Abstract
Pongamia pinnata is a plant known for its therapeutic usage in Indian traditional medicine. Despite the controversy regarding toxic flavonoid and erucic acid content, the seed of this plant is consumed in tribal medicine and its oil is used in Ayurveda to treat psoriasis and arthritis. This study explored the potential anti-arthritic effects of a P. pinnata seed (hexane) extract (PSE) at non-lethal doses in an adjuvant-induced arthritic rat model; possible mechanisms of any observed effects were also explored. After establishing the lethal doses arising from oral exposure to the extract, the material was administered per os daily at two doses (0.3 g/kg/day; 0.5 g/kg/day) to arthritic rats. Other rats received indomethacin or vehicle (control). Treatments were performed for a total of 14 days. One day after the final exposure, the rats were euthanized to permit harvest of various cells, blood, and tissues for analyses. Paw diameter and tissue myeloperoxidase activity in the paws were evaluated as indices for edema and neutrophil infiltration into the tissue. The severity of arthritis in the experimental rats was assessed via measures of urinary hydroxyproline (HP) and glucosamine, and of serum pro-inflammatory TNFα and anti-inflammatory IL-10. The extent of NF-κB p65 nuclear translocation in peritoneal macrophages harvested from naïve rats and then treated in vitro was also assessed. The results indicated that exposure to PSE significantly decreased paw diameter, tissue myeloperoxidase level, and levels of urinary HP and glucosamine, as well as of serum TNFα and IL-10 in adjuvant-injected (arthritic) rats. In vitro PSE treatment also resulted in a marked inhibition of NF-κB p65 nuclear translocation in primary cultures of peritoneal macrophages. Thus, PSE appears to be able to prevent experimental arthritis, in part, by helping to maintain the balance between pro- and anti-inflammatory cytokines and by inhibiting NF-κB activation.
Introduction
Rheumatoid arthritis (RA) is a systemic inflammatory autoimmune disorder that affects millions of people all around the world (Venkatesha et al., 2011a). RA is characterized by joint swelling, synovial membrane inflammation, and cartilage destruction. Multiple components of immunity and inflammation, such as the balance between pro- and anti-inflammatory cytokines, have been shown to play a role in the onset and progression of this disease (Kim et al., Citation2008). Nuclear factor-κB (NF-κB) plays an important role in the inducible regulation of a variety of genes involved in the development of inflammatory conditions like RA, including those for mediators such as tumor necrosis factor (TNF)-α and interleukins like IL-10, and IL-6 (Tsao et al., Citation1997). IL-1 and TNFα activate self-proliferation of synoviocytes and cause an increase in tissue enzyme production (e.g. matrix metalloproteinases) by chondrocytes or synovial cells, ultimately resulting in degradation of cartilage (Niki et al., Citation2004). During bone erosion and cartilage degradation, TNFα triggers the expression of other cytokines, endothelial adhesion molecules, stimulates collagenase, and triggers osteoclast differentiation (Bazzoni & Beutler, Citation1996).
Therapeutic approaches for the treatment of RA include non-steroidal anti-inflammatory drugs (NSAIDs) and disease-modifying anti-rheumatic drugs (DMARDs) such as methotrexate and sulfasalazine that aim to modulate pain and inflammation. However, these drugs often have adverse effects including gastrointestinal disorder and cardiovascular complications at high doses or during long-term administration. Owing to the side-effects and high cost of conventional anti-inflammatory drugs, the development of alternative therapeutic methods for management of RA is being increasingly explored by researchers throughout the world (Venkatesha et al., 2011b).
Pongamia pinnata (Linn.) Merr. Syn P. glabra Vent. (PP) has been utilized in Indian traditional medicine against many inflammatory disorders including arthritis and psoriasis, as well as in bronchitis, diarrhea, diabetes, skin diseases, hypertension, and many other diseases (Carcache et al., Citation2003; Kesari et al., Citation2010). This fast growing deciduous tree is widely distributed along Southeast Asia and North Australia (Vadivel & Biesalski, Citation2011). Different parts of the plant have been investigated for anti-inflammatory, anti-ulcerogenic, anti-oxidant, and analgesic properties (Bansod et al., Citation2010; Singh & Pandey, Citation1996; Singh et al., Citation1996; Srinivasan et al., Citation2001). Although the seed oil itself is considered inedible due to a high content of flavonoids and erucic acid (Bala et al., Citation2011), it is still routinely used by tribes in parts of India for the treatment of inflammatory disorders (Silja et al., Citation2008). The cooked seeds of P. pinnata are also consumed by certain tribal sects in India (Vadivel & Biesalski, Citation2011). In vivo studies on the anti-inflammatory activity of extracts prepared from P. pinnata seeds revealed that extracts prepared using petroleum ether and chloroform retained the maximum activity (Singh et al., Citation1996). Prabha et al. (Citation2009) attributed the ulcer-protective effects of a methanolic seed extract to the presence of flavonoids (mainly Karanjin), a finding subsequently confirmed by Vismaya et al. (Citation2011).
Despite containing a natural combination of anti-inflammatory constituents, there has been no scientific report to date on the potential anti-arthritic activity of the seed or its extracts. Accordingly, in this study, the effect of P. pinnata seed extract (PSE) against adjuvant-induced arthritis (AIA) in rats, a widely used experimental model that closely resembles human arthritis where neutrophils, macrophages, and lymphocytes all participate in the development and pathogenesis of the disease (Solomon et al., Citation2005) was investigated. Studies were also undertaken to begin to explain underlying mechanisms for any observed effects.
Materials and methods
Reagents
Complete Freund’s adjuvant, glucosamine, and indomethacin were purchased from Sigma (St. Louis, MO). Hydroxyproline, p-nitrophenyl phosphate, and p-nitrophenol were each bought from SRL (Mumbai, India). Antibody against NF-κB p65 (Alexa Fluor® 488-tagged) was obtained from Santa Cruz Biotechnology, Inc. (Santa Cruz, CA).
Animals
Male Wistar rats (≈120 g) were obtained from the Animal Facility at CNCI (Kolkata, India) and housed in cages in specific-pathogen free facility maintained at 23 [±2] °C, with a relative humidity of 60 [±5]%, and with a 12-h light/dark cycle. All rats had ad libitum access to standard rodent chow and filtered water. All animal experiments were approved by the Animal Ethics Committee of the University of Calcutta and were in accordance with the guidelines of the Committee for the Purpose of Control and Supervision of Experiments on Animal (CPCSEA), Government of India (IAEC Ref no:820/04/ac/CPCSEA.2010).
Preparation of Pongamia pinnata seed extract
Pongamia pinnata seeds were collected from a licensed dealer in Kolkata, India and authenticated at the Department of Pharmacognosy, in the National Research Institute for Ayurvedic Drug Development (Kolkata). The specimen (Seed K-04) was kept in a herbarium in the same department. The mature seeds were air-dried and then coarsely powdered. This material (≈2 kg) was then extracted with hexane (5 L) for 72 h in a Soxhlet apparatus. The solvent was then removed from the extract by distillation over a steam bath and the oily residue (weighing ≈590 g) was collected. This residue was then placed in a vacuum dessicator (5–10 mm Hg) for 48 h to remove the last trace of solvent, and a pure oil was obtained (yield of ∼12.9%). The oil was collected and weighed to ascertain its mass (1 ml PSE oil weighed 1.12 g); this information was then used to deliver the PSE in terms of mass rather than a volume in the subsequent studies outlined herein.
Minimum lethal dose determination and general toxicity of PSE
Minimum Lethal Dose (MLD) determination in the rats was performed according to the methods of Litchfield and Wilcoxon (Citation1949). After weighing, rats were administered a single dose of PSE per os (i.e. by gavage) in graded doses (10–30 g/kg) and lethality was recorded for up to 24 h post-exposure.
General toxicity studies were then carried out in rats by treating them per os with PSE at 0.3 or 0.5 g/kg/day, levels ≈1/50th and 1/75th of the above-determined MLD dose, over a total of 14 days. Food and water intake of the experimental animals along with their body weights were monitored throughout the study period. After 14 days of treatment, the rats were each placed in metabolic cages to permit collection of their urine. The urine collected was qualitatively analyzed using Multistix reagent strips (Siemens, Baroda, India); these could simultaneously assess levels of urobilinogen, protein, blood, ketone, bilirubin, glucose, pH, and specific gravity. To assess any PSE-induced nephrotoxicity, urinary creatinine levels were measured with a commercial kit (Merck Specialities Pvt Ltd., Ponda, India). These rats were euthanized by ether overdose on Day 15 and their blood was collected for analysis. Serum isolated from these blood samples was analyzed for enzymes related to liver function, such as serum glutamate pyruvate transaminase (SGPT) and glutamate oxaloacetate transaminase (SGOT), using commercial kits (Piramal Health Care Ltd., Navi, Mumbai, India). Serum lactate dehydrogenase (LDH) levels were assessed (as a measure of tissue damage or cell death) using a commercial kit from Crest Biosystems (Pune, India).
Induction of arthritis by adjuvant
Arthritis in the rats was induced using Freund’s complete adjuvant (FCA), according to the method of Newbould (Citation1963), as modified by Chakraborty et al. (Citation2010). Briefly, a fine emulsion (0.1 ml) of FCA in olive oil (1:2, v/v; at concentration of 0.25 mg heat-killed Mycobacterium tuberculosis/ml of emulsion) was intradermally injected into the planter surface of the right hind foot of each rat. The day after the injection, drug treatments were initiated in the rats. Groups of rats were to receive daily treatments of PSE per os at one of two doses, i.e. 0.3 or 0.5 g/kg/day, while others received the standard drug indomethacin (1 mg/kg/day), and controls received the olive oil vehicle. Treatment was performed for 13 consecutive days. On Day 14, urine was collected from the rats. The following day, all rats were euthanized by ether overdose, and blood was collected from the hepatic portal vein (for the isolation of serum for assessment of biochemical parameters). At necropsy, the paws were removed and weighed, and the tissues were processed for use in a tissue myeloperoxidase (MPO) assay (see below).
Assessment of paw edema and paw tissue myeloperoxidase (MPO) activity
Arthritis severity was assessed from paw swelling. The day of arthritis induction was considered as Day 0. The paw diameters of all rats were measured using Vernier calipers on Day 0, the first day after adjuvant injection (Day 1), and then every 5 days until Day 15 of the study (for a total of four measures/rat).
The activity of tissue MPO was assessed using the method of Bradley et al. (Citation1982). On Day 15, paw samples from normal, arthritic, PSE-treated, and standard drug-treated rats were isolated. The tissues were then placed in 50 mM potassium phosphate buffer (pH 7.2; containing 1% hexa-decyl-trimethylammonium bromide [HTAB]) to yield a final concentration of 10% [w/v], and homogenized. The resulting homogenates were then centrifuged (10 000 rpm, 4 °C, 15 min) and the supernatant recovered and assayed for MPO activity. In brief, samples (50 µl) were mixed with 50 mM phosphate buffer (500 µl; pH 6.0) containing 1 mM o-dianisidine dyhydro-chloride and 0.001% (v/v) hydrogen peroxide. Absorbance was then kinetically measured (at 480 nm) at 30-s intervals up to 2 min. MPO activity was then expressed as unit MPO/mg tissue protein, where 1 U was defined as a 0.1 absorbance change at 480 nm (Raj et al., Citation1998). Protein levels in each homogenate were determined in parallel using the method of Lowry et al. (Citation1951).
Assessment of urinary parameters
Urinary hydroxyproline (HP) concentration was measured according to Neuman and Logan (Citation1950) and expressed in terms of µg hydroxyproline/day. Glucosamine levels in urine was determined by the method of Elson and Morgan (Citation1933), modified by Chakraborty et al. (Citation2010), and expressed as µg glucosamine/day.
Assessment of select phosphatase enzymes and pro-/anti-inflammatory cytokines in serum
Serum alkaline and acid phosphatase (ACP and ALP) activities were measured via the method of Michell et al. (Citation1970). Serum levels of IL-10 and TNFα were determined using enzyme-linked immunosorbent assay (ELISA) kits purchased from R&D (Minneapolis, MN). All assays were performed according to manufacturer instructions. The limits of detection for the IL-10 and TNFα kits were 12.5 and 31.2 pg/ml, respectively.
In vitro assessment of NF-κB p65 nuclear translocation in macrophages
Peritoneal macrophages were isolated from naïve rats using standard techniques (Tripathi et al., Citation2008). Upon harvesting, the isolated cells were re-suspended in complete RPMI (Himedia, Mumbai, India) containing 10% fetal bovine serum (Gibco, Grand Island, NY). The cells were then seeded onto sterile coverslips (at 2 × 106 cells/ml) and cultured at 37 °C in a 5% CO2 incubator to permit adherence. The following day, the medium was removed and cells were then treated with complete RPMI containing PSE (suspended 1:10 [w/v] in dimethyl sulfoxide [DMSO, 99% purity; Sigma]) for 6 h. To determine non-lethal doses for use in these in vitro studies, PSE (at 1–5 µg/culture) was first tested. At the end of the 6-h treatment period, cell viability was assessed via trypan blue staining to quantify toxicity of the PSE preparation under the experimental conditions.
Based on the results of these pilot studies, another set of naïve rat peritoneal macrophages was obtained and cultured for 24 h as above. The following day, the medium was removed and these cells were then treated with complete RPMI containing 2 µg PSE (suspended 1:10 [w/v] in DMSO) for 6 h. Each culture then received LPS (lipopolysaccharide, Escherichia coli 0111:B4; Sigma) at a final (culture) level of 100 ng/ml, and was incubated a further 18 h at 37 °C. The slides were then removed, washed with phosphate-buffered saline (pH 7.4) and the adherent cells were fixed in 3.7% formaldehyde solution for 20 min. These cells were then permeabilized in 0.1% Triton X-100 in PBS solution for 10 min prior to undergoing incubation for 1 h in a 3% bovine serum albumin solution to block potential non-specific antibody binding. Thereafter, the cells were incubated overnight at 4 °C with 1 µg/ml Alexa 488-conjugated NF-κB p65 primary antibody. After gently washing away any unbound antibody, the cells were counter-stained with 4′,6-diamidino-2-phenylindole DAPI (1 µg/ml; MP Biomedicals, Illkirch, France) to permit nuclear staining. The coverslips were then washed, mounted with DPX (Merck, Mumbai, India), and then confocal images acquired using a disc scanning confocal microscope (Olympus, New Delhi, India) and Andor Bioimaging software (Belfast, UK). Quantitative image analysis was performed using Image J v3.91 software (Noursadeghi et al., Citation2008).
Statistical analysis
All the results were expressed as mean ± SE. Routinely, each mean was obtain from n = 6 values/group. Statistical significance was determined using a one-way analysis of variance (ANOVA) followed by Bonferroni and Tukey’s post-hoc tests. A p value <0.05 was considered as significant.
Results
Toxicity studies with PSE
Using the oral treatment regimens employed here, the minimum lethal dose of PSE was determined to be 28 g/kg body weight. Toxicity studies with PSE delivered daily by gavage for 14 days at doses 0.3 g/kg/day; 0.3 PSE and 0.5 g/kg/day; 0.5 PSE were carried out in rats for preliminary safety evaluation. Over the treatment period, PSE rats remained as healthy as untreated controls, i.e. each displayed normal food and water intake and body weight gain. After 14 days, qualitative urine analysis did not show any significant changes in PSE treated rats as compared to the normal controls. There was no significant change in urinary creatinine as well (). Serum SGOT, SGPT, and LDH levels after PSE treatment were not significantly altered ().
Paw edema and paw tissue MPO activity in adjuvant-treated rats
Paw diameters in 0.3 PSE, 0.5 PSE, and indomethacin (1 mg/kg/day; IND) treated rats showed a marked decrease compared to those in vehicle-treated arthritic counterparts (). Within the first day of treatment (i.e. Day 2), paw diameters were smaller (relative to values in adjuvant-treated rats that received only vehicle) by 9.04, 13.02, and 8.55%, respectively, in the 0.3 PSE, 0.5 PSE, and IND rats compared to vehicle control rats. By Day 14 post-FCA injection, the paw diameter values were significantly (relatively) decreased, again, respectively, by 10.11, 16.29, and 13.74% relative to the adjuvant/vehicle rat values.
Figure 3. Effect of PSE and standard drug treatment(s) on paw diameter in rats with FCA-induced arthritis. *Value significantly different vs vehicle-treated rats (as well as those that received no treatments post-FCA injection [arthritis control]) (p < 0.05).
![Figure 3. Effect of PSE and standard drug treatment(s) on paw diameter in rats with FCA-induced arthritis. *Value significantly different vs vehicle-treated rats (as well as those that received no treatments post-FCA injection [arthritis control]) (p < 0.05).](/cms/asset/78bed7b7-db51-4118-b392-7b7d3aa16344/iimt_a_824931_f0003_b.jpg)
By Day 15, paw tissue MPO levels were significantly decreased in rats that had received the 0.3 PSE, 0.5 PSE, or IND treatments as compared to those in paw samples from vehicle-treated arthritic rats (). The level of reduction in tissue MPO due to the 0.3 PSE treatment was 56.85% and 93.19% for 0.5 PSE. Compared to values seen in samples from the non-FCA-injected control rats, the only FCA-injected rats that still had values that were significantly higher were the vehicle-treated and 0.3 PSE hosts; treatment with 0.5 PSE and IND both resulted in reductions to near-background non-FCA-injected control rat values.
Figure 4. Effect of PSE and standard drug treatment(s) on tissue MPO levels in FCA-induced arthritis. Values shown are mean ± SE (n = 6). Value significantly different vs: *naïve rats (no FCA; Normal Control); #Arthritis and Vehicle-Treated Controls; or, ‡0.3 PSE rats (among FCA-injected rats that received drug treatments) (p ≤ 0.05).
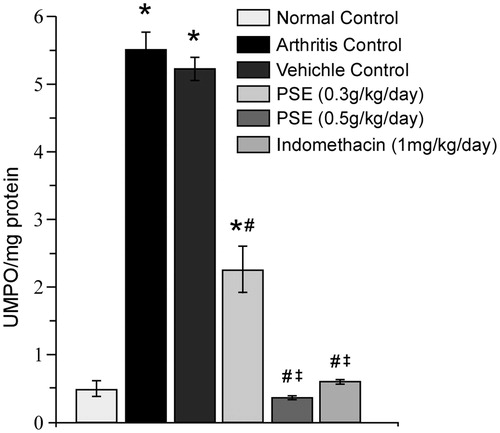
Urinary parameters and serum levels of key enzymes/cytokines in adjuvant-treated rats
Day 14 urinary HP and glucosamine levels were significantly decreased (compared to those in arthritic rats that received vehicle only) as a result of treatment with 0.3 PSE, 0.5 PSE, and IND (). The level of reduction in urinary HP due to the 0.3 PSE regimen was 36.28% and 44.03% for the 0.5 PSE treatments. In regard to urinary glucosamine, the extent of reduction due to the 0.3 PSE regimen was 10.08% and 26.06% for 0.5 PSE. Still, compared to the non-FCA-injected control rats, all FCA-injected rats had values of each parameter that were significantly higher. From both end-points, there appeared to be a dose-related trend in the level of amelioration. Further, with HP, the 0.5 PSE led to a value equivalent to that obtained with IND; this was not the case, however, with glucosamine.
Figure 5. Effect of PSE and standard drug on urinary hydroxyproline and glucosamine level in FCA induced arthritis. Values shown are mean ± SE (n = 6). Value significantly different vs: *naïve rats (no FCA; Normal Control); #Arthritis and Vehicle-Treated Controls; or, ‡0.3 PSE rats (among FCA-injected rats that received drug treatments) (p ≤ 0.05).
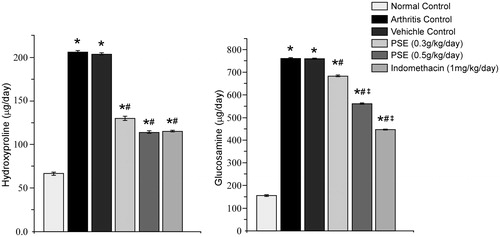
The 0.3 PSE, 0.5 PSE, and IND treatments also led to significant decreases in serum ACP and ALP levels relative to those in vehicle-treated arthritic rat sera (). The level of reduction in ACP due to the 0.3 PSE regimen was 14.81% and 40.40% for the 0.5 PSE treatments. For ALP, these reductions were 30.93% due to 0.3 PSE and 47.27% due to 0.5 PSE. Compared to the non-FCA-injected control rats, all FCA-injected rats still had values of each enzyme that were significantly higher. With both enzymes, there appeared to be a dose-related trend in the level of amelioration. Interestingly, with ACP, the IND treatments led to a value equivalent almost achieving that of the non-arthritic control rats; this was not the case in ALP, however, with IND having the least effect of the treatments; the net change from the vehicle-treated arthritic hosts was only 21.15%, a level significantly higher than that from either PSE regimen.
Figure 6. Effect of PSE and standard drug on serum ACP and ALP level in FCA induced arthritis. Values shown are mean ± SE (n = 6). Value significantly different vs: *naïve rats (no FCA; Normal Control); #Arthritis and Vehicle-Treated Controls; ‡0.3 PSE rats or †IND rats (among FCA-injected rats that received drug treatments) (p ≤ 0.05).
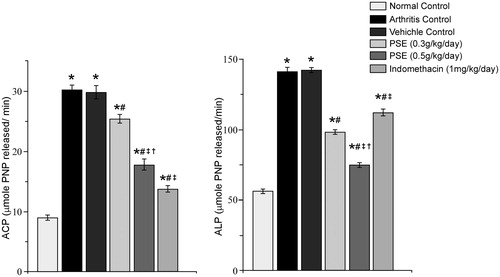
Lastly, by Day 15 of the study, the 0.3 PSE, 0.5 PSE, and IND treatments led to significant decreases in serum TNFα levels relative to those in vehicle-treated arthritic rat sera (). The level of reduction in TNFα levels with 0.3 PSE was 24.29% and 31.69% with the 0.5 PSE treatments. Compared to the non-FCA-injected control rats, only the vehicle-treated and 0.3 PSE FCA-injected rats still had TNFα levels that were significantly elevated. Values for 0.5 PSE and IND rats were each roughly equivalent (and near non-FCA control level).
Figure 7. Effect of PSE and standard drug on serum TNFα and IL-10 level in FCA-induced arthritis. Values shown as mean ± SE (n = 6). Value significantly different vs: *naïve rats (no FCA; Normal Control); #Arthritis and Vehicle-Treated Controls; or ‡0.5 PSE rats (among FCA-injected rats that received drug treatments) (p ≤ 0.05).
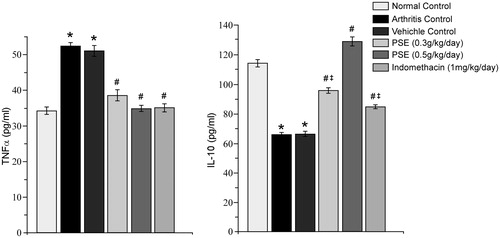
With regard to IL-10, 0.3 PSE, 0.5 PSE, and IND treatments led to significant increases in serum levels of the cytokine relative to vehicle-treated arthritic rat values (). The level of increase in IL-10 levels to 0.3 PSE was 31.22% and 48.62% for 0.5 PSE. Compared to the non-FCA-injected control rats, the vehicle-treated, 0.3 PSE, and IND-treated FCA-injected rats still had IL-10 levels that were still significantly reduced. Values for the 0.5 PSE rats were increased compared to those of the non-FCA normal control level.
NF-κB p65 nuclear translocation in macrophages treated in vitro with PSE
The dose of 2 µg PSE used in these in vitro studies was non-lethal, i.e. 95% cells were viable after 6 h incubation with PSE. Confocal microscopy (used to visualize effect of PSE on NF-κB p65 nuclear translocation after LPS activation) yielded clear evidence that PSE treatment prevented nuclear translocation normally induced by LPS (). In general, there was a significant 78.7% increase in NF-κB p65 nuclear translocation in LPS only-activated macrophages as compared to that seen in un-stimulated control cells. As a result of the PSE treatment, there was a significant 34.6% decrease in translocation compared to levels in the vehicle-treated LPS-activated cells (); these levels were on a par with those in un-stimulated cells. Curiously, the mere presence of the DMSO vehicle alone itself led to a significant (i.e. 28.5%) decrease in translocation compared to levels noted with the LPS only cells.
Figure 8. Microscopic evaluation of the effect of PSE on NF-κB translocation on primary macrophages (Magnification: 60×). (A) Cells were immunostained with NF-κB tagged with Alexa 488 (green/grey hue) and were counter-stained with DAPI (nuclear stained, blue/white hue). Macrophages were pre-treated with PSE (2 µg in 1:10 dilution with DMSO) for 6 h followed by LPS stimulation for 18 h. (a and a1) Normal control; (b and b1) LPS-treated; (c and c1) Vehicle control (DMSO + LPS); and (d and d1) PSE-treated. [a, b, c, d: Merged images of Alexa488 (green/grey hue) and DAPI (nuclear stain, blue/white hue); a1, b1, c1, d1: NF-κB tagged with Alexa488 (green/grey and white hue)]. (B) Images were analyzed (Image J v1.46 software)—ROI analysis was performed and translocations of NF-κB were represented by Alexa488 nucleus:cytoplasm intensity ratios measured from images like those shown in a1, b1, c1, and d1. Values shown as mean ± SE (n = 6). Value significantly different vs: *Normal (no LPS, no vehicle, no PSE); or #LPS-only control (p < 0.05).
![Figure 8. Microscopic evaluation of the effect of PSE on NF-κB translocation on primary macrophages (Magnification: 60×). (A) Cells were immunostained with NF-κB tagged with Alexa 488 (green/grey hue) and were counter-stained with DAPI (nuclear stained, blue/white hue). Macrophages were pre-treated with PSE (2 µg in 1:10 dilution with DMSO) for 6 h followed by LPS stimulation for 18 h. (a and a1) Normal control; (b and b1) LPS-treated; (c and c1) Vehicle control (DMSO + LPS); and (d and d1) PSE-treated. [a, b, c, d: Merged images of Alexa488 (green/grey hue) and DAPI (nuclear stain, blue/white hue); a1, b1, c1, d1: NF-κB tagged with Alexa488 (green/grey and white hue)]. (B) Images were analyzed (Image J v1.46 software)—ROI analysis was performed and translocations of NF-κB were represented by Alexa488 nucleus:cytoplasm intensity ratios measured from images like those shown in a1, b1, c1, and d1. Values shown as mean ± SE (n = 6). Value significantly different vs: *Normal (no LPS, no vehicle, no PSE); or #LPS-only control (p < 0.05).](/cms/asset/11d3f5ca-bc60-40fd-ba52-f4cfd675363e/iimt_a_824931_f0008_b.jpg)
Discussion
Pongamia pinnata seed is a natural combination of anti-inflammatory constituents and is used in the Indian traditional system of medicines, Ayurveda and Unani to treat chronic inflammatory disorders like rheumatism and psoriasis. However, trustworthy traditional knowledge, supported by experimental evidences, is necessary to validate the medicinal values of these plants (Ahmed, Citation2010). In this regard, we explored the anti-arthritis efficacy of PSE, at non-toxic doses in a rat adjuvant-induced arthritis (AIA) model, a model that nearly mimics rheumatoid arthritis (RA) in humans. We also intended in an in vitro study to illustrate a probable mechanism of action induced by PSE for prevention of the disease progression.
The hexane extract of the P. pinnata seed (PSE) and the seed oil have been characterized in detail in various studies (Bala et al., Citation2011; Bimla & Kalidhar, 2004; Birajdar et al., Citation2011; Karmee & Chadha, Citation2005; Shameel et al., Citation1996; Sangwan et al., Citation2010), as is summarized in . Fatty acids, including palmitic, stearic, arachidic, oleic, and linoleic acids, are present, with oleic acid being predominant. There is also an abundance of flavonoids such as karanjin, pongapin, and pongaglabrin. Six compounds (two sterols, three sterol derivatives, one disaccharide) were also reported by Shameel et al. (Citation1996). Trace amounts (i.e., 0.88%) of hiragonic and octadecatrienoic acids were also found. A presence of phytochemical quercitin was also reported in P. pinnata seeds (Khatri & Patel, Citation2012) and also in other plants of the Fabaceae family (Oleszek & Stochmal, Citation2002; Yang et al., Citation2013).
Table 1. Fatty acid profile of Pongamia pinnata hexane seed extract.
Clearly, PSE is a natural combination of flavonoids, polyunsaturated fatty acids, and some other key phytochemicals. Among its varied constituents, linolenic acid, β-sitosterol, and stigmasterol have in particular been shown to impart anti-inflammatory properties (Gabay et al., Citation2010; Loizou et al., Citation2010; Ren & Chung, Citation2007). It was then not surprising to us that treatment with PSE seemed to provide significant protection against fluid accumulation and edema in AIA rats. Edema is generally associated with an increase in vascular permeability, extravasation of fluid/proteins, and cellular infiltration at the site of inflammation (Ramprasath et al., Citation2006).
It was also seen here that myeloperoxidase (MPO) levels that reflect cellular infiltration to the site, particularly neutrophils (Smith, Citation1994), were also significantly decreased due to the PSE treatment. Prokopowicz et al. (Citation2012) recently suggested that MPO has a pro-inflammatory role in general that is also a particular feature of chronic inflammation. In general, MPO released from activated neutrophils catalyzes hydrogen peroxide-mediated oxidation of halide ions to hypohalous acids, especially the very reactive species hypochlorous acid (HOCl). In RA patients, HOCl released from activated neutrophils found in synovial fluid damaged cartilage components (Schiller et al., Citation2003). The findings of the present study demonstrated that oral treatment with PSE led to reduced MPO activity in the paw tissues of AIA rats and this was associated with reductions in edema formation and, probably, damage to cartilage components.
The study here also showed that PSE treatment restored urinary HP and glucosamine levels to near normal. These two products, products of collagen and cartilage matrix glycoprotein degradation, are important markers in AIA and RA (Prockop & Kivirikko, Citation1967). Increases in serum levels of ACP and ALP are also useful markers to reflect significant lysosomal degradation during the inflammation associated with these arthritic states (Narendhirakannan et al., Citation2007; Weissmann, Citation1972). That the PSE treatment significantly decreased ACP and ALP levels in the serum of the treated rats provided further evidence that this material imparted some measure of host protection against lysosomal degradation, and thus mitigated the potential severity of the adjuvant-induced disease.
The loss of maintenance of a balance between pro- and anti-inflammatory cytokines is an important feature in any experimental model of FCA-induced arthritis. Among those signaling products, TNFα is a key pro-inflammatory cytokine secreted from monocytes and macrophages that had been stimulated by activated T-cells (Waksman, Citation2002). It was seen here that administration of PSE significantly reduced serum TNFα levels. Alone, this could explain some of the mitigating effects of the PSE against the arthritic outcomes. However, our studies also showed that the PSE also led to significant increases in serum levels of IL-10, an anti-inflammatory cytokine, that could inhibit (IFNγ)-induced macrophage activation and, as a result, macrophage TNFα production (Oswald et al., Citation1992). Previous studies have shown that oleic acid increases IL-10 levels while linoleic acid decreases them in experimental inflammatory models (Cardoso et al., Citation2011; Magdalon et al., Citation2012). Accordingly, in the present study, the increase in serum IL-10 levels by PSE treatment could be attributed, in part, to a presence of oleic acid, as it was a major component in the extract.
Among the downstream signal transduction regulators in macrophages, NF-κB is an essential transcription factor that regulates gene expression for various cytokines and has a significant role in development of arthritis. Under normal conditions, NF-κB is in an inactive state in the cytosol as it remains bound to its inhibitor IκBα. Normally, inflammatory stimulation leads to phosphorylation and subsequent degradation of IκBα; this, in turn, results in the liberation and nuclear translocation of NF-κB, whereupon it activates transcription of pro-inflammatory genes (Kim et al., Citation2009). It was seen here in an in vitro study that PSE could inhibit NF-κB nuclear translocation in macrophages (upon LPS stimulation). This indicated to us that the anti-inflammatory effect of PSE seen in vivo is likely a result, in part, of an inhibition of NF-κB biochemistry. Moreover, the inhibition of NF-κB translocation caused by the vehicle DMSO is probably due to the anti-inflammatory effect of DMSO as reported in previous studies (Bolduc et al., Citation2001; Colucci et al., Citation2008; Kelly et al., Citation1994). Nevertheless, PSE treatment here caused significant NF-κB inhibition as compared to what was seen in the DMSO-treated LPS-activated cells; this clearly indicated that PSE has inhibitory effects on NF-κB translocation well beyond any that could be attributed to the vehicle itself.
Conclusion
Taking all the findings here together, it can be deduced that PSE inhibited edema formation in the AIA rats by inhibition of neutrophil influx, an essential event for the tissue to return to normal and for the resolution of inflammation. It is likely that changes in NF-κB signaling induced by the PSE led to changes in the release of pro-inflammatory cytokines (directly or indirectly) that, in turn, had impact on immune cell signaling events needed for continued recruitment of neutrophils/other cells to the inflammatory site, their release of cell/tissue damaging products (like HOCl) and, consequently, a continuing progression of disease severity. Which particular components—alone or in combination—within the PSE that provide these effects remains to be determined in ongoing studies in our laboratories.
In conclusion, this study has provided primary evidence in support of an anti-arthritic effect for PSE (as currently used in traditional medicine) and that could also be used to help define the mechanism of action for these effects. In addition, the study data has also provided researchers important information for potentially pursuing the use of PSE as an effective treatment against inflammatory diseases, in particular those sharing an etiology with arthritis.
Declaration of interest
The authors report no conflicts of interest. The authors alone are responsible for the content and writing of the paper.
This work was funded by the Department of Science & Technology, Government of West Bengal [Ref No: 666 (Sanc.)/ST/P/S&T/9G-6/2010].
Abbreviations | ||
AIA, | = | adjuvant-induced arthritis; |
ACP, | = | acid phosphatase; |
ALP, | = | alkaline phosphatase; |
FCA, | = | Freund’s Complete Adjuvant; |
HTAB, | = | hexa-decyl-trimethylammonium bromide; |
IL, | = | interleukin; |
IFN, | = | interferon; |
MLD, | = | minimum lethal dose; |
MPO, | = | myeloperoxidase; |
PNP, | = | p-nitrophenol; |
PNPP, | = | p-nitrophenyl phosphate; |
PSE, | = | Pongamia pinnata seed extract; |
RA, | = | rheumatoid arthritis; |
TNFα, | = | tumor necrosis factor-α; |
LPS, | = | lipopolysaccharide; |
NF-κB, | = | nuclear factor-κB. |
Acknowledgement
We are grateful to Centre for Research in Nanoscience and Nanotechnology (CRNN), University of Calcutta and Division of Molecular Medicine, Bose Institute for infrastructural help. We are indebted to Dr Mradu Gupta, Institute of Post Graduate Ayurvedic Education and Research, for consultation on Ayurvedic literature.
References
- Ahmed, S. 2010. Green tea polyphenol epigallocatechin 3-gallate in arthritis: Progress and promise. Arthritis Res. Ther. 12:1–9
- Bala, M., Nag, T. N., and Kumar, S. 2011. Proximate composition and fatty acid profile of Pongamia pinnata, a potential biodiesel crop. J. Am. Oil Chem. Soc. 88:559–562
- Bansod, M. S, Kagathara, V. G., and Somkuwar, A. D. 2010. Evaluation of analgesics and anti inflammatory activity of a poly-herbal formulation. Int. J. Pharm. Tech. Res. 2:1520–1527
- Bazzoni, F., and Beutler, B. 1996. The tumor necrosis factor ligand and receptor families. New Engl. J. Med. 334:1717–1725
- Bimla, M., and Kalidhar, S. B. 2004. Phytochemical evaluation of Pongamia pinnata L. seed oil. Indian J. Pharm. Sci. 3:447–448
- Birajdar, S., Ramesh, S., Chimkod, V., and Patil, C. S. 2011. Phytochemical and physiochemical screening of Pongamia pinnata seeds. Int. J. Biotech. Appl. 3:52–54
- Bolduc, L., Labrecque, B., Cordeau, M., et al. 2001. Dimethyl sulfoxide affects selection of splice sites. J. Biol. Chem. 276:17597–17602
- Bradley, P. P., Priebat, D. A., Christensen, R. D., and Rothstein, G. 1982. Measurement of cutaneous inflammation: Estimation of neutrophils content with an enzyme marker. J. Invest. Dermatol. 78:206–209
- Carcache, B. E., Kang, Y. H., Park, E. J., et al. 2003. Constituents of stem bark of Pongamia pinnata with the potential to induce quinine reductase. J. Nat. Prod. 66:1197–1202
- Cardoso, C. R., Favoreto, S. Jr, Oliveira, L. L., et al. 2011. Oleic acid modulation of the immune response in wound healing: A new approach for skin repair. Immunobiology 216:409–415
- Chakraborty, M., Bhattacharya, S., Bhattacharjee, P., et al. 2010. Prevention of progression of adjuvant induced arthritis by oral supplementation of Indian freshwater mussel (Lamellidens marginalis) aqueous extract in experimental rats. J. Ethnopharmacol. 132:316–320
- Colucci, M., Maione, F., Bonito, M. C., et al. 2008. New insights of dimethyl sulphoxide effects (DMSO) on experimental in vivo models of nociception and inflammation. Pharmacol. Res. 57:419–425
- Elson, L. A., and Morgan, W. T. 1933. A colorimetric method for determination of glucosamine and chondrosamine. Biochem. J. 27:1824–1828
- Gabay, O., Sanchez, C., Salvat, C., et al. 2010. Stigmasterol: A phytosterol with potential anti-osteoarthritis properties. Osteoarthr. Cartilage. 18:106–116
- Karmee, S. K., and Chadha, A. 2005. Preparation of biodiesel from crude oil of Pongamia pinnata. Bioresource Tech. 96:1425–1429
- Kelly, K. A., Hill, M. R., Youkhana, K., et al. 1994. Dimethyl sulfoxide modulates NF-κB and cytokine activation in LPS-treated murine macrophages. Infect. Immun. 62:3122–3128
- Kesari, V., Das, A., and Rangan, L. 2010. Physico-chemical characterization and anti-microbial activity from seed oil of Pongamia pinnata, a potential biofuel crop. Biomass Bioenergy 34:108–115
- Khatri, P., and Patel, R. 2012. An phytochemical overview of various parts of Pongamia pinnata (Karanj). World J. Pharm. Res. 2:146–165
- Kim, D. H., Shin, E. K., Kim, Y. H., et al. 2009. Suppression of inflammatory responses by celastrol, a quinone methide triterpenoid isolated from Celastrus regelii. Eur. J. Clin. Invest. 39:819–827
- Kim, H. R., Rajaiah, R., Wu, Q. L., et al. 2008. Green tea protects rats against autoimmune arthritis by modulating disease-related immune events. J. Nutr. 138:2111–2116
- Litchfield, J. T., and Wilcoxon, F. 1949. A simplified method of evaluating dose-effect experiment. J. Pharmacol. Exp. Ther. 96:99–102
- Loizou, S., Lekakis, I., Chrousos, G. P., and Moutsatsou, P. 2010. β-Sitosterol exhibits anti-inflammatory activity in human aortic endothelial cells. Mol. Nutr. Food. Res. 54:551–558
- Lowry, O. H., Rosebrough, N. J., Farr, A. L., and Randall, R. J. 1951. Protein measurement with the Folin-Phenol reagent. J. Biol. Chem. 193:265–275
- Magdalon, J., Vinolo, M. A., Rodrigues, H. G., et al. 2012. Oral administration of oleic or linoleic acids modulates the production of inflammatory mediators by rat macrophages. Lipids 47:803–812
- Michell, R. H., Karnovsky, M. J., and Karnovsky, M. L. 1970. Distributions of some granule-associated enzymes in guinea pig polymorphonuclear leukocytes. Biochem. J. 116:207–216
- Narendhirakannan, R. T., Subramanian, S., and Kandaswamy, M. 2007. Anti-inflammatory and lysosomal stability actions of Cleome gynandra L. studied in adjuvant-induced arthritis in rats. Food Chem. Toxicol. 45:1001–1012
- Neuman, R. E., and Logan, M. A. 1950. The determination of hydroxyproline. J. Biol. Chem. 184:299–306
- Newbould, B. B. 1963. Chemotherapy of arthritis induced in rats by mycobacterial adjuvant. Br. J. Pharmacol. Chemother. 21:127–136
- Niki, Y., Yamada, H., Kikuchi, T., et al. 2004. Membrane-associated IL-1 contributes to chronic synovitis and cartilage destruction in human IL-1α transgenic mice. J. Immunol. 172:577–584
- Noursadeghi, M., Tsang, J., Haustein, T., et al. 2008. Quantitative imaging assay for NF-κB nuclear translocation in primary human macrophages. J. Immunol. Meth. 329:194–200
- Oleszek, W., and Stochmal, A. 2002. Triterpene saponins and flavonoids in the seeds of Trifolium species. Phytochemistry 61:165–170
- Oswald, I. P., Wynn, T. A., Sher, A., and James, S. L. 1992. IL-10 inhibits macrophage microbicidal activity by blocking the endogenous production of TNFα required as a co-stimulatory factor for IFNγ-induced activation. Proc. Natl. Acad. Sci. USA 89:8676–8680
- Prabha, T., Dorababu, M., Goel, S., et al. 2009. Effect of methanolic extract of Pongamia pinnata Linn seed on gastroduodenal ulceration and mucosal offensive and defensive factors in rats. Indian J. Exp. Biol. 47:649–659
- Prockop, D. J., and Kivirikko, K. I. 1967. Relationship of hydroxyproline excretion in urine to collagen metabolism. Ann. Int. Med. 66:1243–1266
- Prokopowicz, Z., Marcinkiewicz, J., Katz, D. R., and Chain, B. M. 2012. Neutrophil myeloper-oxidase: Soldier and statesman. Arch. Immunol. Ther. Exp. 60:43–54
- Raj, D. A., Booker, T. S., and Belcastro, A. N. 1998. Striated muscle calcium-stimulated cysteine protease (calpain-like) activity promotes myeloperoxidase activity with exercise. Pflugers Arch. 435:804–809
- Ramprasath, V. R., Shanthi, P., and Sachdanandam, P. 2006. Immunomodulatory and anti-inflammatory effects of Semecarpus anacardium Linn. nut milk extract in experimental inflammatory conditions. Biol. Pharm. Bull. 29:693–700
- Ren, J., and Chung S. H. 2007. Anti-inflammatory effect of α-linolenic acid and its mode of action through the inhibition of nitric oxide production and inducible nitric oxide synthase gene expression via NF-κB and mitogen-activated protein kinase pathways. J. Agric. Food Chem. 55:5073–5080
- Sangwan, S., Rao, D. V., and Sharma, S. 2010. A Review on Pongamia Pinnata (L.) Pierre: A great versatile leguminous plant. Nature Sci. 8:130–139
- Schiller, J., Fuchs, B., Arnhold, J., and Arnold, K. 2003. Contribution of reactive oxygen species to cartilage degradation in rheumatic diseases: Molecular pathways, diagnosis and potential therapeutic strategies. Curr. Med. Chem. 10:2123–2145
- Shameel, S., Usmanghani, K., Ali, M. S., and Ahmad, V. U. 1996. Chemical constituents from the seeds of Pongamia pinnata (L.) Pierre. Pak. J. Pharm. Sci. 9:11–20
- Silja, V. P., Varma, K. S., and Mohanan, K. V. 2008. Ethnomedicinal plant knowledge of Mullu kuruma tribe of Wayanad district, Kerala. Indian J. Trad. Knowl. 7:604–612
- Singh, R. K., Joshi, V. K., Goel, R. K., et al. 1996. Pharmacological actions of Pongamia pinnata seeds -- a preliminary study. Indian J. Exp. Biol. 34:1204–1207
- Singh, R. K., and Pandey, B. L. 1996. Anti-inflammatory activity of seed extracts of Pongamia pinnata in rat. Indian. J. Physiol. Pharmacol. 40:355–358
- Smith, J. A. 1994. Neutrophils, host defense, and inflammation: A double-edged sword. J. Leukocyte Biol. 56:672–686
- Solomon, S., Kassahn, D., and Illges, H. 2005. The role of the complement and the FcγR system in the pathogenesis of arthritis. Arthritis Res. Ther. 7:129–135
- Srinivasan, K., Muruganandan, S., Lal, J., et al. 2001. Evaluation of anti-inflammatory activity of Pongamia pinnata leaves in rats. J. Ethnopharmacol. 78:151–157
- Tripathi, S., Bruch, D., and Kittur, D. S. 2008. Ginger extract inhibits LPS-induced macrophage activation and inhibition. BMC Complement. Alt. Med. 8:1–7
- Tsao, P. W., Suzuki, T., Totsuka, R., et al. 1997. Effect of dexamethasone on the expression of activated NF-κB in adjuvant arthritis. Clin. Immunol. Immunother. 83:173–178
- Vadivel, V., and Biesalski, H. K. 2011. Contribution of phenolic compounds to the anti-oxidant potential and Type II diabetes related enzyme inhibition properties of Pongamia pinnata L. Pierre seeds. Process Biochem. 46:1973–1980
- Venkatesha, S. H., Yu, H., Rajaiah, R., et al. 2011a. Celastrus-derived celastrol suppresses autoimmune arthritis by modulating antigen-induced cellular and humoral effector responses. J. Biol. Chem. 286:15138–15146
- Venkatesha, S. H., Rajaiah, R., Berman, B. M., and Moudgil, K. D. 2011b. Immunomodulation of autoimmune arthritis by herbal CAM. Evid.-Based Complement Alt. Med. 2011:1–13
- Vismaya, S. M., Rajashekhar, S., Jayaram, V. B., et al. 2011. Gastroprotective properties of karanjin from Karanja (Pongamia pinnata) seeds: Role as anti-oxidant and H+, K+-ATPase inhibitor. Evid.-Based Complement Alt. Med. 2011:1–10
- Waksman, B. H. 2002. Immune regulation in adjuvant disease and other arthritis models: Relevance to pathogenesis of chronic arthritis. Scand. J. Immunol. 56:12–34
- Weissmann, G. 1972. Lysosomal mechanisms of tissue injury in arthritis. New Engl. J. Med. 286:141–147
- Yang, W. S., Jeong, D., Nam, G., et al. 2013. AP-1 pathway-targeted inhibition of inflammatory responses in LPS-treated macrophages and EtOH/HCl-treated stomach by Archidendron clypearia methanol extract. J. Ethnopharmacol. 146:637–644