Abstract
The immunotoxic potential of trichloroethylene (TCE) and perchloroethylene (PERC) was assessed after inhalation exposure through the evaluation of the antibody forming cell (AFC) response to sheep red blood cells (SRBC). Female Sprague-Dawley rats were exposed to TCE or PERC vapor at 0, 100, 300, or 1000 ppm for 6 h/day, 5 days/week for 4 weeks (20 exposure days). Additional 0 ppm control groups were included and were dosed with cyclophosphamide via intraperitoneal injection to serve as positive immunosuppressive controls in the SRBC assay. Additional end-points evaluated included liver, kidney, spleen, and thymus weights, hematology, cellular differentials in bronchoalveolar lavage fluid, histopathology of select tissues, and assessment of the phagocytic activity of pulmonary alveolar macrophage (PERC only). Exposure to the high concentration of TCE (1000 ppm) resulted in increases in relative liver and kidney weights and suppression of AFC responses (AFC/spleen and AFC/106 spleen cells) by ≈ 70%, while no treatment-related effects were noted at 100 and 300 ppm. Animals exposed to PERC at levels of 300 or 1000 ppm had statistically significant increases in relative liver weights that were accompanied by very slight hypertrophy of the centrilobular hepatocytes. Animals exposed to PERC did not demonstrate a treatment-related effect on the AFC response and no effect was noted on the phagocytic activity of pulmonary alveolar macrophages. The results of these studies indicate that TCE had immunotoxic potential only at high exposure concentrations (1000 ppm), while PERC, at similar exposure concentrations, did not display any evidence of immunotoxicity.
Introduction
Trichloroethylene (TCE) and perchloroethylene (PERC) are two commercially important chlorinated hydrocarbon solvents that are primarily used as chemical intermediates but are also used as metal cleaning and degreasing agents and in the dry cleaning of textiles. Their volatile nature has the potential of contributing to human and environmental exposure and therefore extensive evaluations on the hazard and risk potential of these chemicals have been completed (ATSDR, 1997a, b). However, further examination of the immunotoxic potential of TCE has been identified as priority data need by the Agency for Toxic Substances and Disease Registry (ATSDR).
Numerous non-guideline studies have reported variable immune-related effects for TCE using laboratory animals. CD-1 female mice demonstrated statistically suppressed antibody responses to sheep red blood cells (SRBC) following a 4-month exposure to 2.5 and 5 mg/ml TCE in drinking water ( Citation Sanders et al., Citation1982). Subsequent studies in the same laboratory employing 14-day gavage administration found no effects on the immune system in female B6C3F1 and male CD-1 mice at doses up to 800 mg/kg/day ( Citation NTP, Citation1992a, b, c). A more recent developmental immunotoxicity study reported reductions in antibody responses to SRBC in B6C3F1 mice following 3 and 8 weeks of exposure to drinking water containing TCE at concentrations of 1.4 and 14 ppm ( Citation Peden-Adams et al., Citation2006). Citation Aranyi et al. (Citation1986) reported that mice exposed to TCE by inhalation for 3 h at ≥ 10 ppm became more susceptible to infection by Streptococcus zooepi-demicus administered simultaneously as an aerosol, while pulmonary bactericidal activity against [35S]-Klebsiella pneumonia appeared unaffected. Another study reported reduced serum IgG antibody levels in MRL-lpr/lpr male mice (a mouse model for systemic autoimmunity) during Weeks 2–8 of a TCE inhalation exposure at 2000 ppm (4 h/day, 6 days/week) ( Citation Kaneko et al., Citation2000). This study also reported histopathological changes in the spleen following TCE vapor exposure at 500 ppm, while no changes were observed in thymus morphology at any exposure level. These effects are in contrast to the findings of another study that found no histopathological effects in the spleens from rats and mice following 6 weeks of inhalation exposure to 700 ppm TCE (8 h/day, 5 days/week) ( Citation Prendergast et al., Citation1967).
In comparison to TCE, there have been fewer studies conducted to specifically examine the immunotoxic potential of PERC. In one study, immunological effects were observed in female B6C3F1 mice exposed to a mixture of 25 chemicals in drinking water for 14 or 90 days ( Citation Germolec et al., Citation1989). At the highest dose level examined, mice exhibited a reduced response in the SRBC assay and an increased host susceptibility to infection by Plasmodium yoelii. The maximum concentration of PERC in this study was 6.8 ppm but, because the study involved assessment of a mixture, the contribution of PERC to this effect cannot be assessed. Another study reported that mice exposed for 3 h to an atmosphere of PERC at 50 ppm showed an increase in susceptibility to lung infection by S. zooepidemicus and decreased pulmonary bactericidal activity to K. pneumoniae ( Citation Aranyi et al., Citation1986). However, the significance of this result is uncertain due to the variability in the responses observed in control animals and the overall relevance of this response to humans (ATSDR, 1997a; ECETOC, 1999). Studies in rats and mice reported that PERC did not alter hepatic or splenic immune lymphocytotoxic activities in vivo, although an inhibitory effect was noted in vitro ( Citation Schlichting et al., Citation1992). Immunological monitoring of dry-cleaning workers has suggested potential effects on the response of the respiratory immune system, such as alveolar macrophage activity ( Citation Andrys et al., Citation1997). However, overall there are no consistent data from repeat-exposure general toxicity studies to indicate that PERC elicits effects on immune organs (NTP, 1986) and, therefore, the immunotoxicity potential of PERC cannot be judged using the current, limited dataset (WHO, 2006).
Due to the inconsistencies in the above studies, additional data were considered necessary to more definitively assess the immunotoxic potential of TCE and PERC. The purpose of the present studies was to further assess the immunotoxic potential of these chemicals under guideline study conditions through the evaluation of the antibody response to sheep red blood cells (SRBC). For TCE, the study was conducted to fill the priority data need as identified by the ATSDR. In the case of PERC, the study was conducted as part of voluntary programs to fill data needs for the ATSDR and the Voluntary Children’s Chemical Evaluation Program (VCCEP). As human exposure to these chemicals is most likely to occur through inhalation, discussions with the ATSDR concluded that this was the most relevant route of exposure for this evaluation.
Materials and methods
Animals
Female Sprague-Dawley rats (Crl:CD®(SD)IGSBR); Charles River Laboratories, Inc., Portage, MI), 7–8-weeks-of-age at study start, were used in the evaluation. The rats were VAF/Plus® (Virus Antibody Free) and were selected because of their general acceptance and suitability for toxicity testing, availability of immunotoxicology background data, and the reliability of the commercial supplier. Rats were housed in stainless steel cages and were provided LabDiet Certified Rodent Diet (PMI Nutrition International, St. Louis, MO) and water ad libitum, except during inhalation exposures. All rats were fed and handled in compliance with standards set forth by the US Animal Welfare Act and according to the recommendations of the National Institutes of Health ‘Guide for the Care and Use of Laboratory Animals’ in facilities fully accredited by the Association for Assessment and Accreditation of Laboratory Animal Care International (AAALAC International). The procedures performed on the rats were reviewed and approved by a veterinarian and the Institutional Animal Care and Use Committee (IACUC).
Test chemicals
TCE was obtained from The Dow Chemical Company (Freeport, TX) and was 99.99% pure as determined by gas chromatography analysis. PERC was obtained from The Dow Chemical Company (Harvey, LA) and was 99.98% pure as determined by gas chromatography analysis. Cyclophosphamide (CYP; cyclophosphamide monohydrate) was obtained from Sigma-Aldrich (St. Louis, MO). Sheep red blood cells (SRBCs) were obtained from Covance Research Products Inc. (Denver, PA); all culture media and supplements were purchased from Life Technologies (Grand Island, NY).
Test chemical exposure generation
The animals were exposed to filtered air (0 ppm; control), TCE, or PERC vapors in 4 m3 stainless steel and glass Rochester-type whole-body inhalation exposure chambers. Chamber airflow was maintained at ≈900 L/min (12–15 calculated air changes per hour). Target exposure concentrations of TCE or PERC were generated using the glass J-tube method. Liquid test material was metered into the J-tube assemblies (one per exposure chamber), vaporized in an inert nitrogen atmosphere, and then mixed and diluted with filtered chamber supply air to achieve the target chamber concentration of TCE or PERC. The control chambers (0 ppm) received the same volumetric flow of nitrogen as the TCE or PERC exposure chambers. Prior to study initiation, each whole-body chamber was tested for exposure atmosphere homogeneity from at least three sample points in the breathing zone of the animals and from the reference sampling point of the chamber. Variation in test atmosphere concentration, compared to the reference sampling point, was < 10%. In addition, the position of each exposure cage was altered daily to further ensure uniform exposure of all animals in the whole-body exposure chamber throughout the study. During exposure, the chambers were monitored for oxygen content, which remained ≥ 20% O2 at all times. The chamber concentrations of TCE or PERC were analytically determined at least once per hour with a Miran 1A infrared (IR) spectrophotometer (Foxboro/Wilks, South Norwalk, CT) that sampled from the breathing zone of rats in each exposure chamber. The IR spectrophotometer was calibrated and a standard curve was compiled prior to and at the end of the study, using standards prepared by vaporizing measured volumes of TCE or PERC into Tedlar® sample bags (Series 233, SKC, Eight-Four, PA) along with metered volumes of dry, compressed air. A computer-based data acquisition and control system (CAMILE® TG Camile Products, LLC, Indianapolis, IN) was used to calculate and store the TCE or PERC chamber concentrations, and to record chamber airflow, temperature, and relative humidity data.
Study design and end-points
The TCE and PERC studies were conducted as independent investigations following the principles outlined in the US Environmental Protection Agency Health Effects Test Guideline for Immunotoxicity (OPPTS 870.7800). Dose levels were selected to result in some level of general toxicity without resulting in overt toxicity and/or stress that may preclude the assessment of immunotoxicity and to best inform downstream risk assessment analyses. Doses were selected based on a review of previous toxicity studies (ATSDR 1997a, b; ECETOC, 1999). The high dose of 1000 ppm was selected to produce moderate toxicity, including slight effects on body weight and liver and/or kidney weights. The low dose was selected to target a no-observable-effect-level for the study and the mid-dose to characterize any dose-related effects on the evaluated end-points. The high dose for both materials was also in excess of the limit-dose specified in the EPA OPPTS 870.7800 guideline on immunotoxicity testing.
In each study, there were groups of rats for the analysis of organ weights, histopathology, hematology, and bronchoalveolar lavage (n = 8/group) and separate groups for the analysis of the antibody response (n = 8/group; ). Rats were exposed to TCE or PERC vapor at 0, 100, 300, or 1000 ppm for 6 h/day, 5 days/week for 4 weeks (20 exposure days). For the assessment of the antibody response, a second 0 ppm group injected with cyclophosphamide (20 mg/kg; see SRBC methods) was included which served as the positive immunosuppressive control in the SRBC assay. A CYP positive control group for evaluation of BAL, hematology, histopathology, and organ weights was not included in the PERC study.
Table 1. General design for TCE and PERC inhalation studies outlining separate groups of animals for evaluation of the SRBC antibody response.
One day following the last exposure, all animals were anesthetized by the inhalation of CO2/O2, weighed, and blood samples were obtained from the orbital sinus (n = 8 per group). Their tracheas were exposed and clamped, and the animals were euthanized by decapitation. A complete necropsy was conducted on all animals by a veterinary pathologist assisted by a team of trained individuals. The liver, kidneys, thymus, spleen, lung (TCE only), and brain (PERC only) were trimmed and weighed immediately. The ratios of organ weight to terminal body weight were calculated.
Bronchoalveolar lavage (BAL)
The lungs with attached trachea from all animals not designated for the SRBC assay were canulated. For the TCE study, the left extra-pulmonary bronchus was temporarily clamped, and the right lung lobes were lavaged with five volumes of sterile pyrogen-free saline. For the PERC study, the entire lung was lavaged with eight volumes of sterile, pyrogen-free saline. Total nucleated cells/ml lavage fluid was determined using a hemocytometer. BAL cells were recovered from lavage fluid by centrifugation and the cell-free supernatant was processed for total protein and lactate dehydrogenase (LDH) determinations using a Hitachi 914 Clinical Chemistry Analyzer. In some instances, due to low cell yields, lavage samples from rats were combined between two animals from each group. In these instances, the individual cell pellets were suspended using RPMI 1640 culture media, combined, and centrifuged again. Cell smears were made with a cytocentrifuge and stained with Diff-Quick (modified Giemsa stain). Differential cell counts for BAL fluid were determined by counting 200 cells per animal. The number of each cell type from recovered lavage fluid (e.g., neutrophil, macrophage, eosinophil, and lymphocyte) was estimated by multiplying the total recovered cells for each sample by the percent occurrence of that cell type in the corresponding differential count.
BAL cell phagocytosis
For the PERC study, the phagocytic activity of the BAL cells was also assessed. Lavage fluid from each sample was centrifuged at 300 x g for 10 min at 4°C and the cell pellets were re-suspended with 1 ml Dulbecco’s Modified Eagle Medium (DMEM) containing 10% fetal bovine serum (FBS), 1% penicillin/streptomycin and 2.5% HEPES (DMEM +). The total number of cells/ml was determined using a Coulter Z1 cell counter and the cell concentration was adjusted to a concentration of ~1 × 106 cells/ml. Five hundred microliters from each lavage preparation was incubated for 20 min in a rotating waterbath at 37°C (experimental) or in ice at 4°C (background), with gentle vortexing every 10 min. At the end of 20 min, 5 × 107 1-µm diameter fluorescent carboxylate latex beads (Polysciences, Inc., Warrington, PA) in a volume of 5 µl (prepared in DMEM +) were added to each tube. Incubations at 37°C (rotating waterbath) and 4°C (ice) resumed for an additional 2 h with gentle vortexing every 10 min. After the 2-h period, the sides of the tubes were gently scraped using polypropylene disposable spatulas. Each sample was then gently layered atop 3 ml gradient buffer (DMEM + containing 2% FBS and 2% bovine serum albumin) and centrifuged immediately at 150 x g for 10 min at room temperature. The supernatant was then aspirated and discarded. To determine cell viability, 1 ml propidium iodide (1 µg/ml) solution was added and the samples incubated at 4°C in the dark for 10 min. After incubation, the samples were centrifuged at 150 x g for 10 min at room temperature and the supernatant discarded. The cell pellets were washed with 0.5 ml buffer (IsoFlow, Beckman Coulter, Indianapolis, IN) centrifuged at 150 x g for 10 min at room temperature and the supernatant discarded. The cell pellets were then suspended in 1 ml of buffer containing 0.5% formalin.
Slides were prepared by centrifuging 200 µl of each formalin-fixed sample (4°C and 37°C) at 400 rpm for 10 min using a Cytospin II cytocentrifuge (Shandon, Pittsburgh, PA). To facilitate visualization of the cells, one drop of ProLong® Gold antifade reagent with DAPI stain (Life Technologies) was added to each slide. Slides were subsequently evaluated for cellular phagocytic capability based upon fluorescent microscopic evaluations. A Leitz Model DMRX epifluorescence microscope (Leica Microsystems Inc. Buffalo Grove, IL) was used to count a total of 300 macrophages (400× final magnification) and the percentage of cells containing fluorescent beads (with the 4°C background control subtracted) was determined. Cells staining positive for PI were excluded. In order to thoroughly assess any alterations in phagocytic ability, cells containing beads were further categorized as cells containing 1–4 beads and ≥ 5 beads.
Histopathology
After bronchoalveolar lavage, the entire lung was inflated with 10% neutral phosphate-buffered formalin and placed in a large volume of the same fixative. For both TCE and PERC, the spleen, thymus, mediastinal and tracheobronchial lymph nodes, and left lung lobes were collected from each rat not dedicated for the SRBC antibody response and were preserved in 10% neutral phosphate-buffered formalin for histopathologic evaluation. For PERC, the liver, trachea, nasal tissue, and bone marrow of the sternum and femur/tibia were also collected and evaluated histopathologically. Paraffin embedded tissues were sectioned (~ 6-µm thick), stained with hematoxylin and eosin, and examined by a Board Certified Veterinary Pathologist. Selected findings were graded to reflect severity of lesions to evaluate: (1) contribution of a specific lesion to the health status of an animal; (2) exacerbation of common naturally occurring lesions as a result of the test material; and (3) dose–response relationships for treatment-related effects.
Hematology
Blood samples, collected from the orbital sinus during necropsy, were mixed with ethylenediamine-tetraacetic acid (EDTA) and hematologic parameters were assayed using an Advia 120 Hematology System (Bayer Corporation, Tarrytown, NY). Evaluated parameters included hematocrit (HCT), hemoglobin (HgB) concentration, red blood cell (RBC) count, total white blood cell (WBC) count, platelet (PLAT) count, differential WBC count, red blood cell indices, mean corpuscular hemoglobin (MCH), mean corpuscular volume (MCV), mean corpuscular hemoglobin concentration (MCHC), and reticulocyte count (RET; PERC only).
SRBC assay
The immunotoxic potential of TCE and PERC was assessed through the evaluation of the antibody forming cell (AFC) response to sheep red blood cells (SRBC). Four days prior to sacrifice (i.e. Day 25), rats designated for the SRBC assay were immunized with a single intra-venous (IV) injection of 2 × 108 SRBC (in 0.5 ml) via the lateral tail vein. On Days 25–28, the positive control group for the assay was injected intraperitoneally (IP) with cyclophosphamide (CYP; Sigma) at 20 mg/kg. Approximately 24 h after the last inhalation exposure (i.e. Day 29), rats were euthanized via CO2 asphyxiation followed by cervical dislocation. Body weights were recorded and spleens were surgically removed and placed into tubes containing 5% FBS/RPMI 1640.
Single cell suspensions of the spleens were prepared by gentle disaggregation using a tissue homogenizer (Stomacher® 80 Lab System, Seward Ltd., London, UK). The cells were washed twice in 5% FBS/RPMI, spun down at 300 x g for 10 min, and the cell pellet was re-suspended in 5 ml of 5% FBS/RPMI. A 1:1000 dilution of the spleen cells was prepared in 10 ml of Isoton II® and cell counts then determined using a Coulter Z1® cell counter (Beckman Coulter, Miami, FL) with three drops of Zap-o-Globin (Beckman Coulter, Fullerton, CA) added to lyse red blood cells. Three dilutions of spleen cells were prepared (i.e. 1:50, 1:100, or 1:200) using 5% FBS/RPMI and were mixed with washed SRBC (same lot used for immunization) and guinea pig complement (Accurate Chemical & Scientific Corp., Westbury, NY). The mixture was poured into the middle of a Petri dish, a humidified coverslip was placed on the mixture, and after ≈ 1 min the dish was placed in a dry incubator set at 37°C. Each dish was removed from the incubator 3 h later and total plaques counted. Plaques were formed as a result of complement-mediated lysis of the SRBC by antibodies secreted by the plasma cells (differentiated B-cells). Calculations for this assay included determination of AFC/spleen and AFC/106 spleen cells.
Statistics
Descriptive statistics only (means and standard deviations) were determined for chamber concentration, temperature, humidity, and airflow, body weight gains, RBC indices and WBC differential counts, BAL differential cell counts (TCE only), and BAL macrophage phagocytic activity data (categorized by number of beads/macrophage; 1–4 or ≥ 5; PERC only). An outlier test was run for all continuous data.
AFC response data, body weight, food consumption, appropriate hematologic data, terminal body weight, organ weights (absolute and relative), BAL total cell and differential cell data, total protein, LDH levels, and total macrophage phagocytic activity were first evaluated by Bartlett’s test (α = 0.01) for equality of variances. Based upon the outcome of Bartlett’s test, a parametric or non-parametric analysis of variance (ANOVA) was performed. If the ANOVA was significant at α = 0.05, a Dunnett’s test (α = 0.05), or the Wilcoxon Rank-Sum (α = 0.05) test with Bonferroni’s correction was performed.
Results
Trichloroethylene
Exposure concentrations and in-life data
Mean chamber concentration values for the study were 0, 97.8 (± 2.0), 297.9 (± 1.4), and 1017.4 (± 24.2) ppm vs the targeted values of 100, 300, and 1000 ppm, respectively. All rats survived to the scheduled necropsy and there were no treatment-related clinical observations that were attributable to TCE exposure.
TCE exposure resulted in a concentration-dependent reduction in body weight and body weight gain throughout the study. Effects on body weight were statistically identified as decreased on Day 17 in animals exposed to 300 ppm or 1000 ppm TCE. This decrease was preceded by suppressed body weight gains during the first 2 weeks of exposure and was consistent with reduced food consumption in these groups during the first 2 weeks of the study. Notably, feed consumption and weight gain for all TCE exposed groups were similar to controls over the last 2 weeks of the study and body weights were not statistically different from controls over these last 2 weeks, including the final body weights.
Clinical and anatomic pathology
There were no changes in any of the hematological parameters, including differential white blood cell counts, in TCE exposed animals. The positive control CYP induced statistically significant decrements in white blood cell and red blood cell counts, hemoglobin content, hematocrit, and in platelet counts. The differential white blood cell counts showed a modest relative increase in the percentage of neutrophils in the CYP-treated group, which was due to a decrement in lymphocyte counts (data not shown).
There were no treatment-related effects on lung, spleen, or thymus weights; however, rats exposed to 1000 ppm TCE displayed 13% and 17% increases in relative liver and kidney weights, respectively, which were statistically significant relative to controls (). Statistically significant decreases in the weights of lymphoid organs (spleen and thymus) were only seen in the CYP positive control group.
Table 2. Terminal body weights and absolute and relative organ weights for animals exposed to TCE.
There were no treatment-related gross pathologic observations for any animal in any of the TCE-exposed groups. There were no treatment-related histopathological changes in any of the organs examined that were associated with exposure to TCE. Animals in the CYP positive control group had histopathological changes that included slight or moderate atrophy of the mediastinal and tracheobronchial lymph nodes, slight or moderate atrophy of the white pulp of the spleen, and slight or moderate atrophy of the thymus.
Bronchoalveolar lavage (BAL)
Inhalation of TCE had no effect on BAL total protein or LDH levels, when compared to controls. Similarly, there were no alterations in differential cell counts (macrophage, neutrophils, lymphocytes, eosinophils) evaluated from BAL samples after exposure to TCE. Animals treated with CYP (and administered room air) demonstrated a distinctive cellular differential due to an influx of neutrophils (data not shown).
SRBC antibody-forming cell (AFC) assay
The immunotoxic potential of TCE was assessed through the evaluation of the antibody response to sheep red blood cells (SRBC). Animals exposed to TCE (all exposure levels) demonstrated a slight reduction in the number of spleen cells that lacked a dose response and was not statistically different from controls (). The reduced spleen cell numbers contributed to a slightly lower number of AFC/spleen in the 100 and 300 ppm groups; however, the 1000 ppm group showed a more pronounced and statistically significant 71% decrease in the AFC/spleen response (). When the AFC responses were normalized for spleen cell numbers (AFC/106 spleen cells), rats administered 100 ppm and 300 ppm TCE did not demonstrate suppression in AFC activity relative to controls (). In contrast, animals exposed to 1000 ppm TCE had a 64% decrease in the AFC/106 splenocytes response, relative to controls. Based on these data, TCE was considered to cause suppression in the antibody response to SRBC at 1000 ppm.
Figure 1. Results of the SRBC antibody forming cell (AFC) assay for TCE. Exposure to TCE resulted in a slight decrease in the number of spleen cells that was without dose response and was not statistically significant (a). No effect on the AFC responses was noted for the 100 and 300 ppm groups, while the 1000 ppm group had a 71% and 64% decrease in the (b) AFC/spleen and (c) AFC/106 splenocytes responses when compared to controls. The positive control CYP resulted in the expected decreases in spleen cell counts and AFC responses. Values represent the mean (± SE) of eight animals. * p < 0.05.
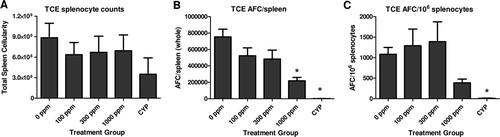
Animals administered room air but receiving IP injections of the positive control CYP (20 mg/kg for 4 days) demonstrated marked suppression in splenocyte numbers and AFC responses (). CYP treatment reduced spleen cell numbers by 60% and the number of AFC per spleen and per million splenocytes by > 99%.
Perchloroethylene
Exposure concentration and in-life data
Mean chamber concentration values for the study were 0, 104.4 (± 0.8), 304.5 (± 2.0), and 1010.1 (± 5.7) ppm vs targeted values of 100, 300, and 1000 ppm, respectively. All rats survived to the scheduled necropsy and there were no clinical observations that were attributable to PERC exposure.
A statistically significant reduction in mean body weight values was identified in rats exposed to 1000 ppm PERC when compared to their respective controls for the first 2 weeks of the study. As with the TCE study, this decrease was consistent with reduced body weight gains and food consumption during this period. Over the remainder of the study, feed consumption and weight gains for all PERC exposed groups were similar to controls and body weights were not statistically different from controls.
Clinical and anatomic pathology
There were no statistically significant changes in any of the hematologic parameters, including differential white blood cell counts for PERC-exposed rats when compared to their respective controls. Animals exposed to 300 or 1000 ppm had statistically significant treatment-related increases in mean relative liver weights of 8% and 10%, respectively, relative to the controls (). Although it did not reach a level of statistical significance in any exposure group, a minimal reduction in mean terminal body weights was noted as exposure concentrations to PERC increased.
Table 3. Terminal body weights and absolute and relative organ weights for animals exposed to PERC.
There were no treatment-related gross pathologic observations for any animal in any exposure group. The only treatment-related histopathologic alteration noted was very slight hypertrophy of centrilobular hepatocytes in four of eight rats exposed to 300 ppm, and in seven of eight rats exposed to 1000 ppm. The hepatocellular hypertrophy corresponded to the higher relative liver weights at these exposure concentrations, and was interpreted to be a non-adverse adaptive effect. Clinical and anatomic pathology end-points were not evaluated for CYP-treated animals in the PERC study.
Bronchoalveolar lavage (BAL)
There were no differences in the total protein concentration or LDH enzyme activity in the recovered BAL fluid of PERC-exposed animals, when compared to controls. Exposure to PERC did not result in statistically significant alterations in the total number, type, or percent occurrence of cells (macrophage, neutrophils, lymphocytes, eosinophils) present in BAL fluid.
Exposure to PERC at target concentrations of 100, 300, and 1000 ppm did not result in a statistically significant alteration in the ability of PAM to phagocytize fluorescent microspheres when compared to control animals ().
Figure 2. Phagocytic activity of pulmonary alveolar macrophages (PAM) following PERC exposure. Exposure to PERC did not result in any alterations in ability of PAM to phagocytize fluorescent bead(s) when compared to controls. The wide un-shaded (white) bars represent the total percentage of PAM containing any number of beads; the counts were further stratified into those containing 1–4 beads (black bars) and those containing ≥ 5 beads (grey bars) to further assess any reduction in activity. Values represent the mean (± SD) from cells of eight animals.
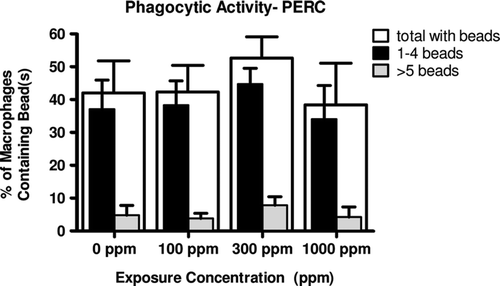
SRBC antibody-forming cell (AFC) assay
Animals exposed to PERC at concentrations of 100, 300, and 1000 ppm did not demonstrate a treatment-related reduction in the number of spleen cells or in AFC responses (). AFC responses were slightly variable across the dose groups, but within known and established variability for this endpoint in the literature ( Citation White et al., Citation2005; Citation Mann et al., Citation2008) and consistent with the lack of an immunosuppressive response to PERC. In contrast, animals administered room air but receiving IP injections of the positive control CYP (20 mg/kg for 4 days) demonstrated a marked reduction in splenocyte numbers and a suppression of the AFC responses. CYP treatment reduced spleen cell numbers by 71% and the number of AFC per spleen and per million splenocytes by > 99%.
Figure 3. Results of the SRBC antibody forming cell (AFC) assay for PERC. Exposure to PERC did not result in a treatment-related effect on the (a) number of spleen cells or (b and c) AFC responses. The positive control CYP resulted in the expected decreases in spleen cell counts and AFC responses. Values represent the mean (± SE) of eight animals. * p < 0.05.
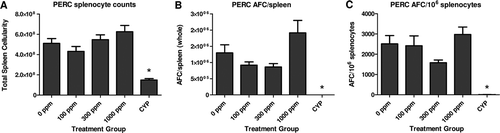
Discussion
The present studies were conducted to further assess the immunotoxic potential of TCE and PERC under guideline study conditions through the evaluation of the antibody response to SRBC after inhalation exposure. The SRBC antibody response is one of the few immunotoxicological end-points that requires all of the cellular components of a classical immune response (e.g. B-cells, T-cells, macrophages), and thus is a sensitive indicator of the immunotoxic potential of a chemical. The results of these studies indicate that TCE has immunotoxic potential only at high exposure concentrations (1000 ppm), while PERC did not display any evidence of immunotoxicity.
The liver and kidney are well-documented target organs for TCE-induced toxicity, consistent with the organ weight effects observed in the present study. We observed statistically significant increases in relative liver and kidney weights (13.1% and 17.4%, respectively) relative to controls, however we did not evaluate the histology of these organs to assess if there were histopathological correlates for these changes. Previously conducted studies have indicated that inhalation of TCE for acute or intermediate periods can cause liver enlargement in laboratory animals and that this effect is reversible upon cessation of exposure and histopathological changes have been observed in some studies but not in others ( Citation Prendergast et al., Citation1967; Citation Kjellstrand et al., Citation1983; Citation Maltoni et al., Citation1988; Citation ATSDR, Citation1997b). Similar effects have been noted for the kidney after inhalation exposure where increased weights were observed after short-term exposures; however, this was in the absence of any histopathological changes ( Citation Prendergast et al., Citation1967; Citation Kimmerle and Eben, Citation1973; Citation Kjellstrand et al., Citation1983). Therefore, the increased liver and kidney weights observed in the present study are consistent with the previously reported results for TCE after inhalation exposure.
In the context of immunotoxicity potential, exposure to TCE did not result in an effect on spleen or thymus weights and histopathology in the present study. Examination of spleen and thymus weights in previously published studies did not indicate any consistent treatment-related effect in response to TCE ( Citation Prendergast et al., Citation1967; Citation Kjellstrand et al., Citation1983; Citation Berman et al., Citation1995). One study did report histopathological changes in the spleen following TCE vapor exposure at 500 ppm, while no changes were observed in thymus morphology at any concentration level ( Citation Kaneko et al., Citation2000). However, effects on the spleen in that study may be due to the mouse strain used (MRL-lpr/lpr), which is known to develop spontaneous autoimmune disease that can affect lymphoid tissues from 6 weeks-of-age ( Citation Murphy, Citation1981; Citation Kanno et al., Citation1992; Citation Ratkay et al., Citation1994). Another study reported significant decreases in thymus weight in B6C3F1 mice after 30 weeks of exposure to 1.4 and 14 ppm TCE in drinking water ( Citation Keil et al., Citation2009); however, this effect was not observed in a developmental immunotoxicology study with TCE in B6C3F1 mice conducted in the same lab at the same concentrations ( Citation Peden-Adams et al., Citation2006). In general, effects on the thymus have not been observed in previous studies with rats and mice after acute, intermediate, or chronic oral administrations ( Citation ATSDR, Citation1997b). Overall, the lack of effects on spleen and thymus weights and histopathology in the present study are consistent with the previously reported results for TCE.
The present study also evaluated the immunotoxic potential of TCE through the evaluation of the antibody response to SRBC. Numerous non-guideline studies have reported variable immunotoxicity potential for TCE using laboratory animals; however, a definitive conclusion on the immunotoxic potential of TCE could not be drawn from the available information ( Citation ATSDR, Citation1997b). The results of the present study indicate that TCE suppressed the AFC/spleen and AFC/106 spleen cells responses in female Sprague Dawley rats by ≈ 70% at an exposure concentration of 1000 ppm, while no effect was noted at 100 and 300 ppm. These decreased AFC results are consistent with that observed in CD-1 female mice that demonstrated a statistically significant 50% suppression in antibody responses to SRBC following a 4-month exposure to 2.5 and 5 mg/ml TCE in drinking water, while no effect was noted at 0.1 and 1.0 mg/ml ( Citation Sanders et al., Citation1982). Based upon an average body weight near 30 g, an approximate drinking volume of 5 ml/day (CRL, 1999; Citation Suckow et al., Citation2001), and an estimated uptake of 90% after oral exposure ( Citation Lash et al., Citation2000), mice in the 2.5 and 5 mg/ml groups would have received ≈ 375 and 750 mg/kg/d of TCE, respectively.
For the present study, taking into account the relative minute volume for rats, the 6-h inhalation exposure period, and an estimated systemic uptake of ~ 70% of the inhaled concentration ( Citation Dallas et al., Citation1991), the estimated doses are ~ 106, 317, and 1056 mg/kg/day for TCE exposure levels of 100, 300, and 1000 ppm, respectively (see Supplementary Table 1 for concentration-to-dose calculations). Therefore, these studies both indicate that TCE is able to cause immune suppression at high exposure concentrations. The NOELs for immunotoxicity reported in this study (300 ppm, or ~ 317 mg/kg) and by Citation Sanders et al. (Citation1982) (~ 150 mg/kg) are considerably higher than the doses reported by Citation Peden-Adams et al. (Citation2006) to elicit immune suppression in mice following only 3 or 8 weeks of TCE administration via drinking water (1–10 ppm) as part of a developmental immunotoxicity investigation. However, the study design, conduct, and age of mice in the Peden-Adams et al. study differed significantly from the present study, such that a meaningful comparison of dose and effect levels cannot be made. Several factors including parental toxicity, coincident toxicity, control of litter-effects, and positive control data were not included in the reporting of the results and make the assessment and comparison of immunotoxic potential difficult.
As with TCE, the liver is also a well-documented target organ for PERC. In the present study slight increases in liver weight were noted after exposure to PERC at concentrations of 300 and 1000 ppm, which were coincident with very slight hypertrophy of centrilobular hepatocytes of the liver in four of eight rats exposed to 300 ppm, and in seven of eight rats exposed to 1000 ppm. In a previously published study, inhalation exposure to PERC resulted in dose-related liver congestion in rats, with 8/20, 10/20, and 15/19 rats affected at 400, 800, and 1600 ppm, respectively, with no liver effects observed at 200 ppm (NTP, 1986). In another inhalation study, mice and rats were exposed to 200 ppm of PERC for 28 days, or 400 ppm for 14, 21, or 28 days; centrilobular hepatocellular vacuolization was induced in mice and rats developed centrilobular hepatocellular hypertrophy ( Citation Odum et al., Citation1988). Although the kidney has also been reported as a target organ for PERC, effects are typically noted at concentrations higher than those that induce liver effects after intermediate exposure durations ( Citation Berman et al., Citation1995; ATSDR, 1997a). Therefore, the results of this study are consistent with that of previously published studies with PERC.
Similar to TCE, we did not observe any effects on the spleen and thymus after PERC exposure. In previously published studies, decreased spleen and thymus weights were observed in rats treated by gavage with PERC at 2000 mg/kg/day for 5 days; however, this effect was not observed at 1000 mg/kg/day ( Citation CitationHanioka et al., 1995). Histopathological changes in the spleen and thymus were not observed in female rats treated by gavage at 1500 mg/kg/day for 14 days ( Citation Berman et al., Citation1995). In addition, in a chronic gavage study with mice and rats, there were no histopathological changes in the spleen, lymph nodes, and thymus at doses that resulted in increased mortality (NCI, 1977). Collectively, these data indicate that the spleen and thymus are not target organs for PERC.
The present study also evaluated the immunotoxic potential of PERC through the evaluation of the antibody response to SRBC. The results indicate that PERC did not demonstrate evidence of immunotoxicity as it did not suppress the antibody response to SRBC at any of the examined concentrations. As part of this study we also evaluated the effect of PERC on the ability of pulmonary alveolar macrophage to phagocytize fluorescent microspheres, as previous studies have suggested potential alterations in macrophage activity after exposure to PERC. One study reported that mice exposed for 3 h to a 50 ppm PERC atmosphere showed decreased pulmonary bactericidal activity against Klebsiella pneumoniae ( Citation Aranyi et al., Citation1986); however, the significance of this result is uncertain due to the variability in the responses observed in control animals and the overall relevance of this response to humans (ATSDR, 1997a; ECETOC, 1999). Immunological monitoring of dry-cleaning workers has also suggested potential effects on the response of the respiratory immune system, such as alveolar macrophage activity ( Citation Andrys et al., Citation1997). Our results are not consistent with these previous reports, as the data did not indicate any inhibition of macrophage phagocytic activity after repeated exposure to high concentrations of PERC.
In conclusion, the results of these studies indicate that TCE had immunotoxic potential only at high exposure concentrations (1000 ppm), while PERC, at similar exposure concentrations, did not display any evidence of immunotoxicity. Reasons for the difference in the immune response to TCE and PERC are unknown, but may be related to differences in metabolism and subsequent metabolites that may be mediating the actual immunosuppressive response. While the metabolism of TCE and PERC is qualitatively similar, it has been well-established that the metabolism of PERC is quantitatively less than that of TCE ( Citation Chiu et al., Citation2007) and significant differences are known to exist in the rates of metabolism, metabolite intermediates, and the chemical reactivity of certain analogous metabolites ( Citation Lash et al., Citation2007). For example, although both substances are substrates for cytochrome P450 2E1, TCE is a far better substrate and therefore the overall metabolism and kinetics for formation of metabolites differ significantly from that of PERC ( Citation Lash et al., Citation2007). Therefore, the differences in metabolism between TCE and PERC may be one factor contributing to their differing effects on the antibody response to SRBC.
Supplementary Material
Download MS Excel (12.5 KB)Acknowledgements
The studies and manuscript preparation were funded by the Halogenated Solvents Industry Alliance, Inc., Arlington, VA.
Declaration of interest
All authors of this manuscript are employees of The Dow Chemical Company.
References
- Andrys, C., Hanovcova, I., Chylkova, V., Tejral, J., Eminger, S., Prochazkova, J. 1997. Immunological monitoring of dry-cleaning shop workers--exposure to tetrachloroethylene. Cent. Eur. J. Publ. Health 5:136–142.
- Aranyi, C., O’Shea, W. J., Graham, J. A., Miller, F. J. 1986. Effects of inhalation of organic chemical air contaminants on murine lung host defenses. Fundam. Appl. Toxicol. 6:713–720.
- ATSDR (Agency for Toxic Substances and Disease Registry). 1997a. Toxicological Profile for Tetrachloroethylene (PERC). Atlanta, GA: U.S. Department of Health and Human Services.
- ATSDR. 1997b. Toxicological Profile for Trichloroethylene (TCE). Atlanta, GA: U.S. Department of Health and Human Services.
- Berman, E., Schlicht, M., Moser, V. C., MacPhail, R. C. 1995. A multidisciplinary approach to toxicological screening: I. Systemic toxicity. J. Toxicol. Environ. Health 45:127–143.
- Chiu, W. A., Micallef, S., Monster, A. C., Bois, F. Y. 2007. Toxicokinetics of inhaled trichloroethylene and tetrachloroethylene in humans at 1 ppm: Empirical results and comparisons with previous studies. Toxicol. Sci. 95:23–36.
- CRL (Charles River Laboratories). 1999. Charles River Laboratories Technical Bulletin No. 1 1999. Availale onlie at: www.criver.com.
- Dallas, C. E., Gallo, J. M., Ramanathan, R., Muralidhara, S., Bruckner, J. V. 1991. Physiological pharmacokinetic modeling of inhaled trichloroethylene in rats. Toxicol. Appl. Pharmacol. 110:303–314.
- ECETOC (European Centre for Ecotoxicology and Toxicology of Chemicals). 1999. Joint Assessment of Commodity Chemicals No. 39: Tetrachloroethylene (CAS #127-18-4). Brussels: European Centre for Ecotoxicology and Toxicology of Chemicals.
- Germolec, D. R., Yang, R. S., Ackermann, M. F., Rosenthal, G. J., Boorman, G. A., Blair, P., Luster, M. I. 1989. Toxicology studies of a chemical mixture of 25 groundwater contaminants. II. Immunosuppression in B6C3F1 mice. Fundam. Appl. Toxicol. 13:377–387.
- Hanioka, N., Jinno, H., Toyo’oka, T., Nishimura, T., and Ando, M. (1995). Induction of rat liver drug-metabolizing enzymes by tetrachloroethylene. Arch Environ Contam Toxicol. 28, 273–280.
- Kaneko, T., Saegusa, M., Tasaka, K., Sato, A. 2000. Immunotoxicity of trichloroethylene: A study with MRL-lpr/lpr mice. J. Appl. Toxicol. 20:471–475.
- Kanno, H., Nose, M., Itoh, J., Taniguchi, Y., Kyogoku, M. 1992. Spontaneous development of pancreatitis in the MRL/Mp strain of mice in autoimmune mechanism. Clin. Exp. Immunol. 89:68–73.
- Keil, D. E., Peden-Adams, M. M., Wallace, S., Ruiz, P., Gilkeson, G. S. 2009. Assessment of trichloroethylene (TCE) exposure in murine strains genetically-prone and non-prone to develop autoimmune disease. J. Environ. Sci. Health 44:443–453.
- Kimmerle, G., Eben, A. 1973. Metabolism, excretion and toxicology of trichloroethylene after inhalation. 1. Experimental exposure on rats. Arch. Toxicol. 30:115–126.
- Kjellstrand, P., Holmquist, B., Alm, P., Kanje, M., Romare, S., Jonsson, I., Mansson, L., Bjerkemo, M. 1983. Trichloroethylene: Further studies of effects on body and organ weights and plasma butyrylcholinesterase activity in mice. Acta Pharmacol. Toxicol. (Copenhagen) 53:375–384.
- Lash, L. H., Fisher, J. W., Lipscomb, J. C., Parker, J. C. 2000. Metabolism of trichloroethylene. Environ. Health Perspect. 108 (Suppl 2):177–200.
- Lash, L. H., Putt, D. A., Huang, P., Hueni, S. E., Parker, J. C. 2007. Modulation of hepatic and renal metabolism and toxicity of trichloroethylene and perchloroethylene by alterations in status of cytochrome P450 and glutathione. Toxicology 235:11–26.
- Maltoni, C., Lefemine, G., Cotti, G., Perino, G. 1988. Long-term carcinogenicity bioassays on trichloroethylene administered by inhalation to Sprague-Dawley rats and Swiss and B6C3F1 mice. Ann. NY Acad. Sci. 534:316–342.
- Mann, C. M., Peachee, V. L., Trimmer, G. W., Lee, J. E., Twerdok, L. E., White, K. L. Jr. 2008. Immunotoxicity evaluation of jet a jet fuel in female rats after 28-day dermal exposure. J. Toxicol. Environ. Health 71:495–504.
- Murphy, E. D. 1981. Lymphoproliferation (lpr) and other single-locus models for murine lupus. In: Immunologic defects in laboratory animals. Vol. I (Gershwin, M. E.Merchant, B., Eds.). New York: Plenum Press, pp. 143–173.
- NCI (National Cancer Institute). 1977. Bioassay of tetrachloroethylene for possible carcinogenicity. Washington, DC: U.S. Department of Health, Education, and Welfare, Public Health Service, National Institutes of Health, DHEW Publ (NIH) 77–813.
- NTP (National Toxicology Program). 1986. Toxicology and carcinogenesis studies of tetrachloroethylene (perchloroethylene) (CAS No. 127-18-4) in F344/N rats and B6C3F1 mice (inhalation studies). US National Toxicology Program technical report series no. TR 311. National Toxicology Program, Research Triangle Park, NC.
- NTP. 1992a. Dose range-finding study for immunological evaluation of trichloroethylene (corn oil) in female B6C3F1 mice. National Toxicology Program Report of Task II-1-RF-TCE-14-1-PO. National Toxicology Program, Research Triangle Park, NC.
- NTP. 1992b. Dose range-finding study for immunological evaluation of trichloroethylene in 10% Emulphor in female B6C3F1 mice. National Toxicology Program Report of Task II-2-RF-TCE-14-2-PO. National Toxicology Program, Research Triangle Park, NC.
- NTP. 1992c. Dose range-finding study for immunological evaluation of trichloroethylene in 10% Emulphor in male CD-1 mice. National Toxicology Program Report of Task II-2-RF-TCE-14-4-PO. National Toxicology Program, Research Triangle Park, NC.
- Odum, J., Green, T., Foster, J. R., Hext, P. M. 1988. The role of trichloracetic acid and peroxisome proliferation in the differences in carcinogenicity of perchloroethylene in the mouse and rat. Toxicol. Appl. Pharmacol. 92:103–112.
- Peden-Adams, M. M., Eudaly, J. G., Heesemann, L. M., Smythe, J., Miller, J., Gilkeson, G. S., Keil, D. E. 2006. Developmental immunotoxicity of trichloroethylene (TCE): Studies in B6C3F1 mice. J. Environ. Sci. Health 41:249–271.
- Prendergast, J. A., Jones, R. A., Jenkins, L. J. Jr.Siegel, J. 1967. Effects on experimental animals of long-term inhalation of trichloroethylene, carbon tetrachloride, 1,1,1-trichloroethane, dichlorodifluoromethane, and 1,1-dichloroethylene. Toxicol. Appl. Pharmacol. 10:270–289.
- Ratkay, L. G., Tait, B., Tonzetich, J., Waterfield, J. D. 1994. Lpr and MRL background gene involvement in the control of adjuvant enhanced arthritis in MRL-lpr mice. J. Autoimmunity 7:561–573.
- Sanders, V. M., Tucker, A. N., White, K. L. Jr., Kauffmann, B. M., Hallett, P., Carchman, R. A., Borzelleca, J. F., Munson, A. E. 1982. Humoral and cell-mediated immune status in mice exposed to trichloroethylene in the drinking water. Toxicol. Appl. Pharmacol. 62:358–368.
- Schlichting, L. M., Wright, P. F., Stacey, N. H. 1992. Effects of tetrachloroethylene on hepatic and splenic lymphocytotoxic activities in rodents. Toxicol. Ind. Health 8:255–266.
- Suckow, M. A., Danneman, P., Brayton, C., (Eds.). 2001. The Laboratory Mouse. New York: CRC Press.
- White, K. L. Jr., Germolec, D. R., Booker, C. D., Hernendez, D. M., McCay, J. A., Delclos, K. B., Newbold, R. R., Weis, C., Guo, T. L. 2005. Dietary methoxychlor exposure modulates splenic natural killer cell activity, antibody-forming cell response and phenotypic marker expression in F0 and F1 generations of Sprague Dawley rats. Toxicology 207:271–281.
- WHO (World Health Organization). 2006. Concise International Chemical Assessment Document 68: Tetrachloroethene. Geneva: World Health Organization.