Abstract
The T-cell-dependent antibody response (TDAR) is a functional assay used in immunopharmacology and immunotoxicology to assess ability to mount an antibody response to immunization. Keyhole limpet hemocyanin (KLH) is extensively used as the immunogen of choice in non-clinical and clinical settings. Native KLH is comprised of high molecular weight (HMW; 4–8 MDa) assemblies of KLH subunit dimers (> 600–800 kDa). It is not known how the different forms (HMW vs subunit) and manufacturing processes (commercial sources) may impact the nature of anti-KLH immune responses (e.g. magnitude and inter-animal variability). Anti-KLH IgM and IgG responses were studied in Sprague-Dawley rats immunized with different forms and commercial sources of KLH: 100 µg of HMW KLH from two different sources or subunit KLH from three different sources. Biophysical and biochemical analyses were conducted to characterize the KLH formulations. Anti-KLH IgM and IgG responses were measured using a proprietary indirect quantitative electrochemiluminescence immunoassay. The HMW KLH preparations showed a greater number of sub-visible particles (2–150 µm size range) than the subunit KLH preparations. All HMW KLH and all subunit KLH were equivalent on SEC (hydrodynamic volume), PAGE (size and charge), and SDS-PAGE (molecular radius). Robust primary and secondary anti-KLH responses were detected for both sources of HMW KLH. The subunit KLH immunizations resulted in lower IgG and IgM responses compared to the HMW KLH, with the exception of Stellar Biotechnologies subunit KLH that produced both robust primary and secondary responses, which approached the HMW KLH responses. Inter-animal variability for IgM and IgG responses was lower with HMW KLH than with subunit KLH. In conclusion, different forms and commercial sources of KLH were associated with different magnitudes and inter-animal variability in IgM and IgG responses, a critical finding to take into consideration when designing TDAR studies for robust immunotoxicology or immunopharmacology testing.
Introduction
The T-cell dependent antibody response (TDAR) is a functional assay used in immunopharmacology and immunotoxicology to assess the ability of a test system to mount an antibody response (IgM and/or IgG) to immunization. TDAR is widely recognized as a standard tool for monitoring the effects of drugs on the immune system. It is designated in regulatory guidance documents as one of the recommended assays to evaluate potential immunotoxicity of investigational new drugs (FDA, Citation2002; ICH, Citation2005).
Keyhole limpet hemocyanin (KLH) is extensively used in TDAR testing as the immunogen of choice in non-clinical and clinical settings. KLH is a very large, highly immunogenic, glycoprotein complex isolated from the hemolymph of the marine mollusk Megathura crenulata. KLH has been widely used for decades as a carrier protein for haptens, an adjuvant, and a stand-alone antigen for immune status assessment in immunotoxicology applications. Properties accounting for the widespread use of KLH include: (i) availability of clinical grade material and utilization in the clinical setting; (ii) large molecular size; (iii) solution stability; (iv) endogenous adjuvant activity (Herscowitz et al., Citation1972; Weigle, Citation1964); (v) processing via C-lectin receptor (CLR) pathways (Presicce et al., Citation2008); and (vii) up-regulated expression of T-helper (TH)-1 and TH2 cytokines and cross-presentation of antigen to induce both cell-mediated immunity (TH1) and antibody (TH2) responses (Kantele et al., Citation1999; Yefenof et al., Citation1990).
Native KLH is comprised of high molecular weight (HMW; 4–8 MDa) assemblies of KLH subunit dimers (> 600–800 kDa). Differences in immunogenicity between different preparations have been observed in the past. Herscowitz et al. (Citation1972) demonstrated that crude KLH was more immunogenic than purified KLH in rabbits. Miller et al. (Citation2005) demonstrated that a KLH isolated using a process preserving the high molecular weight structure of the molecule was more immunogenic than a purified immunocyanin containing KLH subunits in healthy volunteers. These studies suggest that immunotoxicology data may be difficult to interpret across laboratories and that reagent differences may be complicating the analysis of immune status results. It is not known how the different forms (HMW versus subunit) and manufacturing processes (commercial sources) may impact the nature of anti-KLH immune responses (e.g. magnitude and inter-animal variability). The purpose of the present study was to evaluate antibody response to different forms and sources of KLH. Our aim is to optimize the TDAR so as to improve standardization and support the translation of new pharmaceuticals from bench to clinic.
Anti-KLH IgM and IgG responses were studied in female Sprague-Dawley rats immunized on Days 1 and 22 with 100 µg of HMW KLH from two different commercial sources or subunit KLHs from three different commercial sources, without co-injection with any adjuvant. Biophysical and biochemical characterizations of the KLH formulations were conducted. Anti-KLH IgM and IgG responses were measured on Days 1, 8, 15, 22, 29, 36, and 43 using a proprietary quantitative indirect electrochemiluminescence (ECL) immunoassay.
Methods
Test system
Female Sprague-Dawley rats (≈10 weeks-of-age at first dose) were obtained from Charles River Laboratories (Wilmington, MA) and cared for in accordance to the Guide for the Care and Use of Laboratory Animals, 8th Edition (2011). Animals were pair-housed at an AAALAC, Intl-accredited facility in non-sterile ventilated microisolator housing on corncob bedding. All research protocols were approved by Amgen Inc. (Seattle, WA) Institutional Animal Care and Use Committee. Animals had ad libitum access to pelleted feed (2020X Teklad; Harlan Laboratories Inc., Madison, WI) and water (reverse osmosis-purified) via automatic watering system or water bottle. Animals were maintained on a 12:12 h light:dark cycle in rooms at 72 °F (±5 °F) and 30–70% relative humidity, and had access to enrichment opportunities (enrichment tubes and nylabones). All animals were determined to be specific-pathogen-free.
KLH
The following different formulations of KLH were utilized and identified as test articles #1–#5: (1) Stellar KLH High Molecular Weight (HMW, GMP grade solution [Stellar Biotechnologies Inc., Port Hueneme, CA]); (2) Stellar KLH Subunit (subunits, GMP Grade solution, [Stellar]); (3) Biosyn VACMUNE® (subunits, High Purity Grade/Clinical Grade (HPG/CG) in water [Biosyn Corporation, Carlsbad, CA]); (4) Imject® Mariculture KLH (mcKLH subunits in water, research grade, Pierce-Thermo Scientific Cat #77649; Rockford, IL]); and (5) Imject® mcKLH (HMW lyophilized in phosphate-buffered saline [PBS], research grade [Pierce; Cat #77600]). Formulation of the test articles was conducted as follows: Stellar KLH HMW (1 mg/ml) was provided as a solution in Dulbecco’s PBS (D-PBS) and no dilution or preparation was required. Stellar KLH Subunit, Biosyn VACMUNE® Subunit, and Pierce Imject® Subunit-each at 20 mg/ml in water-were diluted in water for injection the day of immunization. Pierce Imject® HMW in PBS was supplied lyophilized (20 mg units) and re-constituted in 2 ml ultra-pure water (resulting buffer composition of 0.1 M sodium phosphate and 0.15 M NaCl [pH 7.2]) and diluted in PBS on the day of immunization. Endotoxin levels provided by manufacturers were: Stellar KLH HMW, 0.05 EU/mg; Stellar KLH Subunit, 1.00 EU/mg; Biosyn VACMUNE® Subunit, < 11.7 EU/mg; Pierce Imject® Subunit, < 11.0 EU/mg; Pierce Imject® HMW, not available.
Immunization protocol and sample collection
The 100 µg dose of KLH was consistent across forms/sources of antigen to assure proper comparisons and has commonly been utilized in rat TDAR studies (Kim et al., Citation2007). Animals (n = 10 per group) were shaved 1–3 days in advance of dosing in the area of the injection site. KLH was administered by subcutaneous (SC) injection on Day 1 (Site A, left lateral hip area, flank) and Day 22 (Site B, right lateral hip area, flank). Injection sites were circled with a permanent marker and observed daily. Injection site reactions, if any were present, were measured and appearance characterized. Injection sites were then collected at termination.
Blood samples for anti-KLH antibody response were collected from the lateral tail vein (0.5 ml) on Day 1 prior to immunization and on Days 8, 15, 22 (prior to second immunization), 29, 36, and 43. Blood samples were placed into BD Microtainer® no additive (red top) serum separator tubes and allowed to sit until serum was separated from the whole blood. After clotting, samples were centrifuged (2–8 °C) at 4000 rpm for ≈10 min. Each serum sample was divided into two aliquots and transferred into labeled 0.5 ml cryovials and stored in a freezer set to maintain −70 °C (±10 °C) until shipped on dry ice for evaluation of anti-KLH IgM and/or anti-KLH IgG antibodies.
KLH samples characterization
One aliquot (0.5 mL) from each dose formulation as used for immunization on Day 1 and Day 22 was analyzed for protein concentration analysis (UV spectrophotometer method at 280 nm with appropriate extinction coefficients for each test article). Biophysical and biochemical analyses were also conducted to characterize each dose formulation as used for immunization including the following: protein aggregation defined by particle number, size distribution, and morphology (light obscuration and micro-flow imaging), size and molecular weight (high performance liquid chromatography [HPLC] size exclusion chromatography [SEC]), molecular mass, intrinsic charge, and molecular radius of aggregates (native polyacrylamide gel electrophoresis [PAGE]), and electrophoretic mobility and molecular weight of proteins denaturized into subunit (sodium dodecyl sulfate [SDS]-PAGE). For SEC a reference standard parallel run preceded, and followed, each chromatographic run with a test article. For PAGE and SDS-PAGE, lanes of internal standards (Stellar) and high molecular weight markers (BioRad, Hercules, CA) were included on the left and right of each gel. Reference standard KLH HMW and KLH Subunit compositions were commercial preparations subject to additional purification and analytical characterization and comprised > 98% KLH.
Protein aggregation was evaluated by light obscuration for particle number and size distribution and by micro-flow imaging for morphology to determine the number and characteristics of particles present. Samples were diluted (2–10×) in PBS (Stellar KLH HMW and Pierce Imject HMW) or water (Stellar KLH Subunit, Biosyn VACMUNE Subunit, and Pierce Imject Subunit) to keep the particle counts within the detection limits of the instrument. Samples were degassed in a vacuum chamber at 75 torr for 2 h. For the light obscuration measurement, a HIAC/Royco liquid particle counter model 9703 with an HRLD-150 sensor and the software PharmSpec (HACH Ultra Analytics, Grants Pass, OR) were used. Particle-free water was washed through the system before each sample analysis to provide for a clean baseline. De-gassed samples were gently swirled, and then measured four times at a volume of 0.5 ml/injection. The first run was discarded, and the last three runs were averaged. Particles were counted as differential counts per 0.5 mL in size ranges of 2–5, 5–10, 10–15, 15–20, 20–25, and 25–150 µm. Final particle numbers were adjusted based on the initial dilution factor.
For micro-flow imaging, particle images were captured on a liquid-borne particle MFI System DPA4100 (Brightwell Technologies, Inc., Ottawa, Canada). Particle-free water was flushed through the system before each sample analysis to provide for a clean base line. Degassed samples were gently swirled, and then drawn from a 1-ml pipette tip into the flow cell using a peristaltic pump. A 0.8 ml sample was loaded one at a time; the first 0.3 ml was discarded and only the following 0.5 ml was analyzed. Five hundred images were collected per sample and processed by the system software to extract each particle and its characteristics. An array of typical particle images from each sample and the longest dimension (feret diameter) were used for further comparison.
HPLC size exclusion chromatography (SEC): SEC was performed on a 10/300GL Superose-6B column (GE Healthcare, Piscataway, NJ) in 100 mM Tris (pH 7.4) containing 5 mM CaCl2 and 5 mM MgCl2. KLH subunit preparations were eluted at a flow rate of 1 ml/min and HMW preparations at 0.5 ml/min. Standards were run eight times and test articles in duplicate.
Polyacrylamide gel electrophoresis (PAGE): Native PAGE was performed on precast CriterionTM Tris-HCl 7.5% polyacrylamide gels (BioRad) according to the manufacturer’s instructions using Colloidal Blue Staining (Invitrogen, Carlsbad, CA) and KLH HMW and KLH Subunit standards (Stellar). Absorption at 280 nm was used to confirm protein concentration to achieve equivalent sample loading.
SDS polyacrylamide gel electrophoresis (SDS PAGE): SDS PAGE was performed on pre-cast 3–8% gradient NuPAGE Tris-acetate gels (Life Technologies, Grand Island, NY) according to manufacturer instructions using Colloidal Blue Staining, HiMark high molecular weight markers, and the KLH HMW and KLH Subunit standards. Absorption at 280 nm was used to confirm protein concentration to achieve equivalent sample loading.
Injection site examination and histological evaluation
Injection sites were examined for changes in skin color, temperature, swelling, and/or induration during the in-life phase. At necropsy, injection sites and the surrounding skin were collected from each animal and placed into labeled cassettes and immersed in 10% neutral buffered formalin (NBF). After fixation, tissues were trimmed, embedded in paraffin, sectioned, stained with hematoxylin and eosin (H & E), and evaluated by light microscopy. Reactions were graded for intensity on a 4-grade scale (minimal, mild, moderate, and marked).
Anti-KLH IgM and IgG quantitation
The assay for IgG and IgM antibodies against KLH will be described in greater detail elsewhere (manuscript in preparation). Briefly, we developed an ECL based indirect assay on the Meso Scale Discovery (MSD, Rockville, MD) Sector Imager 6000. KLH subunit-coated MSD plates were blocked and incubated with serum specimens diluted (1:150) in blocking buffer/assay diluent. Each specimen was analyzed in duplicate. After washes in PBS/0.05% Tween-20/0.25 mM Thimerosal to remove non-specific antibodies, rat antibodies against KLH were detected via ruthenium-conjugated Protein G (IgG detector) or ruthenium-conjugated goat anti-rat IgM. After a final wash step, tripropylamine MSD read buffer was added to each well. Using the MSD 6000 plate reader, an electrical current is placed across the plate-associated electrodes. The result is a series of electrically induced oxidation-reduction reactions involving ruthenium (from the captured complex) and tripropylamine, leading to a luminescent signal. The subsequent electrochemiluminescence is measured and is quantified as ECL units. Each plate contained negative (pooled normal rat serum, PNRS) and positive (pooled highly reactive rat specimens from a previous TDAR experiment) controls run in quadruplicate. Primary and secondary IgM and IgG areas under the curve (AUCs) were defined by ECL units across study Days 1–22 and the x-axis, and ECL units across study Days 22–43 and the x-axis using the Log-linear/Linear Trapezoidal Rule.
Statistical analysis
All data were analyzed using SAS v9.2 in the Windows VISTA Professional environment. Statistical significance was evaluated at the p = 0.05 level. Natural log-transformed IgM and IgG anti-KLH titer (ECL) values at each collection timepoint and natural log-transformed AUC (primary and secondary) values were evaluated using a one-way analysis of variance (ANOVA) model. For each ANOVA analysis, if variance heterogeneity among groups was concluded [smaller AICC (Small Sample – Size Corrected Akaike Information Criterion] for the model assuming unequal variances as compared to the model assuming unequal variances, the final model would assume variance heterogeneity across groups. If the overall group effect was statistically significant, the pair-wise comparisons were performed without multiple comparison adjustment given the exploratory nature of the study.
Results
Dose analysis
All dose formulation protein concentrations were within 20% of the 1-mg/ml target concentration (samples ranged between 0.8–1.1 mg/ml on Days 1 and 22) when protein content was analyzed by spectrophotometry using the appropriate extinction coefficient for each test article (data not shown).
Biophysical particle analysis
A significant number of micron size particles were detected in all KLH samples by both light obscuration and micro-flow imaging techniques. However, a significantly greater number of particles in the 2–150 µm size range were detected in both of the KLH HMW samples as compared to the KLH Subunit samples. Both HMW samples also contained the highest number of particles in each of the size ranges tested (). All KLH samples contained a heterogeneous mixture of aggregates that appeared amorphous in morphology (). The largest size aggregates were detected in the Stellar KLH HMW (≥115 µm) and Pierce Imject® HMW (≥70 µm) samples, followed by the Stellar KLH Subunit (≥20 µm), Biosyn VACMUNE® Subunit (≥20 µm) and Imject® Subunit (≥20 µm) samples. The size threshold indicates the lower size limit of the particles that were used for comparison. It is worth noting that dilution of the samples before analysis may have caused reversing of some of the particles to monomer, thus the final particle population observed may be enriched for the irreversible particle component. Particle counts and size distributions were similar for the two KLH HMW samples and for the three KLH Subunit samples, respectively.
Figure 1. Particle number, size distribution, and morphology of KLH preparations from different sources. (a) KLH preparations were examined by light obscuration to determine the number and size range of particles present. The differential particle numbers per milliliter in each size range tested are shown. Bars represent average of three runs. (b) Particle images were captured on a Micro-flow Imaging system and representative images of the largest particles detected are shown. The size threshold designates the lower size limit of the particles that were used for comparison.
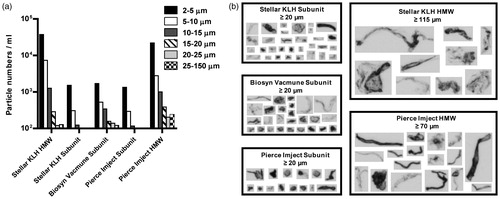
Size exclusion chromatography (SEC)
KLH HMW standard eluted from Superose 6B as a major peak, at a flow rate of 0.5 ml/min at 28.6–28.7 min (); and at a flow rate of 1 ml/min, KLH Subunit standard eluted at 28.5–28.7 min (). Test articles exhibited the same chromatographic behavior as the respective HMW or subunit standards. Elution times for the respective test articles are summarized in . Within the known variations of the assay, no significant differences were observed between the SEC chromatograms of the two HMW test articles and of the three subunit test articles, respectively.
Figure 2. Size Exclusion Chromatography (SEC). HMW KLH standard eluted from Superose 6B as a major peak, at a flow rate of 0.5 mL/min at 28.6–28.7 min (a); and at a flow rate of 1 mL/min, KLH subunit standard eluted at 28.5–28.7 min (b).
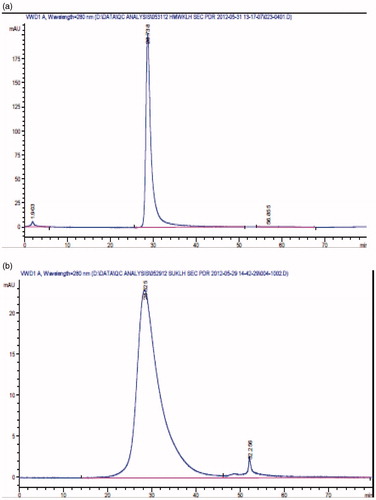
Table 1. Size exclusion chromatography (SEC) of high molecular weight (HMW) and subunit (SU) KLH test articles and standards.
Native polyacrylamide gel electrophoresis (PAGE)
KLH HMW standard and KLH Subunit standard both exhibited the same characteristic three-band pattern on native PAGE, i.e. lanes #8 and #3, respectively, in . All HMW and subunit KLH test articles exhibited the same 3-band pattern, as summarized in . In prior studies (Stellar; unpublished observations), purified KLH HMW and KLH Subunit both exhibit the same characteristic pattern of three bands on native PAGE. By comparison with sequence database information for KLH isoforms KLH1 and KLH2, the PAGE bands were identified as follows: the ∼710 kDa band is the KLH2 isoform (non-dissociated); the ∼669 kDa band is KLH1 (dimeric); and the ∼440 kDa band is KLH2 (monomeric).
Figure 3. Native Polyacrylamide Gel Electrophoresis (PAGE, 3 A): HMW KLH standard and KLH subunit standard both exhibited the same characteristic 3-band pattern on native PAGE, i.e. lanes #8 and #3, respectively. All HMW and subunit KLH test articles exhibited the same 3-band pattern. SDS Polyacrylamide Gel Electrophoresis (SDS PAGE, 3B): HMW-KLH standard (Stellar; C-11-0044) and KLH subunit standard (Stellar; E-113) both exhibited the same characteristic band pattern on SDS PAGE, i.e. lanes #8 and #3, respectively. All HMW and subunit KLH exhibited the same band pattern.
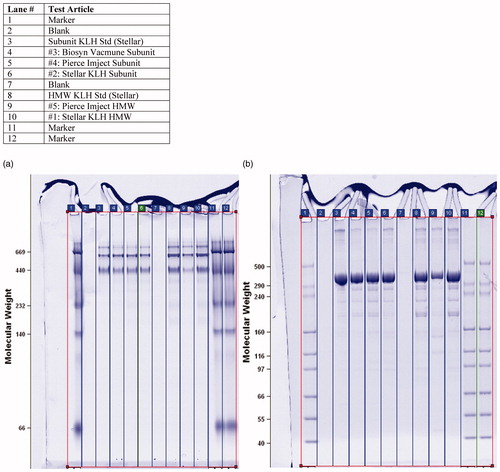
Table 2. Native PAGE analysis of test articles and standards.
SDS polyacrylamide gel electrophoresis (SDS PAGE)
KLH HMW standard (Stellar; C-11-0044) and KLH Subunit standard (Stellar; E-113) both exhibited the same characteristic band pattern on SDS PAGE, i.e. lanes #8 and #3, respectively, in . All HMW and subunit KLH exhibited the same band pattern on SDS PAGE, as summarized in , demonstrating that, after denaturation, all KLH tested have the same chain length, which reflects mass, the sole determinant of the migration rate of proteins in SDS-PAGE. By comparison with sequence database information for KLH isoforms KLH1 and KLH2, the SDS PAGE bands were identified as follows: the ∼550–600 kDa band is KLH1 and KLH2; the ∼160 kDa band is KLH1; the ∼140 kDa band is KLH1; the ∼120 kDa band is also KLH1 (data not shown).
Table 3. SDS PAGE analysis of test articles and standards.
In summary, under the conditions of SEC chromatography on Superose 6B, all HMW and all subunit KLH preparations were equivalent on SEC (size; molecular volume; similar elution patterns); consistent with elution of standards in parallel SEC runs; and in agreement with prior published properties of HMW and various subunit KLH (Swerdlow et al., Citation1996). (Note: under these SEC conditions, higher order particulate aggregates of KLH would not be expected to enter the column matrix). Similarly, native PAGE gel separation of all five test articles based on size and charge resulted in three bands corresponding to KLH1 or KLH2 isoforms; in agreement with internal standards run on the same gels; in agreement with prior identification of gel bands by in-gel digestion and tandem mass spectrometry sequencing; and in agreement with prior published properties of HMW and subunit KLH (Swerdlow et al., Citation1996). Separation of polypeptides in the respective different KLH (HMW and subunit) preparations by SDS-PAGE (based on electrophoretic mobility and molecular radius) resulted in four bands corresponding to either KLH1 or KLH1 and KLH2 isoforms.
Injection site examination and histological evaluation
All test articles were well tolerated. The only clinical observations noted at the injection sites were minimal pinpoint red foci noted on Day 23 in all compound groups (greater prevalence with the Pierce Imject® compounds, least prevalence with the Stellar compounds) and a slight raised bump, ∼1 mm in diameter, in 1–3 animals in the subunit compound groups on Day 25. Light microscopy evaluation of injection sites revealed the presence of minimal mix cell infiltrates consisting of polymorphonuclear inflammatory cells (PMN; neutrophils/eosinophils) with small mononuclear cells (lymphocytes/plasma cells) or minimal mononuclear cell infiltrates. An increased inflammatory response occurred in rats administered Pierce Imject® HMW in comparison to rats administered other antigens ().
Table 4. Severity scores of subcutaneous infiltrates.
Anti-KLH IgM and IgG responses
The results of anti-KLH IgM and IgG analysis are presented in, respectively, and ; the mean ECL responses (AUC values) for each treatment group are presented in . Robust and similar anti-KLH IgG and IgM responses were detected for both sources of HMW KLH (Stellar and Pierce). Generally, the KLH subunit immunizations resulted in lower IgG and IgM responses, with the exception of the Stellar KLH Subunit IgG and IgM responses (secondary responses in particular), which approached the HMW KLH responses. Within the treatment groups (), particularly during the secondary IgG and IgM responses, variability was lowest for the HMW KLH immunizations, both HMW KLHs showing similar AUC percent coefficient of variation (% CV). When comparing both Stellar KLH HMW and Stellar KLH Subunit, the subunit preparation was associated with greater variability. Among the three subunit preparations, the Stellar KLH Subunit was less variable than the Biosyn VACMUNE® and Pierce Imject® subunits, as illustrated by secondary IgG AUC %CVs. The very low variability associated with the Biosyn VACMUNE® Subunit primary IgM and IgG responses is likely related to the very low immunogenicity observed after the first injection, as some animals did not mount a detectable response. The impact of differences of variability associated with the different sources/forms of KLH on animal group size necessary to detect changes (associated with a putative treatment with a test article) is illustrated in .
Figure 4. Ln transformed anti-KLH IgM response. The mean of the duplicates for each animal/timepoint is presented. The line connects the group mean for each timepoint. Group positions on the x-axis are offset slightly to aid visualization. All groups were assayed according to the same schedule: prime and boost of 100 µg KLH (SC) on Days 1 and 22; antibody serum draws were taken on Days 1, 8, 15, 22, 29, 36, and 43.
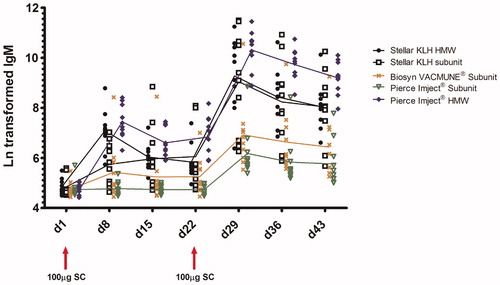
Figure 5. Ln-transformed anti-KLH IgG response. The mean of the duplicates for each animal/timepoint is presented. The line connects the group mean for each timepoint. Group positions on the x-axis are offset slightly to aid visualization. All groups were assayed according to the same schedule: prime and boost of 100 µg KLH (SC) on Days 1 and 22; antibody serum draws were taken on Days 1, 8, 15, 22, 29, 36, and 43.
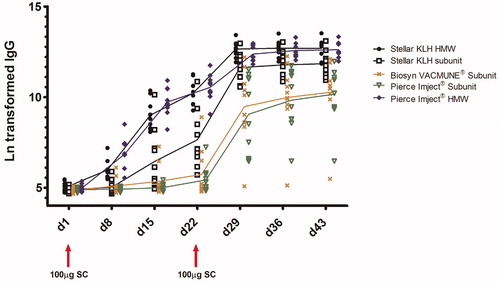
Table 5. Primary and secondary IgM and IgG AUC.
Table 6. Inter-animal variability for primary and secondary IgM and IgG AUC.
Table 7. Impact of KLH form and source on animal group size to detect changes in KLH IgG responses.
Discussion
With the rapid evolution of new immune modulators, substantial resources and attention are being directed toward the evaluation of potential adverse effects of these pharmaceuticals on the immune system. The primary TDAR to antigens is widely recognized, and recommended by regulatory guidelines, as a comprehensive method for in vivo evaluation of immunotoxicity of human pharmaceuticals. Key attributes in selection of appropriate antigen include high tolerability, pharmaceutical quality, robust immunogenicity, and low variability. KLH is well recognized as a standard antigen in TDAR testing and has a four decade established history of safe use in humans and animals as carrier, adjuvant, and agent for immune function testing. KLH represents an ideal neoantigen for TDAR application with translational implications in drug product development from non-clinical studies through post-market surveillance.
Variability in immunogenicity among different preparations and commercial sources of KLH has been reported, suggesting that comparisons of TDAR data generated across laboratories, studies and species may be challenging (Herscowitz et al., Citation1972; Miller et al., Citation2005). The present study provides support that the forms of KLH (HMW and subunit) and manufacturing processes (commercial sources) may have great impact on the magnitude, kinetics, and control of variability in anti-KLH immune responses.
In the present study, all antigens were well tolerated. The only clinical signs noted were minimal small red pinpoints and/or slight raised bumps at the injection site 1–3 days after the second injection. Histopathological evaluation of the injection sites showed that all subunit KLH and the Stellar KLH HMW had no significant or a minor local response to injected antigen consistent with those expected for a nominal response to an injected antigen and do not reflect significant tissue damage.
Immunologists have begun to appreciate that the success of immunization is not only dependent on the nature of vaccine formulations (i.e. immunogen, carrier, and adjuvant), dose, vaccination schedule, and route of administration, but also on certain particulate delivery systems such as liposome, viral-like particles, and bacterial-like particles (Jain et al., Citation2011; Men et al., Citation1996; Manocha et al., Citation2005). Molluscan hemocyanins are decamers, didecamers, and multidecamers of a 340–400 kDa protein subunit. The decamers are short 35 nm particle-like hollow cylinders. In agreement with prior descriptions of preparations of didecameric KLH complexes (Harris et al., Citation1997; Swerdlow et al., Citation1996), the two HMW KLH preparations used in the present study showed a greater number of sub-visible particles (2–150 µm size range) than the three subunit KLH preparations (Harris et al., Citation1997; Swerdlow et al., Citation1996). Nano- or macro particulates are known to display adjuvant properties. In a recent study, Mann et al. (Citation2009) demonstrated that influenza A antigen-entrapped larger lipid particles (400–2000 nm) elicited significantly higher IgG2a and interferon (IFN)-γ responses than smaller (10–100 nm) particles. Using a ferret infection challenge model, vaccination with large lipid vesicles also resulted in greater protection (Feinberg et al., Citation2001). For HMW KLH, larger aggregates may preferentially attach to the surfaces of macrophages or dendritic cells (DC), thus serving as an effective depot system for prolonged and sustained delivery and processing of KLH, which resulted in higher immune responses. High particle numbers in the 2–150 µm size range have been shown to be a necessary and dominant factor in the induction of an aggregate-mediated immune response in vitro (Joubert et al., Citation2012). The high particle numbers detected in the two HMW KLH preparations may in part explain the higher immunogenicity associated with these samples. Furthermore, these results highlight that samples with different aggregate levels can induce varying levels of immunogenic responses.
In this study, all HMW KLH and all KLH subunits were equivalent on SEC (size and molecular weight; similar elution patterns), PAGE (size and charge; three bands corresponding to either KLH 1 or 2 isoforms based on previous information in all five test articles) and SDS-PAGE (molecular radius; four bands corresponding to either KLH1 or KLH1 and 2 for all test articles). Despite finding that no major biochemical and biophysical differences were noted in all three subunit KLH preparations, Stellar KLH Subunit yielded the strongest anti-KLH IgM and IgG responses among the three subunit KLH products ( and ). Similar to the results in this study, Miller et al. (Citation2005) also reported in autologous bone marrow transplant recipients that Biosyn VACMUNE® Subunit had diminished capacity to induce anti-KLH antibodies. The antibody response was corrected with co-administration of Montanide ISA51 (a synthetic oil adjuvant) suggesting that Biosyn VACMUNE® Subunit may have intact antigenicity but diminished adjuvanticity. It has been suggested that adjuvant effects of KLH are mediated by glycan structures through C-lectin receptors (CLR) expressed on DC (Figdor et al., Citation2002; Presicce et al., Citation2008). Important glycan residues including fucose, N-Acetyl-galactos-amine, mannan, and mannose have been identified on KLH and shown to bind DC-SIGN (Dendritic Cell-Specific Intercellular adhesion molecule-3-Grabbing Non-integrin), a C-type lectin receptor present on both macrophages and DC (Kantelhardt et al., Citation2002; Wirguin et al., Citation1995; Wuhrer et al., Citation2004). An intriguing hypothesis is that glycans on KLH that are important for DC activation could be altered or lost during manufacturing processing. Immunomodulatory receptors with specificity for these carbohydrate moieties such as DC-SIGN (Feinberg et al., Citation2001; Gringhuis et al., Citation2009) could account for differences in immunogenicity among sources of KLH. Until there is a clear delineation of KLH glycoprotein biochemistry, in vivo vaccination is the only reliable tool to safeguard consistent immunogenicity of KLH for use in the TDAR assay.
Following processing of the endocytosed KLH, DC migrate to the draining lymph node where they interact with naïve T-cells. DC induce chemokines and homing receptors on KLH-specific T-cells and guide these T-cells to target effector sites (Guy-Grand et al., Citation1974; Kantele et al., Citation1999; McDermott et al., Citation1986). The kinetics of DC activation, migration to draining lymph nodes, and T-cell activation vary between individuals and may contribute to inter-individual variability, especially when serum samples are collected at a single timepoint. The expression of homing receptors on KLH-specific T-cells may also vary with different routes of vaccination, which further complicates the timing of sample collection (Kantele et al., Citation1999). To promote uniform activation of DC and T-cells, the site and route of KLH inoculation, relative to the intended draining lymph nodes, will have to be carefully chosen and rigidly controlled throughout the experiment.
In summary, it is apparent that many factors are involved in achieving strong and consistent anti-KLH IgM and IgG responses. These factors are interconnected, encompassing parameters relating to KLH formulation, manufacturing process, route of administration, and site of KLH inoculation. An optimized KLH formulation and protocol are necessary to offer a sensitive tool to monitor the effect of drugs on the immune system. It is hoped that the information gathered here will assist in the preparation of optimized KLH formulations using reproducible processes so as to achieve and sustain the production of strong and consistent TDAR responses as desired. KLH is a valuable immunotoxicology tool and represents an ideal neoantigen for TDAR application with reports of variability suggesting only the need for greater standardization (Descotes, Citation2009). Improved standardization of KLH source and TDAR protocols holds promise for improving the predictability of immunotoxicity testing in drug product development, especially with regard to the translation of the new immune modulating drugs from non-clinical studies through post-market surveillance. It would be beneficial to test the same antigen in non-clinical and clinical studies to be able to better understand the translatability of this assay.
Declaration of interest
Herbert Chow is an employee and shareholder of Stellar Biotechnologies. John Sundsmo is a former employee of Stellar Biotechnologies. The other authors report no conflicts of interest.
References
- Descotes, J. 2009. Immunotoxicity of monoclonal antibodies. MAbs. 1:104–111
- FDA. 2002. Guidance for industry: Immunotoxicology Evaluation of Investigational New Drugs. Bethesda, MD
- Feinberg, H., Mitchell, D. A., Drickamer, K., and Weis, W. I. 2001. Structural basis for selective recognition of oligosaccharides by DC-SIGN and DC-SIGNR. Science 294:2163–2166
- Figdor, C. G., van Kooyk, Y., and Adema, G. J. 2002. C-Type lectin receptors on dendritic cells and Langerhans cells. Nat. Rev. Immunol. 2:77–84
- Gringhuis, S. I., den Dunnen, J., Litjens, M., et al. 2009. Carbohydrate-specific signaling through the DC-SIGN signalosome tailors immunity to Mycobacterium tuberculosis, HIV-1 and Helicobacter pylori. Nat. Immunol. 10:1081–1088
- Guide for the Care and Use of Laboratory Animals, 8th Edition, 2011. National Academic Press, Washington DC
- Guy-Grand, D., Griscelli, C., and Vassalli, P. 1974. The gut-associated lymphoid system: Nature and properties of the large dividing cells. Eur. J. Immunol. 4:435–443
- Harris, J. R., Gebauer, W., Sohngen, S. M., et al. 1997. Keyhole limpet hemocyanin (KLH), II: Characteristic reassociation properties of purified KLH1 and KLH2. Micron. 28:43–56
- Herscowitz, H. B., Harold, W. W., and Stavitsky, A. B. 1972. Immunochemical and immunogenic properties of a purified keyhold limpet haemocyanin. Immunology 22:51–61
- ICH. 2005. International Conference on Harmonisation of Technical Requirements for Registration of Pharmaceuticals for Human Use, ICH Harmonized Tripartite Guideline S8. Immunotoxicity Studies for Human Pharmaceuticals
- Jain, S., O’Hagan, D. T., and Singh, M. 2011. The long-term potential of biodegradable poly(lactide-co-glycolide) microparticles as the next-generation vaccine adjuvant. Expert Rev. Vaccines 10:1731–1742
- Joubert, M. K., Hokom, M., Eakin, C., et al. 2012. Highly aggregated antibody therapeutics can enhance the in vitro innate and late-stage T-cell immune responses. J. Biol. Chem. 287:25266–25279
- Kantele, A., Zivny, J., Hakkinen, M., et al. 1999. Differential homing commitments of antigen-specific T-cells after oral or parenteral immunization in humans. J. Immunol. 162:5173–5177
- Kantelhardt, S. R., Wuhrer, M., Dennis, R. D., et al. 2002. Fuc(α1→3)GalNAc-: The major antigenic motif of Schistosoma mansoni glycolipids implicated in infection sera and keyhole-limpet haemocyanin cross-reactivity. Biochem. J. 366:217–223
- Kim, C. J., Berlin, J. A., Bugelski, P. J., et al. 2007. Combined analyses of heterogeneous immunotoxicology studies using functional T-cell-dependent antibody response tests. Perspect. Exp. Clin. Immunotoxicol. 1:61–76
- Mann, J. F., Shakir, E., Carter, K. C., et al. 2009. Lipid vesicle size of an oral influenza vaccine delivery vehicle influences the TH1/TH2 bias in the immune response and protection against infection. Vaccine 27:3643–3649
- Manocha, M., Pal, P. C., Chitralekha, K. T., et al. 2005. Enhanced mucosal and systemic immune response with intranasal immunization of mice with HIV peptides entrapped in PLG microparticles in combination with Ulex Europaeus-I lectin as M cell target. Vaccine 23:5599–5617
- McDermott, M. R., Horsewood, P., Clark, D. A., and Bienenstock, J. 1986. T-Lymphocytes in the intestinal epithelium and lamina propria of mice. Immunology. 57:213–218
- Men, Y., Gander, B., Merkle, H. P., and Corradin, G. 1996. Induction of sustained and elevated immune responses to weakly immunogenic synthetic malarial peptides by encapsulation in biodegradable polymer microspheres. Vaccine 14:1442–1450
- Miller, J. S., Curtsinger, J., Berthold, M., et al. 2005. Diminished neo-antigen response to keyhole limpet hemocyanin (KLH) vaccines in patients after treatment with chemotherapy or hematopoietic cell transplantation. Clin. Immunol. 117:144–151
- Presicce, P., Taddeo, A., Conti, A., et al. 2008. Keyhole limpet hemocyanin induces the activation and maturation of human dendritic cells through the involvement of mannose receptor. Mol. Immunol. 45:1136–1145
- Swerdlow, R. D., Ebert, R. F., Lee, P., et al. 1996. Keyhole limpet hemocyanin: Structural and functional characterization of two different subunits and multimers. Comp. Biochem. Physiol. B Biochem. Mol. Biol. 113:537–548
- Weigle, W. O. 1964. Immunochemical properties of hemocyanin. Immunochemistry 1:295–302
- Wirguin, I., Suturkova-Milosevic, L., Briani, C., and Latov, N. 1995. Keyhole limpet hemocyanin contains Gal(β 1-3)-GalNAc determinants that are cross-reactive with the T-antigen. Cancer Immunol. Immunother. 40:307–310
- Wuhrer, M., Robijn, M. L., Koeleman, C. A., et al. 2004. A novel Gal(β1-4)Gal(β1-4)Fuc(α1-6)-core modification attached to the proximal N-acetylglucosamine of keyhole limpet haemocyanin (KLH) N-glycans. Biochem. J. 378:625–632
- Yefenof, E., Zehavi-Feferman, R., and Guy, R. 1990. Control of primary and secondary antibody responses by cytotoxic T-lymphocytes specific for a soluble antigen. Eur. J. Immunol. 20:1849–1853