Abstract
There is currently no validated animal model for evaluating the potential allergenicity of food proteins. This study aimed to compare the allergic reactions between BN and Wistar rats after oral exposure to ovalbumin (OVA) by studying immune responses and clinical manifestations. Female BN and Wistar rats were orally exposed to OVA on days 1 and 14, and thereafter daily from day 15 to day 42. Sera and plasma were screened for OVA-specific antibodies and histamine. On day 49, all the OVA-sensitized animals were orally challenged with OVA before blood pressure was measured. One day later (on day 50), histopathology and differential cell counts were performed. The results indicate that oral exposure of BN rats to OVA yielded IgE, IgG, and IgG2a antibody responses that were generally of higher levels than those observed in Wistar rats (p < 0.05). However, the Wistar rats presented with more serious clinical manifestations and histopathologic changes that could have serious implications for any OVA-induced anaphylaxis. The studies here proved that OVA-sensitized BN and Wistar rats evinced different immune responses and clinical manifestations; these outcomes suggested that the two rat strains might differ in their immunologic mechanisms of allergy and that there was no correlation between immune responses and the severity of clinical symptoms. To be clear, the data from these studies should be viewed as ‘preliminary’, as only a single protein allergen was examined. Accordingly, further studies are needed to compare the allergic reactions between BN and Wistar rats by using purified strong-, weak-, and non-allergenic proteins based on the experiments reported here.
Introduction
Food allergy, an adverse immune response to foods, appears to have an escalating morbidity in recent years. The prevalence of food allergy has been estimated at ≈ 3~4% of adults in Westernized countries and at ≈ 5% in young children (Sicherer and Sampson, Citation2010). Food-induced allergic reactions are responsible for a variety of symptoms and disorders involving the skin, as well as the gastrointestinal and respiratory tracts, and can be attributed to IgE-mediated and non-IgE-mediated (cellular) mechanisms (Sicherer and Sampson, Citation2010). At present, the most important food allergens are peanuts, tree nuts, soy, milk, fish, shellfish, wheat, and egg. However, with the rapid introduction of novel foods, including foods and food products derived from genetically-modified crops, there has been a growing interest in approaches available to assess potential allergenicity of novel gene products (Taylor and Hefle, Citation2001; Lack et al., Citation2002; Hollingworth et al., Citation2003).
Investigators have explored the use of animal models, including rats, mice, and guinea pigs, for assessments of allergenic potential (Knippels et al., Citation1999a; Piacentini et al., Citation2003; Vinje et al., Citation2009). Some disadvantages limit the use of guinea pigs as a suitable model, including significant differences in immunophysiology compared to other species and a lack of tools to study its immune system (Ahuja et al., Citation2010). For mice, the natural complexity of the allergic reactions makes it difficult to find a single reliable marker to quantify the sensitization potential of a protein (Aldemir et al., Citation2009). However, rats have a number of advantages compared with other animal models, particularly with respect to being one of the most commonly-used species in toxicity testing. These include a reasonable amount of knowledge about their immune system and the availability of many tools for immune-related studies (Penninks and Knippels, Citation2001).
It is imperative to note that, currently, none of the more appropriate routes of exposure has been validated in the assessment of the allergenicity of novel proteins. In theory, a parenteral administration of protein avoids the development of oral tolerance and provides a clear indication of the inherent ability of proteins to induce IgE antibody responses. Still, it must be acknowledged that the natural route of feeding during the sensitization period was not taken into account (Dearman and Kimber, Citation2001). Clearly, food allergens can induce anaphylaxis via intra-gastric, sublingual, nasal, or cutaneous routes; nevertheless, oral exposure to food allergens occurs quite often, starting at the earliest stages of a life (Dunkin et al., Citation2011). Therefore, in the present study, we investigated the extent of allergic reactions compared between BN and Wistar rats following oral exposure to ovalbumin.
Materials and methods
Animals and allergens
Female out-bred Wistar and inbred BN rats (each 4-weeks-old) were purchased from Vital River Company (Beijing, China) and maintained on an egg-free diet prior to purchase. All rats were acclimatized for at least 5 days prior to study initiation. Animals were kept under specific pathogen-free conditions maintained at 23 (± 3)°C and a relative humidity of 40–70% with a light/dark cycle of 12 h. All rats were housed in stainless-steel wire cages in groups of four and given ad libitum access to a commercially available egg-free rodent diet and distilled water. The Beijing Municipal Science and Technology Commission of China (NO. SYXK 2010-0036) approved all of the animal studies described herein.
Sensitization and challenge
Rats were exposed by gavage to ovalbumin (OVA, Lot No. A5503, Sigma, St. Louis, MO) on days 1 and 14, and thereafter daily from day 15 to day 42; the dose used was 150 µg OVA/g body weight (BW; 1 ml/rat). Controls received 1 ml saline each time. After this induction period, the rats were not exposed to OVA for 1 week. On day 49, all OVA-sensitized animals were orally challenged with OVA solution in distilled water (750 µg OVA/g[BW], vol = 2 ml/rat); control rats received 2 ml saline.
Blood samples were obtained from the orbital plexus on days 0, 14, 28, 35, and 42. Each sample was allowed to clot for 1 h at room temperature and then centrifuged at 3000 × g (10 min, 4°C) to obtain sera for use in antibody analyses. The sera in day 0 was pooled and used as a negative control. All individual and pooled sera were stored at −20°C until analysis. Samples for plasma histamine level determination and differential cell counts were collected separately (on the same days) into chilled EDTA-K2 tubes.
Antibody (IgE, IgG, and IgG2a) titer determination
Determinations of serum OVA-specific IgG and IgG2a levels were performed using an indirect ELISA with OVA-coated microtiter plates as described in Knippels et al. (Citation1998). For the detection of serum OVA-specific IgE, a capture ELISA was employed. Briefly, 96-wells microtiter plates were coated overnight at 4°C with mouse anti-rat IgE (Lot #553914, BD Biosci- ences, San Jose, CA) solution (100 μl/well, at 1.5 μg/ml in carbonate buffer [pH 9.6]). The coating solution was removed by washing with phosphate-buffered saline (pH 7.4) containing 0.1% Tween-20 (PBST) and non-specific binding blocked by addition of PBST containing 2% non-fat dry milk (150 μl/well) and incubation for 2 h at room temperature. Wells were then washed 3-times with PBST before the addition of the serum samples. After incubation for 1 h at 37°C, the plates were washed and then biotinylated OVA (10 mg/ml in 2% BSA/PBST; 100 μl/well) was added and the plates incubated for 1 h at 37°C. The plates were then washed before horseradish peroxidase-conjugated streptavidin (1:4000 dilution in 1% non-fat dry milk; Lot #LF148729, Thermo Scientific, Rockford, IL; 100 µl/well) was added and the plates incubated for 15 min at 37°C. The plates were then washed 3-times with PBST before 100 μl tetramethylbenzidine (TMB, Lot No. 10085, KPL, Gaithersburg, MD) substrate was added to each well. The plates were incubated ≈ 15 min at room temperature before each well received 100 μl of 2 N sulfuric acid (Stop Solution). Thereafter, the optical density in each well was measured at 450 nm using a Multiskan MK3 automated microplate reader (Thermo Scientific).
All pooled Day 0 sera used as a negative control and all of these samples were measured at a 1:4 dilution. The average absorbance in negative control wells (to which 3-times the standard deviation was added) provided the reference value for determining the titer (presented in terms as log2 titer) in each test sera sample. For each test serum, the reciprocal of the furthest serum dilution yielding an absorbance higher than the reference value was accepted as the antibody titer.
Determination of plasma histamine level
Blood was collected 30 min after the oral exposure to OVA or saline on days 14, 28, and 42. Blood samples of rat collected into chilled tubes were centrifuged at 3000 × g (10 min, 4°C) to obtain plasma aliquots and stored at −80°C until analysis. Plasma histamine levels were then determined using a Rat Histamine (HIS) ELISA Kit (Lot No. H026-10, Groundwork Biotechnology Diagnostics Ltd., San Diego, CA) following manufacturer instructions. In this case, the optical density in each well was measured at 450 nm in the automated microplate reader. The level of sensitivity of the kit was 0.5 ng/ml.
Differential cell counts
Blood samples obtained on day 50 were collected into chilled tubes containing EDTA and analyzed within 4 h. The number of basophils, eosinophils, and neutrophils in the blood were determined using an Animal Hematology System (Type Hemavet 950, Drew Scientific Inc., Dallas, TX).
Determination of blood pressure
On day 49, all OVA-sensitized rats were orally challenged with 2 ml of OVA solution in distilled water (750 µg/g·BW); control rats received 2 ml saline. To avoid stress during experiments, the rats were adapted to the blood pressure cuff during the sensitization period. Prior to the high-dose stimulation, baseline blood pressures of the rats were measured. During the measurement period, the rats were confined in a small tube wrapped with thermal insulation pipe that was maintained at 20°C. An inflatable pressure cuff was put around the tail and a distal taped sensor was used to record the systolic blood pressure. Blood pressure was determined by a non-invasive blood pressure cuff (Type BP-98A, Softron, Tokyo, Japan) at intervals during a period of 7 h.
Histopathologic analysis
Animals were euthanized by cervical dislocation 24 h after the oral challenge (i.e., on day 50). At necropsy, the heart, liver, spleen, lung, and kidney of each rat were preserved in neutral phosphate-buffered 10% (v/v) formaldehyde and embedded in paraffin wax. In the case of the lungs, this occurred after inflation with fixative. Sections (6-µm thick) were then prepared using standard protocols and then the tissues were stained with hematoxylin and eosin (Sigma).
Statistical analysis
Between-group antibody titers, plasma histamine levels, and immune cell subtype numbers were statistically compared using a two-tailed Student’s t-test contained in the SPSS 13.0 program (SPSS Inc., Chicago, IL). Differences were considered statistically significant at p-values ≤ 0.05.
To evaluate blood pressure changes in the OVA-challenged animals, the upper and lower limits of the 95% confidence range of blood pressure values seen with the control rats were used as reference. A drop in blood pressure was considered significant when the pressure value fell outside the lower limit of the 95% confidence range (Knippels et al., Citation1999b).
Results
OVA-specific antibody (IgE, IgG, and IgG2a) titers
Prior to initiation of exposure, blood samples were collected and serum samples were prepared. Sera were pooled and used as negative control in the ELISA tests. Upon oral sensitization with OVA, OVA-specific IgE responses were detectable in the BN and Wistar rats on days 0, 14, 28, and 42. For BN rats, OVA-specific IgE titers increased significantly, with maximal OVA-specific IgE levels demonstrated on day 28 after initiation of oral exposures (: log2 titer = 1.48 ± 0.42 [mean ± SD]), and then slightly decreased. For Wistar rats, the OVA-specific IgE responses were detectable from day 14 onwards, with maximum responses detectable on day 42 (: log2 titer = 1.62 ± 0.28).
Figure 1. Effects on OVA-specific antibody levels. Levels of (a) IgE, (b) IgG, and (c) IgG2a response in young female BN and Wistar rats in the period following oral exposure to OVA. OVA was delivered to BN rats (♦) and Wistar (▪) rats on days 1 and 14, and thereafter daily from day 15 to day 42. At the same time, control BN rats (▴) and Wistar rats (•) received the same volume of saline. Data are presented as mean log2 titer (± SD) of six rats per group. a For BN or b Wistar rats, OVA-exposed group significantly differs from the control at p < 0.05.
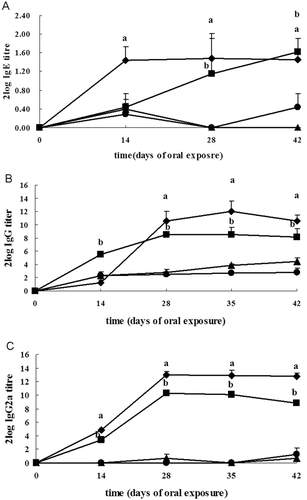
OVA-specific IgG responses were detectable in BN and Wistar rats from day 14 to day 42. Maximal specific IgG antibody responses tended to be provoked at day 35 in BN rats (: log2 titer = 12.00 ± 1.55) and Wistar rats (: log2 titer = 8.54 ± 1.05), with reduced titers observed at day 42 in the BN rats (: log2 titer = 10.58 ± 0.89) and Wistar rats (: log2 titer = 8.15 ± 1.30). A similar kinetic profile was observed for the elicitation of anti-OVA IgG2a antibody production, i.e., OVA-specific IgG2a responses initially increased and then decreased. However, maximal IgG2a responses were induced at day 28 in the BN (: log2 titer = 13.04 ± 0.41) and Wistar (: log2 titer = 10.26 ± 0.43) rats. In addition, oral exposure of BN rats to OVA stimulated IgG and IgG2a antibody responses that were generally at a higher titer than those observed in Wistar rats, with the exception of IgG titers at day 14.
Plasma histamine levels
To determine the clinical consequences of the OVA-specific IgE antibody production, the extent of mast cell degranulation was assessed using measures of histamine. Maximal histamine levels tended to be provoked on day 28 following initiation of exposure, with reduced levels observed on day 42 in both rat strains (). Moreover, both BN and Wistar rats showed significantly increased plasma levels of histamine on day 28.
Figure 2. Effects on histamine release. Release in young female (a) BN and (b) Wistar rats after oral exposure to OVA or saline on days 1 and 14, and thereafter daily from day 15 to day 42. White bars represent controls and black bars the OVA-exposed groups. Levels of histamine in plasma were measured using ELISA. Mean values (ng/ml) with 95% CI (confidence intervals) for the different groups are shown. * Significantly differs from control at p < 0.05.
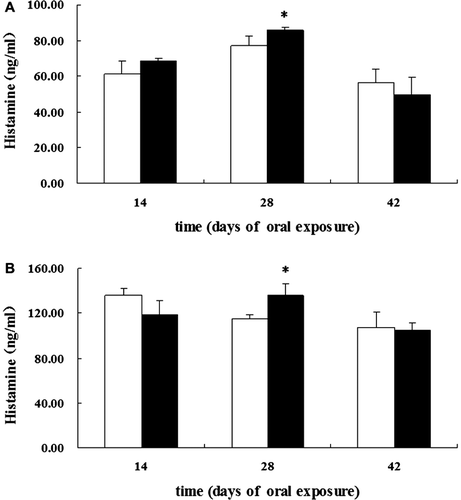
Blood basophil, eosinophil, and neutrophil levels
Another facet of an allergic immune response is an increase of basophils, eosinophils, and neutrophils in the blood. On day 50 following initiation of OVA exposure, immune cell numbers were analyzed in blood samples collected from the rats. OVA exposures resulted in significant increases in the numbers of basophils in both strains of rats (). On the other hand, while sensitized BN rats demonstrated significant increases in the number of eosinophils () and neutrophils () as compared to their strain-matched control group, there were no similar significant increases noted in the sensitized Wistar rats.
Figure 3. Effects on granulocyte levels. Numbers of (a) basophils, (b) eosinophils, and (c) neutrophils in the blood of young female BN and Wistar rats in the period after oral exposure to OVA or saline (on day 49). White bars represent controls and black bars OVA-exposed groups. Data are presented as mean (± SD) of six rats per group. * Significantly differs from control at p < 0.05.
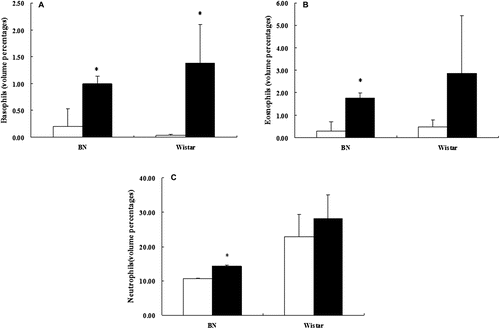
Effects on blood pressure
To further illustrate the clinical consequences associated with any changes in antibody formation, histamine release, and/or immune cell populations, effects on systolic blood pressure were measured on day 49 after initiation of the OVA exposures. Systolic blood pressure was determined in control and oral-sensitized rats upon oral challenge with either saline or OVA. The 95% confidence range of blood pressure values of control animals before challenge was used as a reference range for the evaluation of pressure changes in the challenged rats. No changes in systolic blood pressure were seen in the control rats upon oral challenge with saline. The individual blood pressure pattern of a representative OVA-challenged animal is shown in . A temporary drop in systolic blood pressure in several of the BN rats was found 2 h after an oral challenge with OVA, and returned to normal within 1 h. In the majority of the Wistar rats, an almost continuous decrease in blood pressure was observed 3 h after challenge. Of note, the level of blood pressure dropped beneath the lower 95% confidence limit in two of six BN rats and four of six Wistar rats.
Figure 4. Effects on blood pressure. Levels of change in pressure values on day 49 in young female (a) BN and (b) Wistar rats after oral challenge with OVA (▪) or in control rats after oral challenge with saline (♦). Data shown are means (± SD) of repeated blood pressure measures (in mm Hg) for individual rats for a period of 7 h. The broken lines indicate the upper and lower limits of the 95% confidence range of blood pressure values for the control rats. A drop in blood pressure was considered significant when the value dropped below the 95% confidence range.
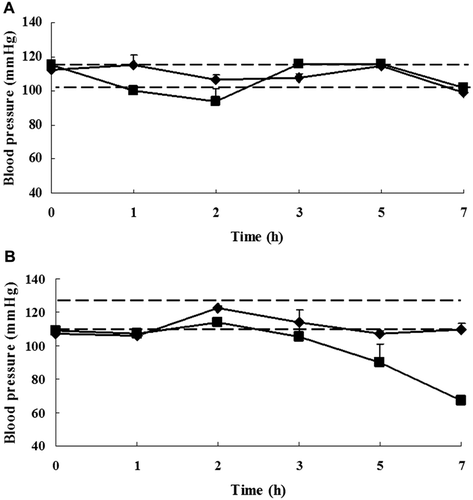
Histopathologic changes in OVA-sensitized rats
As shown in Supplemental Figures S1–S5, saline control rats demonstrated normal morphology in the various organs (e.g., heart, lung, liver, spleen, and kidney) obtained 24 h after the oral challenge (i.e. on day 50). In contrast, diffuse steatosis in cardiac tissues and eosinophil infiltration in lung tissues were mainly observed in OVA-sensitized BN rats. OVA-sensitized Wistar rats also had steatosis in their cardiac and liver tissues, but also displayed macrophage aggregates in their spleens, a slight interstitial pneumonia in the lungs, and evidence of tubulo-interstitial nephritis. summarizes the number of rats that had the various lesions.
Table 1. Histopathologic changes in BN and Wistar rats sensitized to OVA.
Discussion
The IgE-dependent allergic reaction is composed of two phases: an inducing step, in which the immune system of the host is sensitized by the allergen, ending up in specific IgE anti- bodies production which bind to specific cell surface receptors on mast cells in target organs; the second step is a triggering phase mediated by the allergen binding to these IgE and stimulating mediator (histamine) release from mast cells. For evaluating the allergenicity of food antigens, both phases should be examined by appropriate tests (Fritsche, Citation2009).
OVA-specific antibody responses were the markers of the inducing phase. OVA-sensitized BN and Wistar rats both showed significantly higher OVA-specific antibodies titers from day 28 to day 42 compared with the negative control group. However, OVA-specific IgE titers in BN rats were significantly increased, with a maximal level seen on day 28 following initiation of oral exposure. In contrast, in the Wistar rats, the OVA-specific IgE responses were only detectable after day 14. These observations indicate that the BN rats were more sensitive to OVA compared to the Wistar rats with respect to the IgE-dependent allergic reaction. The BN rats also showed a decrease in OVA-specific IgE antibody levels with prolonged oral exposure to the antigen. This is probably attributable, at least in part, to the fact that constant oral exposure may be associated with development of oral tolerance, a process driven by T-regulatory cells and other associated mechanisms (Ahuja et al., Citation2010). On the other hand, in Wistar rats, an almost continuous increase in OVA-specific IgE titers was observed.
It has been demonstrated that induction of a specific IgE antibody is invariably accompanied by induction of an antigen-specific IgG (Vaz et al., Citation1970, 1971; Yamanishi et al., Citation2003). This is especially the case for sub-classes of IgG that are regulated in a manner similar to IgE (as surrogates for IgE). In the rat, IgG2a antibody is subject to similar cytokine regulation as IgE (Saoudi et al., Citation1993; Gracie and Bradley, Citation1996; Kimber et al., Citation2003). Oral exposure of BN rats to OVA led to stimulated IgG and IgG2a antibody responses that were generally expressed as higher titers relative to those in Wistar rat counterparts, with the exception of IgG on day 14.
Plasma histamine level was utilized as a marker of the triggering phase. Both BN and Wistar rats showed significantly increased plasma levels of histamine on day 28. The second parameter of the triggering phase that we studied was an increase of the number of basophils, eosinophils, and neutrophils in the blood. Basophils are recognized as key cells in allergic inflammatory reactions owing to their ability to release the histamine mediator. In contrast, eosinophils may inhibit allergic reactions since they release de-activating histaminase (Renninger et al., Citation2010). While sensitized BN and Wistar rats demonstrated significant increases in their numbers of basophils, eosinophil levels in the BN rats also were significantly increased in their blood and lungs. Thus, it is possible that while both strains of rats had elevations in basophil numbers and there might have been equally elevated levels of histamine release by those cells, the added presence of histaminase-releasing eosinophils may have led to inactivation/degradation of some of the histamine whose release had been triggered by the OVA in the BN rats. If this is the case, then it is plausible to assume that the BN rats may have had the greater ‘release’ of histamine than their Wistar counterparts, but that the ultimate impact of this was mitigated in situ by the added presence of more and more eosinophils.
To further illustrate the clinical consequences associated with any changes in antibody formation, histamine release, and/or immune cell populations, effects on systolic blood pressure were measured on day 49 after initiation of the OVA exposures. These measures would, in turn, aid in our ability to better define mechanisms associated with the pathology of IgE-mediated allergic reactions (Ahuja et al., Citation2010). Some BN rats demonstrated a temporary drop in systolic blood pressure that returned to normal values within 1 h, which is in accordance with previous studies (Knippels et al., Citation1999b; Knippels and Pennicks Citation2002; Jia et al., Citation2005). However, some Wistar rats showed an almost continuous decrease in blood pressure after challenge. Because these clinical manifestations were found to be only evident in some animals, these outcomes could be taken to reflect the situation also seen in food-allergic patients (Knippels et al., Citation1999b).
It has been recognized that eosinophilia is the first inflammatory cell to be linked firmly to asthma pathogenesis (Marsh et al., Citation1985; Dunn et al., Citation1988), followed soon after by the T-cell (Mosmann et al., Citation1986). Histopathologic examination of lungs of OVA-challenged BN rats revealed features associated with increased IgE levels and allergic asthma, namely eosinophilic aggregates. Similar features were seen in the blood analysis results, i.e., sensitized BN rats had significant increases in the number of eosinophils as compared to in same-strain controls. In a strain-specific outcome, an OVA-challenged Wistar rat also manifested slight interstitial pneumonia. These results also corresponded to previous studies (see Dunn et al., Citation1988; Bentley et al., Citation1993; Virchow et al., Citation1994). Moreover, increased eosinophils were observed in patients who will develop late asthmatic reactions 24 h following allergen provocation (Durham and Kay, Citation1985). Since it is recognized that T-helper (TH)-2 cytokines, particularly IL-5 and to a certain extent IL-4, are also key factors in allergic asthma due to their ability to promote eosinophil proliferation in the lungs (Jason et al., Citation2009), measures of systemic and local IL-5 levels might also be useful in our future studies of the inflammation that occurs as a part of the pathology associated with allergic asthma.
Conclusions
OVA-sensitized BN and Wistar rats developed different immune responses and clinical manifestations. Such outcomes suggested to us that the two rat strains may differ in their immunologic mechanisms of allergy, and, further, that there was no correlation between immune responses and the severity of clinical symptoms. To be clear, the data from these studies should be viewed as ‘preliminary’, as only a single protein allergen was examined. Accordingly, further studies are needed to compare the allergic reactions between BN and Wistar rats by using purified strong-, weak-, and non-allergenic proteins based on the experiment we report out here. In addition, as there exists a certain correlation between the presence of select inflammatory mediators and clinical symptoms, assessment of levels of these mediators might be of diagnostic value and possibly useful in monitoring the severity of clinical symptoms in the future.
Note to readers
It became apparent during the journal review process that there were concerns that only a single antigen (i.e., OVA) was utilized in the experiments here and that this might not be sufficient to allow for one to draw conclusions about strain-related differences in immunologic outcomes. Before conducting the experiment, a large number of papers were reviewed that also investigated potential allergenicities of food proteins in an animal model (i.e., Knippels et al., Citation1998, 1999, a, b, c; Akiyama et al., Citation2001; Hylkema et al., Citation2002; Knippels and Penninks, Citation2002; Pilegaard and Madsen, Citation2004). Each of those studies only used well-defined chicken egg white allergen ovalbumin as the model antigen. Accordingly, the current studies compared allergic reactions between BN and Wistar rats after oral exposure to that defined allergen (so that our data could ultimately be compared to those of the previous studies). It is clear now that, in subsequent studies, purified strong-, weak-, and non-allergenic proteins will also need to be used to compare allergic reactions between the BN and Wistar rats. We also want to note that earlier studies that described development of an enteral model for food protein allergenicity only used BN rats (Knippels et al., Citation1998, 1999a, b; Akiyama et al., Citation2001; Hylkema et al., Citation2002; Knippels and Penninks, Citation2002; Pilegaard and Madsen, Citation2004). Lastly, comparative sensitization studies that used Wistar, Piebald Virol Glaxo (PVG), Hooded Lister, and BN rats for comparing responses to OVA only investigated strain-related differences in immune response parameters and not systemic outcomes as well. Thus, while we believe that the studies here are in line with others (for reasons outlined above), they are also unique in that they also provide information about potential strain-specific systemic toxicities (that could be associated with modified immunologic outcomes) in hosts exposed to a strong allergen (OVA) by the enteral route.
Supplementary Material
Download PDF (10.5 MB)Acknowledgments
The National Natural Science Foundation of China (NO. 81072305) provided financial support for this study. The authors wish to thank the Supervision, Inspection, and Testing Center of Genetically Modified Organisms of the Ministry of Agriculture, as well as the Institute of Medicinal Plant Development of China, for their technical assistance.
Declaration of interest
The authors report no conflicts of interest. The authors alone are responsible for the content and writing of the paper.
References
- Ahuja, V., Quatchadze, M., Ahuja, V., Stelter, D. 2010. Evaluation of biotechnology-derived novel proteins for the risk of food-allergic potential: Advances in the development of animal models and future challenges. Arch. Toxicol. 84:909–917.
- Akiyama, H., Teshima, R., Sakushima, J. I., Okunuki, H., Goda, Y., Sawada, J., Toyoda, M. 2001. Examination of oral sensitization with ovalbumin in Brown Norway rats and three strains of mice. Immunol. Lett. 78:1–5.
- Aldemir, H., Bars, R., Herouet-Guicheney, C. 2009. Murine models for evaluating the allergenicity of novel proteins and foods. Regul. Toxicol. Pharm. 54:S52–S57.
- Bentley, A. M., Meng, Q., Robinson, D. S., Hamid, Q., Kay, A. B., Durham, S. R. 1993. Increases in activated T-lymphocytes, eosinophils, and cytokine messenger RNA expression for IL-5 and granulocyte/macrophage colony-stimulating factor in bronchial biopsies after allergen inhalation challenge in atopic asthmatics. Am. J. Respir. Cell. Mol. Biol. 8:35–42.
- Dearman, R. J., Kimber, I. 2001. Determination of protein allergenicity: Studies in mice. Toxicol. Lett. 120:181–186.
- Dunkin, D., Berin, M. C., Mayer, L. 2011. Allergic sensitization can be induced via multiple physiologic routes in an adjuvant-dependent manner. J. Allergy. Clin. Immunol. 128:1251–1258.
- Dunn, C. J., Elliott, G. A., Oostveen, J. A., Richards, I. M. 1988. Development of a prolonged eosinophil-rich inflammatory leukocyte infiltration in the guinea pig asthmatic response to ovalbumin inhalation. Am. Rev. Respir. Dis. 137:541–547.
- Durham, S. R., Kay, A. B. 1985. Eosinophils, bronchial hyper-reactivity and late-phase asthmatic reactions. Clin. Allergy 15:411–418.
- Fritsche, R. 2009. Utility of animal models for evaluating hypo-allergenicity. Mol. Nutr. Food Res. 53:979–983.
- Gracie, J. A., Bradley, J. A. 1996. IL-12 induces IFNγ-dependent switching of IgG allo- antibody subclass. Eur. J. Immunol. 26:1217–1221.
- Hollingworth, R. M., Bjeldanes, L. F., Bolger, M., Kimber, I., Meade, B. J., Taylor, S. L., Wallace, K. B. 2003. The safety of genetically-modified foods produced through biotechnology. Toxicol. Sci. 71:2–8.
- Hylkema, M. N., Hoekstram, O., Luinge, M., Timens, W. 2002. The strength of the OVA-induced airway inflammation in rats is strain dependent. Clin. Exp. Immunol. 129:390–396.
- Jason, H. T., Mercedes, R., Charles, G. I. 2009. Animal models of asthma. Am. J. Physiol. 297:L401–L410.
- Jia, X. D., Li, N., Wu, Y. N., Yang, X. G. 2005. Studies on BN rats model to determine the potential allergenicity of proteins from genetically modified foods. World J. Gastroenterol. 11:5381–5384.
- Kimber, I., Dearman, R. J., Pennincks, A. H., Knippels, L. M., Buchanan, B. B., Hammerberg, B., Jackson, H. A., Helm, R. M. 2003. Assessment of allergenicity on the basis of immune reactivity: Animal models. Environ. Health Perspect. 111:1125–1130.
- Knippels, L. M., Penninks, A. H. 2002. Assessment of protein allergenicity studies in Brown Norway rats. Ann. N.Y. Acad. Sci. 964:151–161.
- Knippels, L. M., Houben, G. F., Spanhaak, S., Penninks, A. H. 1999a. An oral sensitization model in Brown Norway rats to screen for potential allergenicity of food proteins. Methods 19:78–82.
- Knippels, L. M., Penninks, A. H., Spanhaak, S. 1998. Oral sensitization to food proteins: A Brown Norway rat model. Clin. Exp. Allergy 28:368–375.
- Knippels, L. M., Penninks, A. H., Smit, J. J., Houben, G. F. 1999b. Immune-mediated effects upon oral challenge of ovalbumin-sensitized Brown Norway rats: Further characterization of a rat food allergy model. Toxicol. Appl. Pharm. 156:161–169.
- Knippels, L. M., Penninks, A. H., van Meeteren, M., Houben, G. F. 1999c. Humoral and cellular immune responses in different rat strains on oral exposure to ovalbumin. Food. Chem. Toxicol. 37:881–888.
- Lack, G., Chapman, M., Kalsheker, N., King, V., Robinson, C., Venables, K. 2002. Report on potential allergenicity of genetically-modified organisms and their products. Clin. Exp. Allergy 32:1131–1143.
- Marsh, W. R., Irvin, C. G., Murphy, K. R., Behrens, B. L., Larsen, G. L. 1985. Increases in airway reactivity to histamine and inflammatory cells in bronchoalveolar lavage after the late asthmatic response in an animal model. Am. Rev. Respir. Dis. 131:875–879.
- Mosmann, T. R., Cherwinski, H., Bond, M. W., Giedlin, M. A., Coffman, R. L. 1986. Two types of murine helper T-cell clone. I. Definition according to profiles of lymphokine activities and secreted proteins. J. Immunol. 136:2348–2357.
- Penninks, A. H., Knippels, L. M. 2001. Determination of protein allergenicity: Studies in rats. Toxicol. Lett. 120:171–180.
- Piacentini, G. L., Vicentini, L., Bodini, A., Mazzi, P., Peroni, D. G., Maffeis, C., Boner, A. L. 2003. Allergenicity of a hydrolyzed rice infant formula in a guinea pig model. Ann. Allergy Asthma Immunol. 91:61–64.
- Pilegaard, K., Madsen, C. 2004. An oral Brown Norway rat model for food allergy: Comparison of age, sex, dosing volume, and allergen preparation. Toxicology 196:247–257.
- Renninger, M. L., Seymour, R. E., Whiteley, L. O., Sundberg, J. P., Hogen Esch, H. 2010. Anti-IL-5 decreases the number of eosinophils but not the severity of dermatitis in Sharpin- deficient mice. Exp. Dermatol. 19:252–258.
- Saoudi, A., Kulun, J., Huygen, K., De Kozak, Y., Velu, T., Goldman, M., Druet, P., Bellon, B. 1993. TH2-activated cells prevent experimental autoimmune uveoretinitis, a TH1- dependent autoimmune disease. Eur. J. Immunol. 23:3096–3103.
- Sicherer, S. H., Sampson, H. A. 2010. Food allergy. J. Allergy Clin. Immunol. 125:116–125.
- Taylor, S. L., Hefle, S. L. 2001. Will genetically modified foods be allergenic? J. Allergy Clin. Immunol. 107:765–771.
- Vaz, N. M., Phillips-Quagliata, J. M., Levine, B. B., Vaz, E. M. 1971. H-2 linked genetic control of immune responsiveness to ovalbumin and ovamucoid. J. Exp. Med. 134:1335–1348.
- Vaz, N. M., Vaz, E. M., Levine, B. B. 1970. Relationship between H-2 genotype and immune responsiveness to low doses of ovalbumin in the mouse. J. Immunol. 104:1572–1574.
- Vinje, N. E., Larsen, S., Løvik, M. 2009. A mouse model of lupin allergy. Clin. Exp. Allergy 39:1255–1266.
- Virchow, J. C., Kroegel, C., Walker, C., Matthys, H. 1994. Cellular and immunological markers of allergic and intrinsic bronchial asthma. Lung 172:313–334.
- Yamanishi, R., Yusa, I., Bando, N., Terao, J. 2003. Adjuvant activity of alum in inducing antigen- specific IgE antibodies in Balb/c mice: A re-evaluation. Biosci. Biotechnol. Biochem. 67:166–169.