Abstract
Limited non-clinical immunotoxicity data are available in the dog, although this is a major non-rodent species in regulatory safety studies. The present study aimed to test whether widely accepted immunotoxicity endpoints including lymphocyte subset immunophenotyping, the anti-KLH TDAR assay, and histological examination of the main lymphoid organs were reliable to detect immunosuppression induced by cyclosporine and cyclophosphamide in dogs and could, therefore, be used for non-clinical immunotoxicity evaluation in this species. Male and female Beagle dogs were treated orally from Day 1 for 4 weeks with 25 mg/kg cyclosporine daily, or with 2 mg/kg cyclophosphamide on 4 consecutive days each week, or the same volume of drinking water daily. Blood samples were withdrawn pre-test and on Days 11, 18, and 23 to measure standard hematology parameters and analyze lymphocyte subsets. All animals received an intramuscular injection of 5 mg KLH on Day 11. Sandwich ELISA assays were used to quantify anti-KLH IgM and anti-KLH IgG levels in blood samples taken pre-test, on Days 18 and 23, and pre-test, on Days 23 and 28, respectively. At the end of the treatment period, all animals were submitted to histological examination of lymphoid organs, liver, and kidneys. No signs of marked toxicity were observed. No changes in lymphocyte subsets, but markedly decreased primary anti-KLH IgM and IgG responses, and a slightly-to-markedly increased cortex/medulla ratio in the thymus were observed in cyclosporine-treated dogs. Lower total WBC counts correlating with lower total and B-lymphocyte subset and decreased germinal center development in mesenteric lymph nodes, but no changes in primary anti-KLH IgM and IgG responses were observed in cyclophosphamide-treated dogs. These results demonstrate that widely accepted immunotoxicity endpoints can adequately detect the effects of known immunosuppressive drugs in the dog and support the conclusion that it is a relevant animal species for immunotoxicity evaluation.
Introduction
The dog is currently the main non-rodent species utilized in non-clinical toxicity studies of drug candidates. Nevertheless, limited immunotoxicity data in the dog have so far been published. The implementation of ICH guideline S8 on immunotoxicity studies for human pharmaceuticals (ICH S8, 2005) is expected to increase the need for non-rodent immunotoxicity studies. Indeed, immunotoxicology investigations may be needed in the dog if the ‘classical’ 28-day rodent immunotoxicity study does not provide sufficient information for a risk benefit decision or if standard toxicity studies or metabolic data demonstrate that the dog is a more relevant animal species than the rat.
A recent survey of the ILSI HESI Immunotoxicology Technical Committee published in this journal gave a snapshot of immunologic methods and immunotoxicity assays in dogs that are currently in use, mostly in the pharmaceutical industry (Lebrec et al., Citation2012). Although a fairly wide variety of techniques and reagents are successfully used in dog studies, many have not yet been standardized or formally validated, as previously highlighted (Haggerty, Citation2007). The aim of the present study was to test whether widely accepted immunotoxicity endpoints, including lymphocyte subset immunophenotyping, the anti-KLH TDAR assay, and histological examination of the main lymphoid organs, are reliable to detect the well-known immuno-suppressive effects of cyclosporine and cyclophosphamide in the dog and could, therefore, be used for non-clinical immunotoxicity evaluation in this species.
Beagle dogs have been treated with the immunosuppressive drugs cyclosporine and cyclophosphamide for 4 weeks. WBC, total lymphocyte, and B lymphocyte counts were affected by cyclophosphamide but not cyclosporine treatment, while the T-dependent primary antibody response to Keyhole Limpet Hemocyanin (KLH) was reduced by cyclosporine, but not cyclophosphamide treatment. Histology of lymphoid organs was affected by both treatments. The results of this study suggest that the dog can be considered a relevant species for non-clinical immunotoxicity evaluation.
Materials and methods
Animals
Male and female Beagle dogs (aged 12–13 months at the beginning of the study) were obtained from Marshall Farms (North Rose, New York, NY). The animals were housed in a dedicated dog unit at 20 (±5)°C with 50 (±30)% relative humidity, 12-h light/dark exposure, and ≈ 12 cycles of filtered, non-recycled air/h. The dogs were group-housed by sex and treatment after randomization. Dogs were fed 125 C3 pelleted diet (SAFE, Augy, France) once per day. Studies were conducted in accordance with the current guidelines for animal welfare. The procedures used in the study were reviewed and approved by CiToxLAB France institutional ethics committee before the initiation of the study.
Treatments
Groups of three male and three female Beagle dogs were treated orally from Day 1 of the study for 4 weeks. One group was treated daily with 25 mg/kg cyclosporine (Neoral®; Novartis Pharma SAS, Rueil-Malmaison, France). The second group of animals was treated with 2 mg/kg cyclophosphamide (Sigma-Aldrich, St. Quentin-Fallavier, France) on 4 consecutive days each week (namely Days 1–4, Days 8–11, Days 15–18, and Days 22–25 of the study) in combination with 20 mg furosemide (Dimazon®; Intervet, Angers, France) once a day orally as a preventive measure to avoid renal disturbances and hemorrhagic cystitis. Finally, the third group (control group) was given the vehicle (drinking water) under the same volume (2.5 ml/kg) as the treated groups daily.
General toxicity evaluation
Each animal was checked daily for mortality or clinical signs of toxicity during the study. The body weight of each animal was recorded before the beginning of the treatment period, then on the first day of treatment, and once a week until the end of the study. The amount of food consumed by each animal was estimated daily for 7 days before the beginning of the treatment period and then throughout the study.
Standard hematology parameters (total and differential blood cell counts, and coagulation parameters) were determined for all animals in pre-test, and then on Days 11, 18, and 28. Standard clinical chemistry parameters were determined once before the beginning and once at the end of the treatment period.
Immunotoxicity evaluation
All the animals in each treatment group were injected intramuscularly (IM) once with 5 mg KLH (Imject®, Thermo Scientific, Waltham, MA) on Day 11 of the study. Blood samples were drawn pre-test, and then on Days 18, 23, and 28; anti-KLH IgM primary antibody responses were assessed using pre-test, Days 18 and 23 samples, and anti-KLH IgG primary antibody responses using those from pre-test and Days 23 and 28. Blood lymphocyte subset immunophenotyping was also performed on blood taken pre-test, and on Days 11, 18, and 28 of the study. Lymphocyte subsets were characterized with the PE-LSM 11.425 (B-cells), APC-LSM 8.358 (total T-cells), PE-LSM 12.125 (CD4+ T-cells), and FITC-LSM 1.140 (CD8+ T-cells) antibodies (BD Pharmingen, France). All cells were analyzed using a Navios flow cytometer and the data were analyzed using the Navios v.1 software (Beckman Coulter, France).
Serum anti-KLH antibodies were quantified with a sandwich ELISA assay using peroxidase-conjugated anti-dog IgM or IgG and standards for curve calibration and analytical quality controls prepared with purified canine polyclonal anti-KLH IgM and IgG. Briefly, three naïve dogs were immunized IM with 5 mg KLH. Specific polyclonal anti-KLH IgM and IgG solutions were prepared from pooled serum samples by KLH-coupled NHS sepharose chromatography and then protein A affinity chromatography. These anti-KLH immunoglobulin solutions were characterized by 2-D gel electrophoresis and quantified for the preparation of the standard (STD) solutions, and quality control (QC) solutions for the calibration of the ELISA response curves. Standard curves ranged from 10.24–10,000 ng/ml of anti-KLH IgM or IgG, included two anchor points, and were fitted to a 4PL non-linear regression model. Standard curves tested on three independent assays were within acceptance criteria. The whole analytical procedure was validated for specificity, precision and accuracy, repeatability, sample dilution, and short-term, long-term, and freeze–thaw cycle analyte stability. The concentration level of 20 ng/ml was validated as the lower limit of quantification (LLOQ) and the concentration level of 5000 ng/ml as the upper limit of quantification (ULOQ). No non-specific binding for IgG and minimal non-specific binding in a few animals for IgM were observed in dog serum samples taken before the start of treatment and KLH immunization.
On completion of the treatment period, the animals were euthanized by an intravenous injection of sodium thiopental. A complete macroscopic post-mortem examination was then performed on all animals. Histological slides were prepared from bone marrow, thymus, spleen, mandibular and mesenteric lymph nodes, gut- and bronchi-associated lymphoid tissue, embedded in paraffin wax, sectioned at a thickness of ≈ 4-µm, and stained with hematoxylin and eosin (H&E).
Statistical analysis
Both means and standard deviations were calculated for each group whenever at least three data were available. The statistical significance of differences between groups (at p-values of < 0.05) was assessed using a Dunn test.
Results
No unscheduled deaths occurred during the study. Mild-to-moderate unspecific clinical signs were observed in a few animals and were not considered to reflect significant general toxicity or immunotoxicity. Body weight and food consumption were not affected by the treatment. No effects on red blood cells or coagulation parameters were noted in any group, and no changes in white blood cell counts in animals treated with cyclosporine. In cyclophosphamide-treated animals, slightly lower mean white blood cell counts were observed on Days 11, 18, and 28 in both males and females. These decreases reflected lower mean lymphocyte and neutrophil counts (they reached up to −37% and −64% in males and −33% and −42% in females of pre-dose values, respectively; when compared to controls, the decreases were up to −28% and −52% in males and −44% and −42% in females of mean control values, respectively) ().
Table 1. Male (n = 3) and female (n = 3) Beagle dogs treated orally with water (controls), 25 mg/kg cyclosporine daily, or 2 mg/kg cyclophosphamide 4 days/week from Day 1 of a 4-week treatment period. WBC, neutrophil, and lymphocytes were counted by ADVIA120 and lymphocyte subset counts (B-cells, total T-cells, as well as CD4+ and CD8+ T-cells) were analyzed by flow cytometry, before treatment, and on Days 11, 18, and 28 of this 4-week treatment period.
No consistent effects of cyclosporine on lymphocyte subsets were observed (). In cyclophosphamide-treated dogs, absolute counts of B-cells were markedly decreased (down by ≈ −50%) from Day 11 until the end of the treatment, and the observed decreases were somewhat greater in females than in males. Slightly lower total T-cell counts were also noted in both males and females from Day 11 until the end of the treatment, and associated with slight reductions in CD4+ T-helper cell counts and CD8+ T-cytotoxic cell counts.
High IgM and IgG primary responses were observed in control dogs, with maxima seen 7 (Day 18) and 17 (Day 28) days post-KLH injection, respectively (, ). Compared to controls, both anti-KLH IgM and IgG levels were similar in cyclophosphamide-treated dogs. Anti-KLH IgM levels were significantly lower by −68% on Day 18, and −71% on Day 23 in females treated with cyclosporine, but remained within the range of control values in cyclosporine-treated males, even though slightly lower mean values were noted on Days 18 and 23 (of −37 and −22%, respectively) when compared to controls. Anti-KLH IgG levels were significantly decreased in both males and females treated with cyclosporine. Indeed, when compared to controls, all animals treated with cyclosporine had lower anti-KLH IgG levels on Day 23 (mean values −67% in males and −94% in females) and on Day 28 (mean values −80% in males and −96% in females).
Table 2. Anti-KLH IgM and IgG mean responses. Male (n = 3) and female (n = 3) Beagle dogs treated orally with water (controls), 25 mg/kg cyclosporine daily, or 2 mg/kg cyclophosphamide 4 days/week from Day 1 of a 4-week treatment period, were injected intramuscularly with 5 mg KLH on Day +11. Serum anti-KLH IgM and IgG were measured using a sandwich ELISA before treatment and subsequently on Days +18 and +23 (to measure IgM anti-KLH antibody response) and on Days +23 and +28 (to measure IgG anti-KLH antibody response).
Figure 1. Anti-KLH IgM and IgG individual responses. Male (n = 3) and female (n = 3) Beagle dogs treated orally with water (controls), 25 mg/kg cyclosporine daily, or 2 mg/kg cyclophosphamide 4 days/week from Day 1 of a 4-week treatment period, were injected intramuscularly with 5 mg KLH on Day +11. Serum anti-KLH IgM and IgG were measured using a sandwich ELISA before treatment and subsequently on Days +18 and +23 (to measure IgM anti-KLH antibody response) and on Days +23 and +28 (to measure IgG anti-KLH antibody response).
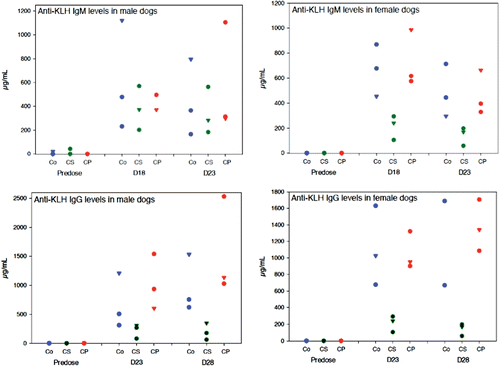
There were no treatment-related organ weight changes in dogs treated with cyclosporine or cyclophosphamide (data not shown). The expected microscopic changes in the thymus were observed in cyclosporine-treated animals and consisted of a slight-to-marked increased cortex/medulla ratio. This finding was characterized by an extension of the cortex that replaced the medullary area, leaving only some islets of the medulla. The thymus was then almost only composed of small thymocytes. There was a decrease in germinal center development (in terms of number and/or size) in the mesenteric lymph nodes of one male treated with cyclosporine and in two females treated with cyclophosphamide. The expected microscopic changes in the kidneys of cyclophosphamide/furosemide-treated animals consisted of tubular basophilia, characterized by cytoplasmic basophilia, less differentiated epithelial cells, anisokaryosis, and no or short brush border. The tubules located in the medullary rays were more severely affected. Occasional tubular single cell necrosis/degeneration was also noted.
Discussion
Despite the key role of dog studies in the non-clinical safety evaluation of drug candidates, few data are available on techniques and methods applicable to immunotoxicity dog studies (Thiem et al., Citation1988; Jones et al., Citation2000; Finco-Kent and Kawabata, Citation2005; Lebrec et al., Citation2012). The aim of the present study was to test whether widely accepted immunotoxicity endpoints including lymphocyte subset immunophenotyping, the anti-KLH TDAR assay, and histological examination of the main lymphoid organs were reliable to detect immunosuppression induced by cyclosporine and cyclophosphamide in dogs and could therefore be used for non-clinical immunotoxicity evaluation in this species.
Cyclosporine (Calne, Citation1979; Takaori et al., Citation1992) and cyclophosphamide (Putnam et al., Citation1975; Medleau et al., Citation1983) were selected as reference agents as they were previously shown to exert immunosuppressive effects in the dog. The dose of cyclosporine used in this study (25 mg/kg) induced a statistically significant decrease in anti-KLH antibody response in Beagle dogs (Finco-Kent and Kawabata, Citation2005), and the dose of cyclophosphamide (2 mg/kg) has previously been shown to induce a statistically significant decrease in humoral immune response to sheep red blood cells, but no prolongation of renal homograft survival in mongrel dogs (Putnam et al., Citation1975).
No consistent effects of cyclosporine on peripheral blood lymphocyte subsets were noted in the present study, and this confirmed previous findings (Takaori et al., Citation1992). In contrast, decreased anti-KLH IgM and IgG levels were observed and again this is consistent with previously published data (Finco-Kent and Kawabata, Citation2005). Finally, cyclosporine was shown to induce expected histological changes in the thymus of treated dogs (Beschorner et al., Citation1987).
In the present study, cyclophosphamide induced a marked decrease in B-cell counts and less pronounced decreases in CD4+ T-cells and CD8+ T-cells. These findings may be explained by the direct cytotoxic effect of cyclophosphamide, which is reflected by lower mean lymphocyte and neutrophil counts in treated dogs as compared to pre-test values or control animals. In contrast, cyclophosphamide induced no changes in primary anti-KLH IgM and IgG responses. These somewhat unexpected findings might be explained by the use of KLH as the T-dependent antigen. Indeed, there is a large body of evidence that cyclophosphamide can decrease humoral immune responses to sheep red blood cells (SRBC) (Shand, Citation1979). SRBC are particulate antigens, whereas KLH is a soluble protein, and this difference has been suggested to account for the apparent reduced sensitivity of TDAR assays using KLH vs TDAR assays using SRBC or differences in sensitivity across mouse and rat strains (White et al., Citation2007). It can be hypothesized that dogs are also a less-sensitive species, which however remains to be fully substantiated. Another explanation for these findings is the schedule of administration: indeed, in the present study, dogs were treated for two 4-day cycles prior to KLH injection. Cyclophosphamide was shown to inhibit the function of Foxp3+ regulatory T-cells (Brode and Cooke, Citation2008), thus providing a possible mechanism for the long-recognized finding that, depending on the dose used and the timing of administration with respect to antigen injection, cyclophosphamide can exert either immunosuppressive or immunoenhancing effects (Shand, Citation1979).
The results of this study suggest that the dog can be considered a relevant species for non-clinical immunotoxicity evaluation.
Declaration of interest
The authors report no conflicts of interest. The authors alone are responsible for the content and writing of the paper.
References
- Beschorner, W. E., Namnoum, J. D., Hess, A. D., Shinn, C. A., Santos, G. W. 1987. Cyclosporin A and the thymus. Immunopathology. Am. J. Pathol. 126:487–496.
- Brode, S., Cooke, A. 2008. Immune-potentiating effects of the chemotherapeutic drug cyclophosphamide. Crit. Rev. Immunol. 28:109–126.
- Calne, R. Y. 1979. Pharmacological immunosuppression in clinical organ grafting. Observations on four agents: Cyclosporin A, Asta 5122 (cytimun), lambda carrageenan and promethazine hydrochloride. Clin. Exp. Immunol. 35:1–9.
- Finco-Kent, D., Kawabata, T. T. 2005. Development and validation of a canine T-cell-dependent antibody response model for immunotoxicity evaluation. J. Immunotoxicol. 2:197–201.
- Haggerty, H. G. 2007. Immunotoxicity testing in non-rodent species. J. Immunotoxicol. 4:165–169.
- ICH. 2005. ICH Guideline S8 on Immunotoxicity Studies for Human Pharmaceuticals. Available online at: http://www.ich.org/products/guidelines/safety/article/safety-guidelines.html.
- Jones, R. D., Offutt, D. M., Longmoor, B. A. 2000. Capture ELISA and flow cytometry methods for toxicologic assessment following immunization and cyclophosphamide challenges in beagles. Toxicol. Lett. 115:33–44.
- Lebrec, H., O'Lone, R., Freebern, W., Komocsar, W., Moore, P. 2012. Survey: Immune function and immunotoxicity assessment in dogs. J. Immunotoxicol. 9:1–14.
- Medleau, L., Dawe, D. L., Calvert, C. A. 1983. Immunosuppressive effects of cyclophosphamide, vincristine, and L-asparaginase in dogs. Am. J. Vet. Res. 44:176–180.
- Putnam, C. W., Halgrimson, C. G., Groth, C. G., Kashiwagi, N., Porter, K. A., Starzl, T. E. 1975. Immunosuppression with cyclophosphamide in the dog. Clin. Exp. Immunol. 22:323–329.
- Shand, F. L. 1979. The immunopharmacology of cyclophosphamide. Int. J. Immunopharmacol. 1:165–171.
- Takaori, K., Nio, Y., Inoue, K., Tun, T., Fukumoto, M., Hashida, T., Yasuhara, M., Hori, R., Tobe, T. 1992. A comparative study on immunosuppressive effects of cyclosporin A and FK 506 on peripheral blood lymphocytes in dogs. Biotherapy 4:129–137.
- Thiem, P. A., Halper, L. K., Bloom, J. C. 1988. Techniques for assessing canine mononuclear phagocyte function as part of an immunotoxicologic evaluation. Int. J. Immunopharmacol. 10:765–771.
- White, K. L. Jr., Sheth, C. M., Peachee, V. L. 2007. Comparison of primary immune responses to SRBC and KLH in rodents. J. Immunotoxicol. 4:153–158.