as after 7 days of warfarin administration, with the numerically highest values after 30 days of warfarin consumption (). During that time period, external (skin) and internal (gastrointestinal) hemorrhages were noted in some of the rats (data not shown).
Figure 1. Anti-coagulant effects of oral warfarin intake. Prothrombin (PT) and partial thromboplastin time (PTT) were determined in rat blood collected at 0, 7, 15, and 30 days following warfarin (3.5 mg/L) consumption. Data are presented as mean ± SD from at least two independent experiments, with 4–6 rats/timepoint/treatment. Value significantly different from control (warfarin intake duration = 0 days) at **p < 0.01 or ***p < 0.001.
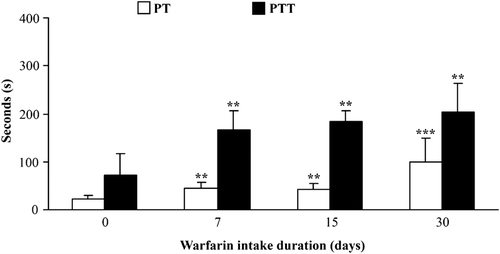
Peripheral blood leukocyte numbers
No changes were noted in total numbers of peripheral blood leukocytes during the entire period of warfarin intake. Thirty days following the start of warfarin consumption, a decrease in the relative percentages (and thus, numbers) of polymorphonuclear cell relative numbers was seen. This outcome was concomitant with a trend (p = 0.07) toward an increase in relative percentages (i.e., numbers) of lymphocytes and a significant increase in relative percentages (i.e., numbers) of monocytes ().
Table 1. Total and differential peripheral blood cell counts in rat that consumed warfarin.
Histology
Histologic analyses of the lungs (as an organ that harbors a large intravascular reservoir of leukocytes both under basal and inflammatory conditions) revealed blood vessel congestion, as well as margination and extravasation of neutrophils that could be found in a widened interstitium, in rats that consumed warfarin (). Signs of recent bleeding were also noted in these rats. Control rat lungs did not present with any of these types of pathologies. In contrast, compared to control host tissues, no striking changes were noted in the liver, kidneys, or skin samples from the warfarin-exposed rats.
Figure 2. Histology of lungs of rats that consumed warfarin. Representative samples from treated and control rats after 30 days of exposure. (a) Widened lung interstitium. Lungs of control rats are shown in insert. (b) Margination and transendothelial migration of neutrophils (arrows). (c) Neutrophils in interstitium (arrows). (d) Fresh erythrocytes and liquid in alveoli.
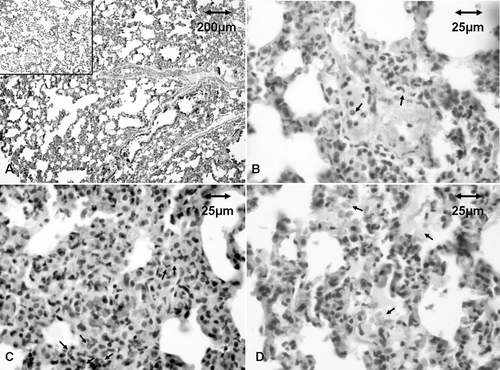
Effect of warfarin intake on peripheral blood mononuclear and polymorphonuclear cell TNFα and IL-6 production and expression
No changes were noted either in spontaneous or LPS-stimulated TNFα production by mononuclear cells during the entire period of warfarin intake (). In contrast, a decrease in both spontaneous and stimulated TNFα production by granulocytes was noted 30 days following the start of warfarin consumption (). This decrease seemed to not be associated with changes in granulocyte metabolic viability, as similar levels of MTT reduction (OD 540 nm; data not presented as figure/table) were noted both in freshly-isolated cells from control (0.31 ± 0.03) and 30-day warfarin-treated (0.32 ± 0.02) rats or following 24 h in culture (0.19 [± 0.01] and 0.17 [± 0.01]) in cells from the respective experimental groups); these outcomes yielded cell survival indices of 0.60 ± 0.02 and 0.55 ± 0.05, respectively, for the granulocytes from controls and rats that consumed warfarin for 30 days. In addition, to correlate any changes in ELISA-measurable cytokine TNFα production with gene expression, mRNA levels were assessed in mononuclear cells and granulocytes from these same 30-day warfarin rats (). As revealed by RT-PCR, similar levels of mRNA for TNFα were noted in mononuclear cells recovered from both control and warfarin-treated rats ); conversely, lower levels of message for TNFα were found with polymorphonuclear cells obtained from the warfarin rats.
Figure 3. Effect of warfarin intake on peripheral blood leukocyte TNFα production and expression. Spontaneous (LPS, 0 ng/ml) and stimulated (100 ng LPS/ml) TNFα production was analyzed in conditioned medium of (a) mononuclear and (b) polymorphonuclear cells isolated from peripheral blood of rats that did not consume warfarin (control, warfarin intake duration = 0 days) and rats that consumed warfarin (3.5 mg/L) for 7, 15, or 30 days. (c) TNFα mRNA expression was analyzed in both cell types isolated from rat blood 0 and 30 days after start of warfarin consumption. Data are presented as mean ± SD (for cytokine production) and mean ± SE (for mRNA expression) from two independent experiments, with 4–6 rats/timepoint/treatment. Value significantly different from control (warfarin intake duration = 0 days) at *p < 0.05 or **p < 0.01.
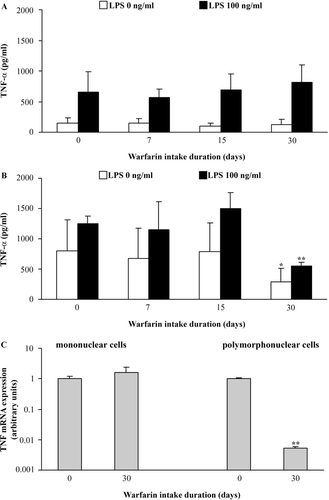
When the dynamics of peripheral blood mononuclear cell IL-6 production was examined, no changes were noted in spontaneous production of this cytokine during the entire period of warfarin consumption. Stimulation with LPS resulted in higher levels of mononuclear cell IL-6 production, compared to those in control rats, during the entire period of warfarin intake, with the highest levels 30 days after the start of consumption (p < 0.05 vs Days 7 and 15) (). In contrast, no changes in either spontaneous or LPS-stimulated IL-6 production by polymorphonuclear cells were observed (). Measurements of IL-6 mRNA in mononuclear cells and granulocytes isolated from hosts after 30 days of warfarin consumption revealed no differences between control and warfarin-treated rats (not shown).
Figure 4. Effect of warfarin intake on peripheral blood leukocyte IL-6 production and expression. Spontaneous (LPS 0 ng/ml) and stimulated (100 ng LPS/ml) IL-6 production was analyzed in conditioned medium of (a) mononuclear and (b) polymorphonuclear cells isolated from peripheral blood of rats that did not consume warfarin (control, warfarin intake duration = 0 days) and rats that consumed warfarin (3.5 mg/L) for 7, 15, or 30 days. Data are presented as mean ± SD from at least two independent experiments, with 4–6 rats/timepoint/treatment. Value significantly different from: control (warfarin intake duration = 0 days) (*p < 0.05); values for warfarin intake duration = 7 days (• p < 0.05), and values for warfarin intake duration = 15 days (# p < 0.05).
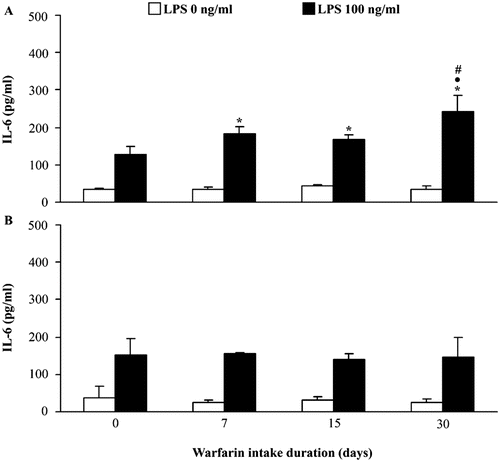
Discussion
In this study, the effect of subchronic (30-day) oral warfarin intake on peripheral blood leukocyte pro-inflammatory cytokine (i.e., TNFα and IL-6) production in rats was examined. The advantage of large quantities of cells that could be obtained from the blood of rats (not as easily achievable in mice) is what permitted us to readily perform peripheral blood cell isolation/separation and cytokine production studies. Differential effects from warfarin intake on peripheral blood leukocyte TNFα production were observed at the end of a 30-day period (without changes in mononuclear cell levels and a decrease in those of polymorphonuclear cells). Conversely, increased LPS-stimulated production of IL-6 by mononuclear cells and a lack of effect on granulocyte production of IL-6 were noted in rats that had consumed the warfarin.
The observed increases in PT and PTT that started 7 days after the start of warfarin consumption demonstrated the anti-coagulant effect, i.e., the basic biological activity of this agent. The appearance of internal as well as external hemorrhage in some animals at the end of 30 days of warfarin intake was in concordance with data that showed that application of warfarin as a rodenticide is associated with impaired coagulation and with hemorrhage (as a delayed effect), the pathology that ultimately results in death of rodents (Kataranovski et al., Citation1988; Lund, Citation1988).
Warfarin consumption was associated with quantitative changes in peripheral blood leukocytes at the end of the 30-day period of intake. The decrease in granulocyte numbers in the peripheral blood of rats at this timepoint could not be ascribed to warfarin cytotoxicity, as there was a lack of change in viability of freshly-isolated granulocytes and their survival following 24 h in culture. A decrease in granulocyte numbers and an increase in numbers of mononuclear cells here demonstrated the differential effects of warfarin on quantitative parameters of circulating leukocytes. The mechanisms of these disparate effects on blood leukocytes are at present not known and might, possibly, reflect differences in warfarin effects on bone marrow. However, we cannot rule out that there might also be differences in peripheral blood leukocyte redistribution patterns as well. Indeed, margination and neutrophil extravasation—as well as their presence in the lung interstitium—indicate an infiltration of these types of cells into this tissue. Lungs harbor intravascular reservoirs of leukocytes, predominantly neutrophils (termed ‘marginated pool’) that constantly exchange with circulating neutrophils (Kuebler, Citation2005).
Beside different quantitative effects, warfarin intake exerted differential qualitative changes in peripheral blood leukocytes (i.e., cytokine production) as well. A decrease in TNFα production by granulocytes from rats that consumed warfarin appeared to have resulted from inhibition at the transcriptional level, as lower levels of TNFα mRNA were noted in these cells. In line with the previously-observed inhibition of transduction of a signal generated by TNFα in a macrophage cell line by warfarin in vitro (Kater et al., Citation2002), the decreases in TNFα expression and production imply that TNFα is a target of warfarin action in phagocytic cells. The lack of the effect on mononuclear cell TNFα expression/production corroborates such an assumption.
Unchanged spontaneous production of IL-6 and significantly higher LPS-stimulated production of this cytokine reflected an increased responsiveness to activation by LPS by peripheral blood mononuclear cells from rats administered warfarin. This reflects their priming state, i.e., a state in which functional response(s) to an activating stimulus is potentiated by a prior exposure to a priming stimulus (Hallet and Lloyds, 1995). The priming of mononuclear cells toward IL-6 production demonstrated pro-inflammatory effects of subchronic oral warfarin intake. Unchanged polymorphonuclear cell IL-6 production (as opposed to priming of mononuclear cells) implies that the effect of warfarin on a given activity in question depends on the type of cell as well. According to the observed warfarin-induced inhibition of TNFα production by granulocytes (as compared to lack of changes in IL-6), it seems that the effect of warfarin on the cells of the same type depends on the cytokine being evaluated.
Differences in the effects on peripheral blood leukocytes (depending on cell type or cytokine produced) might ultimately differentially affect reactions mediated by these cells. According to the presented data, both stimulatory as well as suppressive effects on immune reactivity might be expected. However, although warfarin induced a reduction in TNF production by PMN (which might contribute to an overall immune suppression), a number of PMN activities in rats were up-regulated after warfarin intake, including oxidative activity (respiratory burst, nitric oxide production, and myeloperoxidase content; see Belij et al., Citation2012). Increases in these activities were noted after 30 days of warfarin intake, but not earlier (unpublished data). Therefore, in line with our previous study that showed activation of peripheral blood polymorphonuclear cells in response to acute exposure to warfarin which gained access to the systemic circulation via different routes even after different types of exposures, i.e., peritoneal (Kataranovski et al., Citation2007b) or dermal (Kataranovski et al., Citation2003, Citation2008), the present study and that of Belij et al. (Citation2012) showed that polymorphonuclear cells are a target of warfarin action in subchronic regimens as well.
Apart from a priming of the host mononuclear cells for IL-6 production, a low but increased oxidative activity of MNC (as evaluated by NBT assay of respiratory burst; Choi et al., Citation2006) was noted at both 15 (increased 30% vscontrol values) and 30 days (increased 49% vs control levels) of warfarin intake. These data collectively illustrate the pro-inflammatory effects of warfarin on peripheral blood leukocytes. In light of the cytokine data in this study and other data that clearly indicate pro-inflammatory effects of warfarin on peripheral blood leukocytes, an enhancement of the host immune response might be expected. Accordingly, further examination of a possible impact of warfarin on immunological responses merits future attention.
The immunomodulatory effects of subchronic oral warfarin intake demonstrated in this study could have implications for humans on warfarin therapy. Certain clinical complications connected to warfarin therapy, including thrombosis in patients with over-coagulation (Poli et al., Citation2003) as well as some adverse reactions associated with the presence of inflammatory cells in affected tissues (Kuwahara et al., Citation1995; Hermes et al., Citation1997; Ad-El et al., Citation2000; Kapoor and Bekaii-Saab, Citation2008; Jo et al., Citation2011), might be related to the observed influence of warfarin on peripheral blood leukocyte cytokines. Pro-inflammatory effects (i.e., monononuclear cell priming for IL-6 production) and the impact on TNFα production by granulocytes, the most abundant type of leukocytes in peripheral blood in humans, bear out the need for introspection with regard to the use of warfarin as a therapeutic modality.
Acknowledgments
This study was supported by the Ministry of Education and Science of the Republic of Serbia, Grant #173039. The authors thank dr Djordje Miljković, for expert help in PCR analysis and Jelena Djokić for help in the processing of manuscript.
Declaration of interest
The authors report no conflicts of interest. The authors alone are responsible for the content and writing of the paper.
References
- Ad-El, D. D., Meirovitz, A., Weinberg, A., Kogan, L., Arieli, D., Neuman, A.Linton, D. 2000. Warfarin skin necrosis: Local and systemic factors. Br. J. Plast. Surg. 53:624–626.
- Becker, R. C. 2007. Warfarin-induced vasculopathy. J. Thromb. Thrombolysis 23:79–81.
- Belij, S., Miljković, D., Popov, A., Subota, V., Timotijević, G., Slavić, M., Mirkov I., Kataranovski, D. Kataranovski, M. 2012. Effects of subacute oral warfarin administration on peripheral blood granulocytes in rats. Food Chem. Toxicol. 50:1499–1507.
- Berkarda, B., Bouffard-Eyüboglu, H. Derman, U. 1983. The effect of coumarin derivatives on the immunological system of man. Agents Actions 13:50–52.
- Berkarda, B., Marrack, P., Kappler, J. W. Bakemeier, R. F. 1978. Effects of warfarin administration on the immune response of mice. Arzneimittelforschung 28:1407–1410.
- Bobek, V.Kovarik, J. 2004. Anti-tumor and anti-metastatic effect of warfarin and heparins. Biomed. Pharmacother. 58:213–219.
- Bobek, V., Boubelik, M., Fiserova, A., L’uptovcova, M., Vannucci, L., Kacprzak, G., Kolodzej, J., Majewski, A. M.Hoffman, R. M. 2005. Anti-coagulant drugs increase natural killer cell activity in lung cancer. Lung Cancer 47:215–223.
- Choi, H. S., Kim, J. W., Cha, Y. N. Kim, C. 2006. A quantitative nitroblue tetrazolium assay for determining intracellular superoxide anion production in phagocytic cells. J. Immunoassay Immunochem. 27:31–44.
- Edwards, R. L.Rickles, F. R. 1978. Delayed hypersensitivity in man: Effects of systemic anti-coagulation. Science 200:541–543.
- Eichbaum, F. W., Slemer, O.Zyngier, S. B. 1979. Anti-inflammatory effect of warfarin and Vitamin K1. Naunyn. Schmiedebergs. Arch. Pharmacol. 307:185–190.
- Furie, B. 2000. Oral anti-coagulant therapy. In: Hematology. Basic Principle and Practice (Hoffman, R., Benz, E. J., Shatill, S. J., V, B., Cohen, J. H., Silberstein, L. E.McGlave, P., Eds.). London: Churchill Livingstone/Harcourt Health Science Co, pp. 2040–2046.
- Hallett, M. B.Lloyds, D. 1995. Neutrophil priming: The cellular signals that say ‘amber’ but not ‘green’. Immunol. Today 16:264–268.
- Hermes, B., Haas, N.Henz, B. M. 1997. Immunopathological events of adverse cutaneous reactions to coumarin and heparin. Acta. Derm. Venereol. 77:35–38.
- Hirsh, J., Dalen, J. E., Anderson, D. R., Poller, L., Bussey, H., Ansell, J., Deykin, D. Brandt, J. T. 1998. Oral anti-coagulants. Mechanism of action, clinical effectiveness, and optimal therapeutic range. Chest 114:445S–469S.
- Howe, A. M.Webster, W. S. 2000. Warfarin exposure and calcification of the arterial system in the rat. Int. J. Exp. Pathol. 81:51–56.
- Jo, Y. M., Park, T. H., Jeong, I. H., Kim, H. J., Ahn, J. H., Kim, W. J., Cho, Y. R., Baek, H. K., Kim, M. H. Kim, Y. D. 2011. Warfarin-induced eosinophilic pleural effusion. Korean Circ. J. 41:109–112.
- Kapoor, K. G.Bekaii-Saab, T. 2008. Warfarin-induced allergic interstitial nephritis and leucocytoclastic vasculitis. Intern. Med. J. 38:281–283.
- Kataranovski, D., Savić, I. R., Kataranovski, M. Soldatović, B. 1988. Trombelastography and the six-day-test as reliable methodological aproaches to the discovery of resistance towards anti-coagulant rodenticides in the Norway rat (Rattus norvegicus BERKENHOUT, 1769). Acta Veterin. 38:3–12.
- Kataranovski, M., Kataranovski, D., Zolotarevski, L., Jovic, M. 2007a. Epicutaneous exposure to anti-coagulant rodenticide warfarin modulates local skin activity in rats. Cut. Ocul. Toxicol. 26:1–13.
- Kataranovski, M., Mirkov, I., Vranković, J., Kataranovski, D. Subota, V. 2008. Percutaneous toxicity of anti-coagulant warfarin in rats. Cutan. Ocul. Toxicol. 27:29–40.
- Kataranovski, M., Prokić, V., Kataranovski, D., Zolotarevski, L. Majstorović, I. 2005. Dermatotoxicity of epicutaneously-applied anti-coagulant warfarin. Toxicology 212:206–218.
- Kataranovski, M., Vlaški, M., Kataranovski, D., Tošić, N., Mandić-Radić, S.Todorović, V. 2003. Immunotoxicity of epicutaneously-applied anti-coagulant rodenticide warfarin: Evaluation by contact hypersensitivity to DNCB in rats. Toxicology 188:83–100.
- Kataranovski, M., Živanović, J., Vranković, J., Mirkov, I.Kataranovski, D. 2007b. Granulocyte-stimulating activity of the anti-coagulant warfarin in rats. Arch. Biol. Sci. 59:53–54.
- Kater, A. P., Peppelenbosch, M. P., Brandjes, D. P.Lumbantobing, M. 2002. Dichotomal effect of the coumadin derivative warfarin on inflammatory signal transduction. Clin. Diagn. Lab. Immunol. 9:1396–1397.
- Kuebler, W. M. 2005. Inflammatory pathways and microvascular responses in the lung. Pharmacol. Rep. 57(Suppl):196–205.
- Kurohara, M., Yasuda, H., Moriyama, H., Nakayama, M., Sakata, M., Yamada, K., Kotani, R., Hara, K., Yokono, K.Nagata, M. 2008. Low-dose warfarin functions as an immunomodulator to prevent cyclophosphamide-induced NOD diabetes. Kobe J. Med. Sci. 54:E1–E13.
- Kuwahara, T., Hamada, M., Inoue, Y., Aono, S.Hiwada, K. 1995. Warfarin–induced eosinophilic pleurisy. Intern. Med. 34:794–796.
- Lund, M. 1988. Anti-coagulant rodenticides. In: Rodent Pest Management (Prakash, I. , Ed.). Boca Raton, FL: CRC Press, pp. 341–351.
- Nakano, T., Kawamoto, K., Kishino, J., Nomura, K., Higashino, K. Arita, H. 1997. Requirement of γ-carboxyglutamic acid residues for the biological activity of Gas6: Contribution of endogenous Gas6 to the proliferation of vascular smooth muscle cells. Biochem. J. 323:387–392.
- Nakchbandi, W., Müller, H., Singer, M. V., Löhr, M. Nakchbandi, I. A. 2006. Effects of low-dose warfarin and regional chemotherapy on survival in patients with pancreatic carcinoma. Scand. J. Gastroenterol. 41:1095–1104.
- Oez, S., Platzer, E. Welte, K. 1990. A quantitative colorimetric method to evaluate the functional state of human polymorphonuclear leukocytes. Blut. 60:97–102.
- Perez, R. L., Roman, J., Staton, G. W, Jr., and Hunter, R. L. 1994. Extravascular coagulation and fibrinolysis in murine lung inflammation induced by the mycobacterial cord factor trehalose-6,6′-dimycolate. Am. J. Respir. Crit. Care Med. 149:510–518.
- Poli, D., Antonucci, E., Gensini, G. F., Abbate, R.Prisco, D. 2003. Asymptomatic excessive coumarin anti-coagulation is a risk factor for thrombotic and bleeding complications of oral anti-coagulant therapy. J. Thromb. Haemost. 1:1840–1841.
- Popov, A., Mirkov, I., Zolotarevski, L., Jović, M., Belij, S., Kataranovski, D.Kataranovski, M. 2011. Local pro-inflammatory effects of repeated skin exposure to warfarin, an anti-coagulant rodenticide in rats. Biomed. Environ. Sci. 24:180–189.
- Price, P. A. 1988. Role of Vitamin-K-dependent proteins in bone metabolism. Annu. Rev. Nutr. 8:565–583.
- Reagan-Shaw, S., Nihal, M.Ahmad, N. 2007. Dose translation from animal to human studies revisited. FASEB J. 22:659–661
- Shearer, M. J. 1990. Vitamin K and Vitamin K-dependent proteins. Br. J. Hematol. 75:156–162.
- World Health Organization (WHO). 1995. Anti-coagulant Rodenticides. (Environmental Health Criteria, No. 175) Geneva: WHO, pp. 1–121.
- Yanagita, M., Ishii, K., Ozaki, H., Arai, H., Nakano, T., Ohashi, K., Mizuno, K., Kita, T.Doi, T. 1999. Mechanism of inhibitory effect of warfarin on mesangial cell proliferation. J. Am. Soc. Nephrol. 10:2503–2509.