Abstract
Exposure to loud noise levels represents a problem in all regions of the world. Noise exposure is known to affect auditory structures in living organisms. However, it should not be ignored that many of the effects of noise are extra-auditory. In particular, it has been proposed that noise could affect immune system similarly to other stressors. Nevertheless, only a few studies so far have investigated the effects of noise on the immune function. The aim of the present work was to investigate the effect of chronic (2 weeks) noise (95–97 dBA) exposure on immune responses in BALB/c and C57 mice. To ascertain if the effect of noise is similar to other psychological stressors, the effect of chronic restraint—applied for the same time—on immune response was also analyzed. It was found that chronic noise impaired immune-related end-points in vivo and ex vivo depending on the strain used. Noise, but not restraint, affected C57Bl/6 mouse T-cell-dependent antibody production and ex vivo stimulated T-cell proliferation, but had no effect on these parameters in BALB/c mice or their cells. In fact, none of the stressors altered T-cell responses associated with the BALB/c mice. Further, noise exposure induced a decrease in corticosterone and catecholamines levels in BALB/c mice. In contrast, no differences were seen in these parameters for those BALB/c mice under restraint or for that matter C57Bl/6 mice exposed to restraint or noise. The results of these studies indicate that noise could seriously affect immune responses in susceptible individuals. In addition, it may also be concluded that noise possibility should not be considered a classic stressor.
Introduction
During daily life, people are exposed to potentially hazardous noise levels coming from work environment, urban traffic, household appliances, or discotheques. High levels of occupational noise has become a problem in all regions of the world. In addition, it has been proposed that noise might be responsible for several disorders that affect not only the auditory organs, but also structures belonging to the nervous, endocrine, and cardiovascular systems (Lenzi et al., Citation2003; Uran et al., Citation2012). Therefore, although the auditory effects of noise have been long studied, they do not appear to be the only factors responsible for many of the effects of noise on living organisms. In fact, noise-associated disorders are mainly extra-auditory (Lenzi et al., Citation2003; Prasher, Citation2009; Rabat, Citation2007; Turner et al., Citation2005; Uran et al., Citation2010, Citation2012), and become evident after exposure to noise levels as low as 50 dBA. In particular, it has been suggested that loud noise can induce a variety of symptoms, including changes in anxiety, emotional stress, an increase in social conflicts, as well as general psychiatric disorders (Rabat, Citation2007).
Similarly, animal experiments demonstrated that acute and chronic noise exposures can also induce temporary or permanent changes related to the central nervous system (CNS) (Manikandan et al., Citation2006; Uran et al., Citation2010, Citation2012). However, despite its pivotal importance for the health of individuals, only a few studies so far have investigated the effects of noise on the immune function. In general, these effects are associated with inflammation or immune activity after noise exposure as a putative cause for adult onset hearing loss (HL). In fact, it was described that noise exposure can activate a cochlear immune response which, in the presence of antigen (Ag), allows for greater recruitment of inflammatory cells than occurred in response to Ag alone (Miyao et al., Citation2008).
Regarding the stress response, it was proposed that there are two groups of people, those who are ‘high reactors’, and those who are ‘low reactors’. High reactors are significantly affected by stress, as shown by a significant increase in heart rate, blood pressure, catecholamines, and CD8+ cells as well as a significant decrease in proliferative responses to mitogens like phytohemagglutinin (PHA) and concanavalin A (ConA). Low reactors show little or no change in these parameters (Marsland et al., Citation2002). In addition, it has been observed that two genetically different inbred strains of mice, C57Bl/6 (C57) and BALB/c, differ one from another in several behavioral and immune responses after stress exposure. The BALB/c is considered an emotive anxious strain with increased stress reactivity, whereas C57 is considered non-emotive, non-anxious, and possesses lower stress reactivity (Ducottet & Belzung, Citation2004). In this regard, we have previously demonstrated that stressed BALB/c, but not C57, mice showed a poor learning performance and a decreased T-cell reactivity after 6 weeks of exposure to chronic mild stress (Palumbo et al., Citation2010).
Based on the above, the aim of the present work was to investigate the effect of chronic noise exposure on immune response in BALB/c and C57 mice. To ascertain if the effect of noise is similar to other psychological stressors, the effect of chronic restraint on immune response was investigated. Finally, the adaptive response to stressors involves the activation of the hypothalamic–pituitary–adrenal (HPA) axis and the sympathetic nervous system (SNS) that, in turn, can modulate immune reactivity (Elenkov et al., Citation2000; Roszman & Brooks, Citation1997). Therefore, the participation of catecholamines and corticosterone in the effect of noise exposure and restraint stress on immunity was also analyzed.
Materials and methods
Animals
Inbred female BALB/c and C57Bl/6J (C57) mice were purchased from Veterinary School of the University of Buenos Aires. Sixty-day-old mice weighing 23–25 g at the beginning of the experiments were used. Mice were housed and maintained on an 8:00 AM to 8:00 PM light/dark cycle under controlled temperatures (18–22 °C) and relative humidity (60 [±10]%), and had ad libitum access to standard rodent chow and filtered water. Two weeks before the beginning of the experiments, phases of the estrous cycle were monitored daily in order to verify that all mice have a synchronized estrous cycle.
All animals were handled and sacrificed according to the Institutional Committee for the Use and Care of Laboratory Animal rules (CICUAL, School of Medicine, University of Buenos Aires, Argentina); the protocol was approved by this Committee under resolution 2079/07. The CICUAL adheres to the rules of the ‘Guide for the Care and Use of Laboratory Animals’ (NIH) and the institution has an Animal Welfare Assurance approved by the Public Health Service (PHS) with the assurance number A5801-01. Adequate measures were taken to minimize animal pain or discomfort.
Chronic noise exposure (NS)
To obtain white noise, computer software (TrueRTA) was chosen, using a bandwidth from 20 Hz to 20,000 Hz in octave bands. For sound amplification, an active 2-way monitor (SKP, SK150A, 40 W RMS per channel; Behringer, Willich, Germany) located at 30 cm from the animal cage in a sound chamber of 1 m × 1 m × 1 m was used. Noise intensity was measured using an omnidirectional measurement condenser microphone (Behringer ECM 8000) each day prior to animal exposure, by placing the microphone in the acoustic chamber at several locations, and taking an average of the different readings. Animals were exposed to white noise at 95–97 dBA (20–20 000 Hz) 1 h/day, for 14 days (NS). Background noise level was 55 dBA. Lighting was provided by a 20 W lamp located in the upper left corner of the sound chamber. After the final exposure, the mice were left undisturbed for 1 day before either being euthanized (by CO2 asphyxiation) or undergoing immunization.
Chronic restraint exposure (RS)
Restraint was performed by placing each animal in a well-ventilated polypropylene tube (2.8 cm diameter × 11.5 cm length) for 2 h starting at 10.00 AM. Animals were not physically compressed. This procedure was repeated for 2 weeks and then the mice were left undisturbed for 1 day before euthanization (by CO2 asphyxiation) or immunization.
Antibody production
Sheep red blood cells (SRBC) were obtained from the Laboratorio Alfredo Gutierrez (Buenos Aires, Argentina) for use as the immunogen to evaluate T-cell-dependent humoral responses. In brief, mice were intraperitoneally (IP) immunized on Day 0 and boosted on Day 11 with 0.2 ml of a 4% SBRC solution in saline. Samples of retro-orbital blood were collected for antibody determination on Day 18 (secondary response). Mice injected with the vehicles were used as controls. A quantitative enzyme-linked immunoadsorbent assay (ELISA) was performed to determine SRBC-specific antibodies in serum as previously described (Silberman et al., Citation2003). For this, 96-well plates (Maxisorp Nunc immunoplates, Thermo Fisher Scientific, Langensel-bold, Germany) were coated overnight with SRBC membranes (10 μg/ml). Serial dilutions of sera were then added and the plate incubated for 2 h at room temperature. After this, the plates were washed, and samples incubated with a goat IgG anti-mouse IgM or IgG-phosphatase alkaline conjugate (Sigma, St. Louis, MO) for 2 h at room temperature. After a final washing to remove non-adherent secondary antibody, 100 μl p-nitrophenylphosphate solution (Sigma; 1 mg/ml in 0.1 M sodium carbonate, pH 11) was added to each well as a substrate and left to develop coloration that was ultimately read at 405 nm in a Bio-Rad plate-reader (Hércules, CA). Reactions were considered positive when optical density (OD) values were above the mean value + 2 SD of the normal sera (from non-immunized and/or vehicle injected mice that gave non-statistical differences among them) value. The antibody titer for each serum was defined as the last serum dilution with positive reaction.
Mitogen assay
Due to the well-known lymphoid tissue profiles, cell suspensions were prepared from lymph nodes for use in evaluating T-cell proliferative responses. Lymph node (inguinal, axial and mesenteric) from control and stress exposed mice were obtained and cell suspensions were prepared as previously described (Silberman et al., 2003). T-cell proliferation was determined by culturing 3 × 105 cells/well in 96-well plates in 100 μl (triplicate) aliquots in supplemented medium (RPMI 1640 containing 10% batched-tested non-stimulatory fetal calf serum [Internegocios S.A., Buenos Aires, Argentina], 50 μM β-mercaptoethanol [Sigma], and 2 mM glutamine, 100 U penicillin/ml, and 100 µg streptomycin/ml [each Gibco, Grand Island, NY]). Aliquots of 100 μl of Concanavalin A (ConA; Sigma) were then added to the micro-cultures to yield the optimal mitogen concentration (2 μg/ml) based on previous dose–response curve determinations in pilot studies. In control cultures, stimulants were replaced by 100 μl of culture medium. All cells were then cultured at 37 °C in a 5% CO2 atmosphere for 3 days. Mitogenic activity was ultimately measured by adding 0.75 μCi [3H]-thymidine (Perkin-Elmer, Boston, MA) per well for the last 24 h period of culture and the cells then being harvested and analyzed for incorporated radioactivity in a TriCarb 2800 TR liquid scintillation counter (Perkin-Elmer, Waltham, MA).
Catecholamines assay
Catecholamine concentrations were determined in spleen samples from the mice using the fluorometric assay of Laverty and Taylor (Citation1968). Briefly, spleens removed at necropsy were homogenized in a solution of 12.5% sodium sulfite/10% EDTA (in 0.4 N percloric acid); after 24 h at 4 °C, the material was centrifuged at 5000 rpm for 10 min. Supernatants were recovered and adjusted to pH 8.2 using 0.5 M Tris-HCl/EDTA (pH 9). The material was then transferred to atop a pre-washed alumina column (Sigma). Eluates were recovered using a 0.2 N acetic acid eluent and then oxidized with iodine in an alkaline medium. The fluorescence of the material was recorded at 480 nm in a Jasco FP 770 spectrofluorometer (Tokyo, Japan) using an excitation source of 380 nm. The level of catecholamine present in the sample was then calculated by extrapolation from a reference curve prepared in parallel using noradrenaline as a standard.
Corticosterone determination
To avoid fluctuations on plasma corticosterone levels due to circadian rhythms, animals were bled at 12:00 AM on the day of sacrifice. Corticosterone levels were determined using a standard radioimmunoassay (Etches, Citation1976). The cross-reactivity of the corsticosterone antibody (Sigma) with other relevant steroids was 4.4% (cortisol), 30% (deoxycorticosterone) and 5.5% (testosterone) as determined in pilot analyses. Sensitivity of the corticosterone radioimmuno-assay was 5 ng/ml.
Statistical analysis
Data were analyzed using a one-way analysis of variance (ANOVA) and a Bonferroni’s (t) post-hoc test to examine significance differences between groups. Antibody production data were not normally distributed, so a non-parametric statistic Kruskal–Wallis test was performed. Differences between means in all analyses were considered significant at p ≤ 0.05.
Results
Noise alters IgG antibody production after T-dependent antigen immunization in C57 mice
To investigate whether noise and restraint stress exposure was associated with changes in antibody production, we examined the humoral response to SRBC—a thymus-dependent antigen—in non-exposed and in noise- and restraint-exposed BALB/c and C57 mice. As can be seen in , noise significantly affected the IgG production in C57 mice () but not in BALB/c mice (). Moreover, restraint exposure did not affect the anti-SRBC IgG titers neither C57 () nor BALB/c () (KW (8), C57, H = 6.02, p = 0.044; noise p < 0.05, restraint NS; BALB/c H = 3.60, NS).
Figure 1. Antibody titers after SRBC immunization of normal and stressed C57 and BALB/c mice. Control non-exposed (n = 8, ○) or stress-exposed (noise [NS] n = 8, •; restraint [RS] n = 8, •) C57 mice (a and c) and control non-exposed (n = 8, ○) or stress-exposed (noise n = 8, •, restraint n = 8, •). BALB/c mice (b and d were immunized with SRBC or 4% saline only. Serum was collected on Day 18 and assayed for a presence of IgG by ELISA. Curves shown in (a) and (b) are representative of four assays performed in duplicate. Each experiment was performed with two sera of each experimental condition. Straight line represents OD values + 2 SD of normal sera. Bars in (c) and (d) shows the antibody titer obtained for animals under the different conditions (n = 8 each one). *p < 0.05 with respect to the corresponding control mice.
![Figure 1. Antibody titers after SRBC immunization of normal and stressed C57 and BALB/c mice. Control non-exposed (n = 8, ○) or stress-exposed (noise [NS] n = 8, •; restraint [RS] n = 8, •) C57 mice (a and c) and control non-exposed (n = 8, ○) or stress-exposed (noise n = 8, •, restraint n = 8, •). BALB/c mice (b and d were immunized with SRBC or 4% saline only. Serum was collected on Day 18 and assayed for a presence of IgG by ELISA. Curves shown in (a) and (b) are representative of four assays performed in duplicate. Each experiment was performed with two sera of each experimental condition. Straight line represents OD values + 2 SD of normal sera. Bars in (c) and (d) shows the antibody titer obtained for animals under the different conditions (n = 8 each one). *p < 0.05 with respect to the corresponding control mice.](/cms/asset/76ecd6f1-6d0e-4bb9-90a6-3a03edfce667/iimt_a_800171_f0001_b.jpg)
Noise affects T-cell reactivity of lymphocyte from C57 mice
To analyze the effect of stress exposure on T-cell reactivity, the mitogen-induced proliferative response of T-cells was analyzed. Results showed that mitogen-induced proliferation was significantly decreased among lymphocytes from C57 mice exposed to noise () but not among those cells from hosts exposed to restraint (ANOVA, F(2, 28) = 7.88, p = 0.0019; noise p < 0.01, restraint NS). On the other hand, neither restraint nor noise exposure had an effect on lymphocyte reactivity of cells recovered from BALB/c mice (, F(2, 26) = 2.21 NS).
Figure 2. Cellular proliferation assays. Mitogen-induced proliferation of T-lymphocytes was determined by [3H]-thymidine uptake by cells from control (white bars, n = 11) or noise- (NS; grey bars, n = 11) or restraint-stressed (RS; black bars, n = 9) C57 (a, crossed bars) and BALB/c (b, plain bars; n = 10, 10, and 9 for control, NS, and RS mice, respectively) mice. Results shown correspond to the maximal 3H-thymidine uptake (Day 3 of culture) and represent the mean (±SE) of five independent assays. **p < 0.01 versus corresponding control. [3H]-thymidine uptake (dpm) for non-stimulated cells from C57 hosts: control = 2238 ± 429, noise = 2551 ± 380, and restraint = 2795 ± 499. For BALB/c hosts, these values were: control = 2515 ± 542, noise = 3051 ± 546, and restraint = 2973 ± 486.
![Figure 2. Cellular proliferation assays. Mitogen-induced proliferation of T-lymphocytes was determined by [3H]-thymidine uptake by cells from control (white bars, n = 11) or noise- (NS; grey bars, n = 11) or restraint-stressed (RS; black bars, n = 9) C57 (a, crossed bars) and BALB/c (b, plain bars; n = 10, 10, and 9 for control, NS, and RS mice, respectively) mice. Results shown correspond to the maximal 3H-thymidine uptake (Day 3 of culture) and represent the mean (±SE) of five independent assays. **p < 0.01 versus corresponding control. [3H]-thymidine uptake (dpm) for non-stimulated cells from C57 hosts: control = 2238 ± 429, noise = 2551 ± 380, and restraint = 2795 ± 499. For BALB/c hosts, these values were: control = 2515 ± 542, noise = 3051 ± 546, and restraint = 2973 ± 486.](/cms/asset/afd15260-dfe5-4b19-9fc4-d330253ef093/iimt_a_800171_f0002_b.jpg)
Noise decreases corticosterone and catecholamines levels in BALB/c but not in C57 mice
To investigate the participation of HPA and SNS activation in any disruption of T-cell responses after noise exposure, corticosterone concentrations in plasma and catecholamine levels in the spleen that receives important sympathetic innervation (Hori et al., Citation1995; Kim et al., Citation2004) were determined. Data in show that noise exposure decreased corticosterone levels in BALB/c mice () but did not affect those of C57 (). In addition, animals submitted to restraint did not show differences in corticosterone levels with respect to control mice () (ANOVA, C57, F(2, 23) = 0.32, NS; BALB/c, F(2, 24) = 5.72, p = 0.0093, noise, p < 0.05, restraint, NS). Similar results were found for splenic catecholamine content () (ANOVA, C57, F(2, 23) = 0.54, NS; BALB/c, F(2, 23) = 6.26, p = 0.0068, noise, p < 0.05, restraint, NS).
Figure 3. Serum corticosterone levels and catecholamine concentrations. (a) and (b) show the mean (±SE) corticosterone levels for control (white bars, n = 9) and in noise- (NS; grey bars, n = 9) and restraint-stressed mice (RS; black bars, n = 8) C57 (a, crossed bars) and for BALB/c hosts (b, plain bars; n = 9 for each group). (c) and (d) show the mean (±SE) catecholamine levels for control (white bars, n = 9) and noise- (grey bars, n = 9) and restraint-stressed mice (black bars, n = 8) C57 (crossed bars) and for BALB/c hosts (plain bars n = 9, 9, and 8 for control, NS, and RS mice, respectively). *p < 0.05.
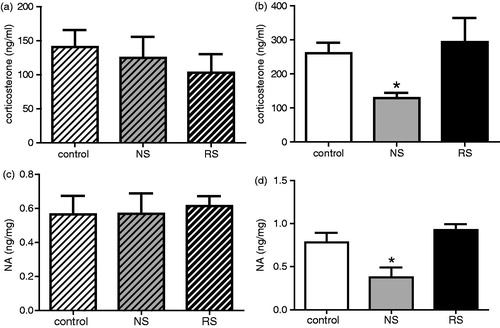
Discussion
Noise has been considered a common non-specific stressor. In addition, it was proposed that the physiological response to noise as a stressor is not different from any other non-specific stressor (Waye et al., 2002; Prasher, 2009). Most researchers would probably accept a classification of hemorrhage and immune challenge as physical stressors, and noise and restraint as psychological stressors (Dayas et al., Citation2001). However, here it was shown that noise and restraint exposure during a 2-week period induced different effects on immune cells depending on the mouse strain used. Chronic noise exposure but not restraint affected the response of T-cells from treated C57 mice. None of the stressors altered the responses of T-cells from BALB/c mice. However, we previously found that BALB/c were more sensitive to chronic mild stress (CMS) exposure than C57 mice, as 6 weeks of CMS exposure induced a decreased T-cell response in BALB/c but not C57 mice (Palumbo et al., Citation2010). In addition, BALB/c hosts showed a decreased immune response after 3 weeks of 6 h-restraint exposure (Frick et al., Citation2009), but not C57 mice (unpublished observations). Moreover, Zheng & Ariizumi (Citation2007) showed that the exposure to 90 dBA white noise for 5 h/day for 3 days induced an increase in immune responses in BALB/c mice, but that a 4-week exposure suppressed it. Similarly, we found that BALB/c mice that underwent acute stress exhibited an increase in their immune response, but that a chronic (4 week) mild stress induced an inhibition of these responses (Silberman et al., Citation2003, Citation2004).
It seems likely that the duration/intensity of any stress is important when considering its impact on individuals (Dhabhar, Citation2008). In the present work, BALB/c mice were exposed to restraint and noise for 1 h/day for 2 weeks; based on the above-noted information, it might be suggested that this duration and intensity was not sufficient to induce changes in the immune responses in this particular strain. Interestingly, in contrast, C57Bl/6 mice showed an impaired immune response after the same noise exposure. It is important to note that C57BL/6 and BALB/c mice are homozygous for Ahl (Johnson et al., Citation2000) that imparts both vulnerability to noise and age-related hearing loss (Jimenez et al., Citation2001). In addition, it has also been reported that both the C57BL6 and BALB/c strains display genetically-determined progressive hearing losses that begin relatively early in life; however, the hearing loss progresses slowly (Turner et al., Citation2005). In addition, it was reported that noise intensities <100 dBA did not produce auditory trauma in these mice (Turner et al., Citation2005). Taking these observations into account, it is possible to conclude that, compared to the BALB/c, the C57BL/6 mice could be the more sensitive strain with respect to any immune/extra-auditory effects of noise.
It is known that the hypothalamic-pituitary-adrenal (HPA) axis and the autonomous nervous system (ANS) modulate the immune system (Elenkov et al., Citation2000; Roszman & Brooks, Citation1997). In general, it was considered that corticosterone and catecholamines levels were increased under a stress condition that, in turn, could induce a decrease in the immune response. However, it was found here that noise exposure induced a decrease in corticosterone and catecholamines levels in BALB/c mice. No differences were observed for BALB/c mice under restraint or for C57 mice exposed to restraint or noise. In addition, O’Connor et al. (Citation2011) demonstrated that low light intensity and continual high background noise (eight weeks) in hens have a detrimental effect on egg production in the early laying phase as well as influencing the time allocated to different behaviors. However, the authors did not find evidence for a physiological stress determined by corticosterone levels and heterophils to lymphocytes (H/L) ratio, under low light intensity or noise. Interestingly, Zheng & Ariizumi (Citation2007) found that both acute and chronic noise exposure induced an increase in corticosterone levels, but that, while acute exposure could enhance immune responses, chronic exposure suppressed it. Those authors also demonstrated that noise-induced reductions in immune function could be related not only to neuroendocrine changes but also to an induced imbalance associated with oxidative stress. As high levels of corticosterone have been associated to an increase in oxidative stress (Costantini et al., Citation2011), perhaps a decrease in corticosterone could represent a counterbalance mechanism that evolved to avoid any stress-induced impairment of the immune response. Clearly, further experiments are necessary to verify this possibility.
Given these results it is possible to postulate that under noise exposure corticosterone and catecholamines seem to play a role in the generation of immune alteration that is different from the classically described for stress experimental models. Thus, an immune response would not be affected when these hormones had decreased levels, as occurred after noise exposure in BALB/c mice, but rather would arise in the absence of changes in hormone levels, as was found in C57 mice. These findings indicate that noise could affect T-cell responses through a different mechanism than other stressors. In fact, we previously determined that noise might not work as a classical stressor, as noted in rats exposed to the same levels of noise (Uran et al., Citation2010). In addition, noise and negative effects—triggered by stress, anxiety, anger and depression—precipitated headaches. However, these two factors did not interact in triggering headaches and they provoke this discomfort by different mechanisms (Martin et al., Citation2005).
In conclusion, the present study suggests that noise affects seriously the immune response in susceptible individuals, probably by a different mechanism than other stressors. It is possible to postulate that noise could be a non-classical stressor. Further studies are necessary to elucidate the exact mechanism(s) by which noise affects the immune response.
Declaration of interest
The authors report no conflicts of interest. The authors alone are responsible for the content and writing of the paper.
Acknowledgements
We thank Daniel Gonzalez for his valuable help in the animal stress model and Noemí Capano and Patricia Fernandez for the secretarial assistance. This work was supported by grants from CONICET (PIP 00281) Argentina.
References
- Costantini, D., Marasco, V., and Møller, A. P. 2011. A meta-analysis of glucocorticoids as modulators of oxidative stress in vertebrates. Comp. Physiol. B 181:447–456
- Dayas, C. V., Buller, K. M., Crane, J. W., et al. 2001. Stressor categorization: acute physical and psychological stressors elicit distinctive recruitment patterns in the amygdale and in medullar noradrenergic cell groups. Eur. J. Neurosci. 14:1143–1152
- Dhabhar, F. S. 2008. Enhancing vs. suppressive effects of stress on immune function: implications for immunoprotection vs. immunopathology. Allergy Asthma Clin. Immunol. 4:2–11
- Ducottet, C., and Belzung, C. 2004. Behavior in the elevated plus-maze predicts coping after subchronic mild stress in mice. Physiol. Behav. 81:417–426
- Elenkov, I. J., Wilder, R. L., Chrousos, G. P., et al. 2000. The sympathetic nerve--an integrative interface between two supersystems: the brain and the immune system. Pharmacol. Rev. 52:595–638
- Etches, R. J. 1976. A radioimmunoassay for corticosterone and its application to the measurement of stress in poultry. Steroids 28:763–773
- Frick, L. R., Arcos, M. L., Rapanelli, M., et al. 2009. Chronic restraint stress impairs T-cell immunity and promotes tumor progression in mice. Stress 12:134–143
- Hori, T., Katafuchi, T., Take, S., et al. 1995. The autonomic nervous system as a communication channel between the brain and the immune system. Neuroimmunomodulation 2:203–215
- Jimenez, A. M., Stagner, B. B., Martin, G. K., and Lonsbury-Martin, B. L. 2001. Susceptibility of DPOAEs to sound over-exposure in inbred mice with AHL. Assoc. Res. Otolaryngol. 2:233–245
- Johnson, K. R., Zheng, Q. Y., and Erway, L. C. 2000. A major gene affecting age-related hearing loss is common to at least ten inbred strains of mice. Genomics 70:171–180
- Kim, D., Seegal, R. F., and Lawrence, D. A. 2004. Splenic norepinephrine and serum corticosterone level fluctuations associated with bacteria-induced stress. Neuroimmunomodulation 11:323–331
- Laverty, R., and Taylor, K. M. 1968. The fluorometric assay of catecholamines and related compounds: improvements and extensions to the hydroxyindole technique. Anal. Biochem. 22:269–279
- Lenzi, P., Frenzilli, G., Gesi, M., et al. 2003. DNA damage associated with ultrastructural alterations in rat myocardium after loud noise exposure. Environ. Health Perspect. 111:467–571
- Manikandan, S., Padmab, M. K., Srikumar, R., et al. 2006. Effects of chronic noise stress on spatial memory of rats in relation to neuronal dendritic alteration and free radical-imbalance in hippocampus and medial pre-frontal cortex. Neurosci. Lett. 399:17–22
- Marsland, A. L., Bachen, E. A., Cohen, S., et al. 2002. Stress, immune reactivity and susceptibility to infectious disease. Physiol. Behav. 77:711–716
- Martin, P. R., Todd, J., and Reece, J. 2005. Effects of noise and a stressor on head pain. Headache 45:1353–1364
- Miyao, M., Firestein, G. S., and Keithley, E. M. 2008. Acoustic trauma augments the cochlear immune response to antigen. Laryngoscope 118:1801–1808
- O'Connor, E. A., Parker, M. O., Davey, E. L., et al. 2011. Effect of low light and high noise on behavioral activity, physiological indicators of stress and production in laying hens. Br. Poult. Sci. 52:666–674
- Palumbo, M. L., Canzobre, M. C., Pascuan, C. G., et al. 2010. Stress-induced cognitive deficit is differentially modulated in BALB/c and C57Bl/6 mice. Correlation with TH1/TH2 balance after stress exposure. J. Neuroimmunol. 218:12–20
- Prasher, D. 2009. Is there evidence that environmental noise is immunotoxic? Noise Health 11:151–155
- Rabat, A. 2007. Extra-auditory effects of noise in laboratory animals: Relationship between noise and sleep. J. Am. Assoc. Lab. Anim. Sci. 46:35–41
- Roszman, T. L., and Brooks, W. H. 1997. Interactive signaling pathways of the neuroendocrine-immune network. Chem. Immunol. 69:203–222
- Silberman, D. M., Wald, M. R., and Genaro, A. M. 2003. Acute and chronic stress exert opposing effects on antibody responses associated with changes in stress hormone regulation of T-lymphocyte reactivity. J. Neuroimmunol. 144:53–60
- Silberman, D. M., Ayelli-Edgar, V., Zorrilla-Zubilete, M., et al. 2004. Impaired T-cell dependent humoral response and its relationship with T-lymphocyte sensitivity to stress hormones in a chronic mild stress model of depression. Brain Behav. Immun. 18:81–90
- Turner, J. G., Parrish, J. L., Hughes, L. F., et al. 2005. Hearing in laboratory animals: Strain differences and non-auditory effects of noise. Comp. Med. 55:12–23
- Uran, S. L., Aon-Bertolino, L., Caceres, L. G., et al. 2012. Rat hippocampal alterations could underlie behavioral abnormalities induced by exposure to moderate noise levels. Brain Res. 1471:1–12
- Uran, S. L., Caceres, L. G., and Guelman, L. R. 2010. Effects of loud noise on hippocampal and cerebellar-related behaviors. Role of oxidative state. Brain Res. 1361:102–114
- Waye, K. P., Bengtsson, J., Rylander, R., et al. 2002. Low frequency noise enhances cortisol among noise sensitive subjects during work performance. Life Sci. 70:745–758
- Zheng, K. C., and Ariizumi, M. 2007. Modulations of immune functions and oxidative status induced by noise stress. J. Occup. Health 49:32–38