Abstract
Exposure to amphibole asbestos has been associated with production of autoantibodies in mice and humans, and increases the risk of systemic autoimmune disease. However, epidemiological studies of chrysotile exposure have not indicated a similar induction of autoimmune responses. To demonstrate this difference in controlled exposures in mice, and to explore possible mechanistic explanations for the difference, C57BL/6 mice were exposed intratracheally to amphibole or chrysotile asbestos, or to saline only. Serum antinuclear antibodies (ANA), antibodies to extractable nuclear antigens (ENA), serum cytokines, and immunoglobulin isotypes were evaluated 8 months after the final treatment. The percentages of lymphocyte sub-sets were determined in the spleen and lungs. The results show that amphibole, but not chrysotile, asbestos increases the frequency of ANA/ENA in mice. Amphibole and chrysotile both increased multiple serum cytokines, but only amphibole increased IL-17. Both fibers decreased IgG1, without significant changes in other immunoglobulin isotypes. Although there were no gross changes in overall percentages of T- and B-cells in the spleen or lung, there was a significant increase in the normally rare populations of suppressor B-cells (CD19+, CD5+, CD1d+) in both the spleen and lungs of chrysotile-exposed mice. Overall, the results suggest that, while there may be an inflammatory response to both forms of asbestos, there is an autoimmune response in only the amphibole-exposed, but not the chrysotile-exposed mice. These data have critical implications in terms of screening and health outcomes of asbestos-exposed populations.
Introduction
Systemic autoimmune diseases (SAID), such as rheumatoid arthritis (RA), systemic lupus erythematosus (SLE), and systemic sclerosis (SSc) occur when abnormalities in immune regulation lead to tissue damage by self-reactive lymphocytes, resulting in severe debilitating symptoms and sometimes death. Several environmental agents, including crystalline silica and asbestos, appear to increase the risk of SAID, possibly through the production of excess cellular debris in the context of a highly inflammatory environment (Brown et al., Citation2005; Cooper et al., Citation2008; Pfau et al., Citation2004). However, the exact mechanisms by which exposure to silicate dusts drive autoimmune responses are not clearly elucidated, and it is not known whether this is a universal response to inhaled mineral dusts.
Silica has long been known to lead to increased formation of anti-nuclear autoantibodies (ANA) and an enhanced risk of SLE, RA, and SSc (Brown et al., Citation2003, Citation2004; Cooper et al., Citation2008). Similarly, exposure to amphibole asbestos results in the development of autoantibodies in non-autoimmune prone mice as well as in humans (Pfau et al., Citation2005, Citation2008, Citation2009). Amphibole is a form of asbestos that consists of long, straight fibers, as opposed to the ‘serpentine’, curly form seen in the commercial asbestos, chrysotile. In our mouse model, the mice exposed to amphibole asbestos (tremolite) exhibited immune complex deposition in the kidneys and mild glomerular changes suggestive of lupus nephritis (Pfau et al., Citation2008). Most importantly, two of the major autoantibodies produced in our mouse model were anti-chromatin and anti-Ro52, both of which are commonly seen in cases of SAID, especially lupus. Asbestos-exposed human subjects also showed a high frequency of these two antibodies (Pfau et al., Citation2005, Citation2009), validating the mouse model as a critical tool.
Epidemiology work based in Libby, Montana, where significant exposures occurred due to the mining and widespread use of amphibole asbestos-contaminated vermiculite, has revealed an increased risk of SAID in that population (Noonan et al., Citation2006). However, while several studies support changes in ANA, rheumatoid factor, or increased overall serum IgG in amphibole-exposed groups, there have been few reports of increased autoimmune responses in humans exposed to chrysotile (reviewed in Bunderson-Schelvan et al., Citation2011). There appear to be three possible explanations for the difference in reported SAID: First, asbestos exposure cohorts tend to be small, and autoimmune changes may have been overlooked. Second, Bernstein et al. (Citation2013) have shown that chrysotile is less biopersistent than amphibole, likely leading to a shorter time in contact with the immune system. Third, there is evidence that chrysotile may induce long-term immunosuppressive effects among lymphocytes sub-sets, leading to susceptibility to cancer but not autoimmune responses (Nishimura et al., Citation2013; Otsuki et al., Citation2007).
These findings led us to expose mice to the different fibers in order to compare change in immune system components 8 months post-exposure that might help elucidate the differences between the fibers’ effects and thus reveal mechanistic etiologies for asbestos-induced auto-antibodies.
Materials and methods
Six-mix and chrysotile fibers
Six-mix (6-Mix) asbestos, a mixture of amphibole fibers from the vermiculite mine in Libby, MT, was provided by the USGS. Intermediate chrysotile was provided by the National Toxicology Program (Dr Dori Germolec). All fibers were suspended in sterile phosphate-buffered saline (PBS, pH 7.4; Sigma, St. Louis, MO), and sonicated (Branson Ultrasonics, Danbury, CT) for 5 min prior to use to minimize aggregation of the fibers. Characteristics of these fibers are summarized in .
Table 1. Fiber summary for Libby 6-Mix and intermediate chrysotile samples.
Mice
The Idaho State University Institutional Animal Care and Use Committee approved all experiments on mice (IACUC protocol #692-0213). The mice used were wild-type C57BL/6 (Jackson Laboratories, Bar Harbor, ME) raised in-house in the Idaho State University Animal Care Facility. These mice were housed under specific pathogen-free (SPF) conditions with a 12 h light–dark cycle, constant temperature (22 °C) and humidity (45%), and ad libitum access to standard rodent chow and filtered water. Only female mice between 6–8-weeks-of-age were used. Initially, 12 mice were to be instilled in each group, but mice were lost due to death during the 8 months, or excess skin barbering that required euthanasia. Thus, the study ended up examining a total of seven saline, 10 chrysotile, and 11 6-Mix exposed mice.
Intratracheal instillations
Exposing mice to 6-Mix, chrysotile, or saline was done by injection of the suspension directly into the trachea. The fiber suspension was created by collecting 1.0 mg dry fiber in a 1.5 ml microcentrifuge tube and adding 1.0 ml sterile PBS, followed by sonication. Mice were injected in the peritoneum with a mixture of 50 µl Xylazine, 40 µl Ketamine, and 40 µl Torbugesic solution (all from MWI Veterinary Supply, Boise, ID) for sedation and then their necks were wiped down with ethanol wipes and shaved. An incision exposed the trachea and 30 µl of the 1 mg/ml fiber suspension (or saline) was injected down the trachea. The incision was closed using an adhesive and cleaned using Betadyne. All mice were allowed to recover from the procedure in a warmed cage. All mice received two instillations 3–4 weeks apart (total exposure = 60 µg of either 6-Mix or chrysotile) and were monitored for 8 months thereafter. Because is it very difficult to extrapolate an appropriate bolus dose that would represent accumulated human exposure over time, an exposure regimen was used that induced fibrosis or autoantibodies as in previous studies with 6-Mix in these mice (Pfau et al., Citation2008; Smartt et al., Citation2010). While a single exposure regimen has induced autoantibodies in these mice, the second exposure improves the reproducibility and magnitude of the autoantibody response (unpublished data). At the end of the 8 month period, mice were euthanized by CO2 asphyxiation (according to IACUC guidelines) and tissues including blood, spleen, and lungs were harvested.
Anti-nuclear antibody (ANA) assay
Serum samples from cardiac punctures were analyzed for anti-nuclear antibodies (ANA). At necropsy, blood was drawn from the heart using a 1.0 ml syringe and collected into microtainer tubes that contained a serum separator (Becton-Dickinson, San Jose, CA). Once the serum was collected, it was stored at 4 °C. The ANA test (ImmunoConcepts, Sacramento, CA), a semi-quantitative analysis that uses indirect immunofluorescence to detect any auto-reactive antibodies in the serum, was modified for the use of mouse serum.
Each serum sample was diluted 1:40 (for screening) by adding 5 µl serum to 195 µl PBS. Diluted sample (20 µl) was then added to dedicated wells on a substrate slide and incubated at room temperature (RT) for 30 min. The slides were then washed with PBS, being careful not to cross-contaminate any samples. The slide was then submerged in PBS for 10 min and then dipped in deionized water 5-times. The slide was then carefully dried by blotting in between the wells.
Fluorescent antibody reagent was prepared by adding 2 µl of goat anti-mouse IgG anti-body conjugated to AlexaFluor 488 (Invitrogen, Eugene, OR) to 1 ml PBS. Antibody solution (20 µl) was then added to each well and incubated in the dark at RT for 30 min. The slide was washed as outlined before and 4–5 drops of Fluorsave (Calbiochem, La Jolla, CA) was added along the mid-line of the slide. A coverslip was then placed on the slide (at angle) and any air bubbles pushed out of the wells. The slides were then viewed using a FITC (488 nm) filter on a Leica DMRB fluorescent microscope in the Advanced Imaging Core Facility at ISU.
RELISA ENA multiparameter antibody screening test
Nuclear antibodies screened by an RELISA ENA test (ImmunoConcepts, Sacramento, CA) were Sm (Smith), RNP, SSA/Ro, SSB/La, Scl-70, and Jo-1. These antibodies were selected for study as each has been associated with several autoimmune syndromes.
Manufacturer protocols for the kit were followed, except as modified for testing mouse serum. Specifically, serum samples were diluted 1:40 by adding 25 μl serum to 975 μl of kit sample diluent. Next, 100 μl of positive and negative control were placed in each of the eight wells in, respectively, kit Multi-parameter strips 1 and 2; diluted sample was added into each of the eight wells of one Multi-parameter strip. The strips were then incubated at RT for 30 min before the samples/controls were removed and the wells washed with PBS containing 0.05% (v/v) Tween-20 (PBS-T, wash buffer). The plates were washed 3–5 times with 250 µl wash buffer/well for 60 s. Plates were tapped on a paper towel to remove all traces of residual wash buffer. After this, 100 μl kit-provided Enzyme Antibody Reagent (EAR) was added to all control wells; horseradish peroxidase (HRP)-conjugated goat anti-mouse IgG (Thermo Fisher Scientific, Pittsburgh, PA) diluted 1:1000 (3 μl in 3 ml PBS) was added to the serum sample wells. All strips were then incubated for 30 min at RT. The EAR and anti-mouse IgG were removed and all wells were washed with PBS-T 3–5 times as above. Aliquots of kit Substrate Solution (100 μl) were then dispensed to each well at a steady rate, so that each well could be incubated at RT for exactly 30 min. Reactions were stopped by adding 100 μl kit Stop Reagent to each well. Reagent was added in the same order to ensure that each well was incubated exactly 30 min. Within 30 min after adding Stop Reagent, the plate was read at 450 nm in a BioTek plate reader (Winooski, VT) and analyzed using Gen 5.0 software in the ISU Molecular Research Core Facility (MRCF).
ELISA for autoantibodies to histone or chromatin
Serum samples were tested for the presence of antibodies to histone or chromatin using specific ELISA kits from Inova Diagnostics (San Diego, CA), according to manufacturer instructions, but modified for mouse serum. Briefly, serum samples were diluted 1:500 in assay diluent and then added to the pre-coated wells (in duplicate) in the 96-well plate. Following incubation at RT, the plates were washed thoroughly and secondary antibody-enzyme conjugate (anti-mouse IgG-HRP, 1:500; Pierce Antibodies, Rockford, IL) was added to the wells. Following incubation and washing, 100 μl kit TMB (3,3′,5,5′-tetramethylbenzidine) substrate was added, followed by 100 μl kit Stop Reagent after color had developed. The plates were then read at 450 nm using the plate reader. To establish positive vs negative readings (since kit was designed for human samples), known ANA− mouse sera were used to set the negative values.
Flow cytometry for lymphocyte sub-sets
Harvested spleens and lungs were each thoroughly minced between frosted ends of glass slides in a petri-dish containing cell culture media (RPMI with 10% fetal bovine serum; CellGro MediaTech, Herndon, VA), and each suspension transferred to 15 ml centrifuge tubes. After larger debris had settled out (10 min at RT), the material above the debris was transferred into a clean 15 ml tube and centrifuged to pellet the cells. Red blood cells (RBC) were eliminated using lysis buffer (eBioscience, San Diego, CA) according to manufacturer protocol, i.e., the cells were re-suspended in lysis buffer for 5 min and then the suspension diluted 5× in PBS and centrifuged (500 × g, 3 min, RT). The pellet was re-suspended in flow cytometry staining buffer (PBS with 1% bovine serum albumin [BSA]) and the cells counted in a Z-series counter (Beckman Coulter, Brea, CA).
Cells were then aliquoted into microfuge tubes at ≈106 cells/sample in 100 μl staining buffer. All antibodies (BD Biosciences, San Jose, CA) were then added at 0.5 μg/106 cells. Sets of cells were stained as follows:
B-cells: CD19 (PE) or IgM (PerCP Cy5.5).
B1a B-cells: IgMpos (PerCP Cy5.5), CD5pos (APC), CD23neg (PE).
B-suppressor cells: CD19pos (PE), CD5pos (APC), CD1dpos (FITC), CD11bpos (PerCP Cy5.5).
T-regulatory cells: CD3pos (PerCP Cy 5.5), CD4pos (PE), CD25pos (FITC), Fox P3pos (APC).
Cells were allowed to stain for 30 min at 4°C (in the dark), and then washed with 1 ml ice-cold PBS. Staining was then analyzed on a FACS Calibur flow cytometer (BD Biosciences) using accompanying Cell Quest software. Isotype control antibodies (4-color) were used to define background staining; < 1% of these controls were allowed in the M1 gate for percent positive. Lymphocytes were determined based on forward- and side-scatter; major sub-sets were then identified based on the first two antibodies listed above in each set using 2-color dot-plots. Tight polygonal regions were used (instead of quadrants) to identify the ultimate sub-populations. A minimum of 10,000 events per sample was captured.
TH1/TH2/TH17 cytometric bead array (CBA)
The cytometric bead array (CBA) mouse TH1/TH2/TH17 cytokine kit (BD Biosciences) examined seven cytokines in a single sample using fluorescent beads that were analyzed via flow cytometry. The cytokines included interleukin (IL)-2, -4, -6, -17, and -10, interferon (IFN)-γ, and tumor necrosis factor (TNF)-α, and are associated with one of the three T-helper cell profiles, TH1, TH2, and/or TH17. The cytokines belonging to the TH17 profile have been associated with autoimmune diseases. The CBA procedure was carried out according to manufacturer instructions. To prepare the top standard, all seven of the lyophilized standard spheres were added to a 15 ml conical tube. The spheres were dissolved in 2 ml of kit assay diluent and incubated at RT for at least 15 min. The top standard was then serially diluted until a ratio of 1:256 was reached. A negative standard was also used that only contained assay diluent.
Creating the mixture of capture beads was done by calculating the appropriate amount of each bead solution to use based on the number of samples for a particular experiment. All seven bead solutions were added to a single 5 ml centrifuge tube (Argos Technologies, Elgin, IL). Fifty microliters of capture bead mixture was added to each 1.5 ml microcentrifuge assay tube. Another 50 µl of standard and sample were added appropriate assay tubes. PE detection reagent (50 μl) from the kit was added to all assay tubes and the mixtures incubated in the dark for 2 h at RT. Kit wash buffer (1 ml) was added to all assay tubes and then each was centrifuged at 200 × g for 5 min. The supernatant was carefully removed, still leaving a tiny amount of fluid to ensure the pellet was not discarded. The pellet was then re-suspended in 300 µl wash buffer and the sample analyzed in the FACS Calibur system for acquisition and analysis following instrument set-up using the provided instrument set-up bead sets. A minimum of 2100 events per sample (300 events per bead set) was captured.
Immunoglobulin isotyping
Mouse serum immunoglobulins were isotyped using a mouse multiplex bead array (BD Biosciences), according to manufacturer instructions. Briefly, serum samples were diluted 1:50 in assay diluent and, along with the kit positive and negative controls, the samples were added to the mixed-bead set. After incubation at RT for 15 min, the beads were washed and then incubated with kit detector (secondary) antibodies at RT for 15 min. The beads were then washed once and re-suspended in assay buffer for analysis on the FACS Calibur system. A minimum of 3000 events per sample was captured. All data were expressed in terms of median fluorescence intensity (MFI).
Statistics
Experiments detecting lymphocytes in the spleen and lungs were analyzed using a non-parametric t-test (Mann-Whitney U Test). The cytokine data were analyzed by comparing means of the treated groups to the control using a 2-tailed t-test. The mean values (±standard error of mean [SEM]) are reported as bars in the figures. Percent positive anti-nuclear antibody results were analyzed using Chi-squared tests to compare treated groups to saline control. Statistical significance for all experiments was determined by a p value < 0.05.
Results
Anti-nuclear antibodies increased in mice exposed to amphibole 6-Mix
Intratracheal treatment with amphibole 6-Mix asbestos led to a significant increase (p = 0.02) in the frequency of positive ANA tests compared to saline treatment (). Of 11 mice exposed to 6-Mix, eight were ANA+ (72.7%). Only one of seven mice treated with saline presented with ANA (14.2%). Of the 10 mice treated with chrysotile, only two displayed ANA (20%), which was not significantly different from saline control (p = 0.64).
Figure 1. Amphibole asbestos increased the frequency of positive ANA tests. The presence of positive ANA tests was evaluated by indirect immunofluorescence on commercially-prepared slides. One mouse of seven (14.1%) exposed to saline was determined to be ANA+; two mice exposed to chrysotile were ANA+ (20%); eight mice exposed to 6-Mix were ANA+ (72.7%). n = 7–11. *Value significantly different compared to saline value using a chi-squared test (p < 0.05).
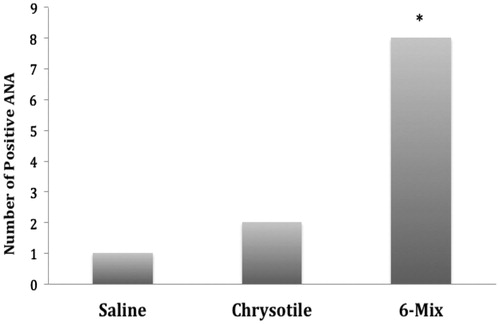
Identification of staining patterns and antigen targets for ANA
Serum samples showing a positive result for ANA by IIF were further studied for the presence of antibodies to ENA, histone, and chromatin. The single ANA+ saline-treated mouse tested positive for Sm, RNP, and Jo-1 (). The two ANA+ samples among the chrysotile-treated mice were both positive homogeneous staining patterns and both were positive for anti-bodies to chromatin -- but negative for antibodies to histone -- suggesting anti-dsDNA (data not shown). These sera were also positive for ENA, including Sm, Jo-1, and SSB-La (). Amphibole-treated mice tested positive for various ENA, with Sm and Jo-1 the most frequent. Despite all but one of the ANA+ amphibole-treated mice showing a mixed homogeneous and speckled staining pattern, no samples tested positive for anti-histone, and only one tested positive for chromatin (data not shown). This suggests a specific epitope not detected by this assay.
Figure 2. Graphical representation of frequency of autoantibodies to specific ENA antigens. ENA autoantibodies were evaluated by Multi-parameter screening ELISA using samples from the ANA+ mice from . Several mice had multiple ENA specificities in the serum. This graph shows the frequency of different ENA antibodies in each treatment group. Data indicate that patterns of ENA specificities seem to be similar for the two fiber treatments.
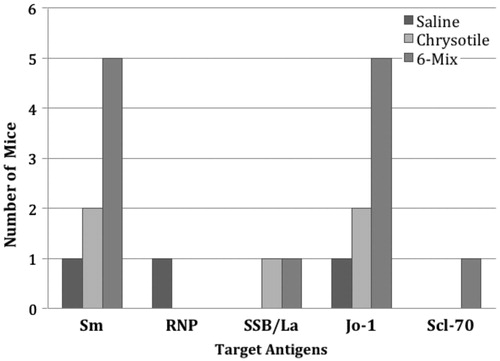
Chrysotile and amphibole both reduce IgG1 sub-type antibodies
There were no significant changes in IgM or IgA in the sera of mice treated with fibers compared to saline (data not shown). However, both fibers induced a consistent reduction in IgG1 among the IgG subtypes (), leading to an altered IgG2/IgG1 ratio suggestive of an inflammatory (TH1) response ().
Figure 3. Exposure effects on serum IgG1 levels. Immunoglobulin sub-types were evaluated by mouse isotyping bead array. (a) No significant differences in any isotypes or sub-types except IgG1 were seen. (b) IgG2/IgG1 ratios. Error bars = SEM; *Value significantly different compared to saline value (p < 0.05).
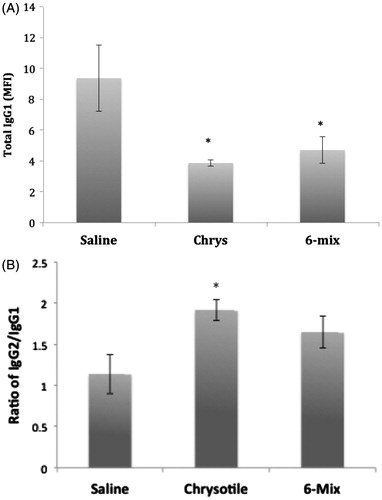
Lymphocyte sub-sets in spleen and lungs of mice exposed to chrysotile or amphibole
There were no changes in overall percentages of B- or T-cells in the spleens or lungs of the mice (data not shown). The percentage of B1a B-cells in the spleen increased in both chrysotile- and 6-Mix-exposed mice (). However, the percentage of B-suppressor cells in the spleen increased significantly only in the mice exposed to chrysotile. Mice exposed to chrysotile also showed a statistically significant increase in percentage of B1a cells in the lungs compared to in the lungs of the saline-treated mice, as well as an increase in the percentage of B-suppressor cells in the lungs. The mice exposed to 6-Mix did not show an increase in lung B-suppressor cells in either the spleen or lungs compared with values in their saline counterparts. There was a slight increase in the percent of regulatory T-cells (CD4+, CD25+, FoxP3+) in the spleens of mice exposed to chrysotile, but not 6-Mix; however, this increase was not statistically significant (p = 0.06 by 2-tailed t-test). The average percentages were: saline = 3.7 (SEM = 1.16), 6-Mix = 3.95 (SEM = 0.48), chrysotile = 5.28 (SEM = 0.96).
Figure 4. Lymphocyte sub-sets in spleen and lungs of mice treated with saline, chrysotile, and 6-mix and then examined after 8 months. Percentages of key lymphocyte subsets in spleen and lungs of mice were evaluated by multi-color flow cytometry. All data are shown as percentages of gated lymphocytes (based on forward- and side-scatter). Error bars = SEM; n = 7–11. *Value significantly different compared to saline value (p < 0.05).
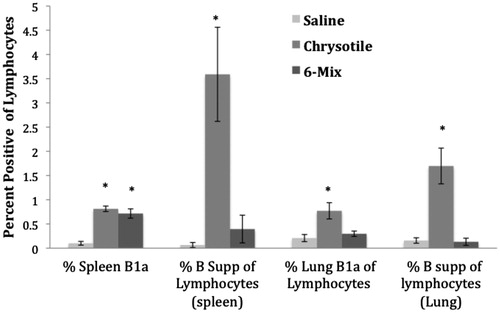
Exposure to amphibole 6-Mix increased production of TH17 serum cytokines
shows the production of the cytokines in the TH1 (), TH2 (), and TH17 () pathways, in response to the fibers. The mice that were exposed to amphibole 6-Mix showed an increase in the production of IL-17 (). Amphibole 6-Mix also caused an increase in production of IL-2, IFNγ (), and IL-6 (); however, chrysotile also caused an increase in these cytokines. Of the TH2 cytokines, neither IL-4 nor IL-10 were significantly elevated with either fiber, although there is a distinct trend for an increase in IL-10 in the 6-Mix exposed mice (, p = 0.06 by 2-tailed t-test). Overall, both fibers increased inflammatory cytokine levels, but only amphibole increased both cytokines critical for any TH17 response.
Figure 5. Induced changes in TH1, TH2, and/or TH17 cytokines. Cytokines attributed to the different T-helper cell responses were evaluated using the mouse TH1/TH2/TH17 Cytokine Bead Array (BD Biosciences). (a) TH1, (b) TH2, and (c) TH17 cytokines. Error bars = SEM. *Value significantly different compared to saline value (p < 0.05), for each individual cytokine.
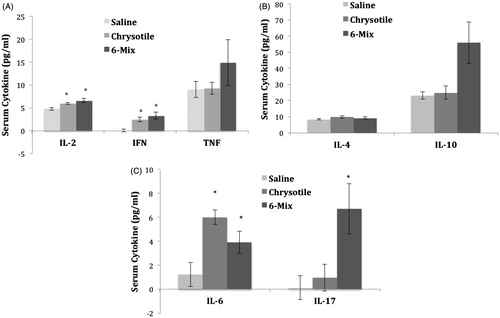
Discussion
Although the definition of asbestos includes both families, amphiboles and serpentine (chrysotile), fibers in these groups are clearly different morphologically, and have unique physicochemical properties (Craighead et al., Citation1980). As a broad group of silicate fibers, asbestos agents, following their inhalation, have been shown to give rise to lung carcinoma, interstitial fibrosis (asbestosis), pleural scarring, and mesothelioma. Any generalization regarding health outcomes must be interpreted with caution, however, since the term ‘asbestos’ is minerologically imprecise, and exposures are rarely pure in terms of fiber type (Case et al., Citation2011; Sporn, Citation2011). This imprecision has led to considerable debate regarding the pathogenicity of different fiber types (Gwinn, Citation2011; Gwinn et al., Citation2011; Lenters et al., Citation2011). While the debate is not completely resolved, current studies continue to support the contention that at least for extended exposures, chrysotile has the ability to induce both cancer and pulmonary/pleural fibrosis (Spyratos et al., Citation2012; van der Bij et al., Citation2013; Wang et al., Citation2013). There is, however, quite a bit of evidence that, at similar exposures, amphibole asbestos is more pathogenic, especially in terms of scarring of the lung parenchyma and pleura (Donaldson et al., Citation2010; Mossman et al., Citation2011). A prevailing theory on the etiology of this difference is that amphibole asbestos is more biopersistent than chrysotile, leading to more accumulation in tissues (Bernstein et al., Citation2005; Sanchez et al., Citation2009).
While there is ample data to support that hypothesis, we wondered whether there might also be a difference in terms of the immunological responses to the fiber types that could further elucidate the health outcomes of exposures, since the presence of ANA has been shown to correlate with lung disease severity or progression in amphibole-exposed cohorts (Tamura et al., Citation1996; Pfau et al., Citation2005). This hypothesis is also supported by the work by a Japanese group (Kumagai-Takei et al., Citation2011, Citation2013) that showed there is a long-term immunosuppression in chrysotile-exposed subjects that could limit the amount of fibrosis, or autoimmunity, but may lead to increased susceptibility to cancer. Comparisons with silica also support the hypothesis that chrysotile does not induce the chronic immune activation/inflammation seen with silica that seems to drive the elevated risk for autoimmune diseases among silica exposed subjects (Otsuki et al., Citation2007). In addition, an in vitro comparison of the effects of 6-Mix and chrysotile on THP-1 monocytic cells and on epithelial cells showed differences in the overall inflammatory/inflammasome response to these fibers (Li et al., Citation2012). While each fiber activated the NLRP-3 inflammasome, the response with amphibole appeared to be mediated by reactive oxygen species; the response with chrysotile may have been due to lysozomal rupture. Therefore, there are differential effects of these fibers even in very early innate immune responses that could greatly impact downstream consequences.
When we first described the increased risk with asbestos exposure for autoimmune responses including autoantibodies (Pfau et al., Citation2005) and autoimmune disease (Noonan et al., Citation2006), we focused on the population of Libby, Montana, where extensive exposures to amphibole asbestos have occurred due to the mining and use of contaminated vermiculite from early in the 20th Century. There have been several other reports of populations exposed to asbestos in which there were increases in ANA, rheumatoid factor, or simply increased serum IgG levels (reviewed in Bunderson-Schelvan et al., Citation2011). In most of these studies, the asbestos fibers were either amphibole or occupational exposures where the specific fibers were not identified but a mixture of amphibole and chrysotile may be assumed (Noonan & Pfau, Citation2011). Only a few of the reports specifically identified chrysotile as the exposure (Bunderson-Schelvan et al., Citation2011). Chrysotile has been used in many more commercial applications than amphibole, including insulation, fabrics, brake linings, and floor tiles. Therefore, there are more occupationally exposed cohorts compared to amphibole exposure, which occurs more commonly from environmental exposures where the fibers are disturbed during excavation and mining. The lack of association between chrysotile exposure and autoimmunity in the literature is therefore striking, and suggests that this may be a very important difference in terms of the immunotoxicology of these fibers.
C57BL/6 mice were used here to compare autoimmune responses following exposure to amphibole asbestos with exposure to chrysotile. This strain of mouse has been shown to be susceptible to pulmonary fibrotic changes in response to both chrysotile and amphibole asbestos (Smartt et al., Citation2010; Sullivan et al., Citation2008), and to autoimmune responses with exposure to amphibole (Pfau et al., Citation2008). We are unaware of any study that has assessed serological markers of autoimmunity in chrysotile-exposed mice. For the current study, the amphibole was obtained from the USGS from the Libby mine site and has been described as ‘6-Mix’ because it was collected from six different sites, combined, and characterized (Meeker et al., Citation2003). It is a combination of amphiboles including winchite, richterite, tremolite, and amosite, and is very likely similar to material that the miners and townspeople were exposed to over decades of mining the asbestos-contaminated vermiculite. The chrysotile asbestos used here was provided by the NIEHS as a well-characterized intermediate-sized serpentine fiber (NTP, Citation1990). The mice were given two spaced doses, based on the hypothesis that the autoantibodies result from repeat events of accumulated dead cell debris in the context of inflammation and lost tolerance (Brown et al., Citation2005; Cooper et al., Citation2008). While we have seen ANA increases in these mice with a single 30 μg dose of amphibole (Pfau et al., Citation2008), the second dose improves the consistency and magnitude of responses. As dosages were on a mass basis, there are differences in numbers of fibers and total surface area to which the mice were exposed (see ). However, since the surface area per mass of chrysotile is higher than with amphibole, using mass-based dosing would seem to increase the effective dose of chrysotile (relative to 6-Mix) based on some studies showing that increased surface area may increase the pathogenicity of fibers (Aust et al., Citation2011; Hillegass et al., Citation2010). It was, therefore, very interesting that, overall, the immune parameters measured in the mice were significantly lower with chrysotile compared to amphibole.
Previously, we reported on antibodies to specific antigens, including extractable nuclear antigens (ENA), in tremolite-exposed C57BL/6, and showed that there was a predominance of antibodies to dsDNA and SSA/Ro, with antibodies to RNP and Scl70 also present (Pfau et al., Citation2008). In the current study, positive ANA tests frequently had homogeneous patterns, suggestive of antibodies to chromatin, similar to our previous work with tremolite, but the assays used did not clearly distinguish whether the antibodies were primarily to histone or dsDNA. This may have been due to the target antigen not being detected by this assay, or from a lack of sensitivity when modifying the clinical assay for testing mouse serum. In addition, we found that mice treated with amphibole 6-Mix showed an increase in the antibodies to Sm and Jo-1, with Scl-70 and SSB/La antibodies also being present. The Smith antibody (Sm) that is frequently linked with SLE and the Jo-1 antibodies are linked to dermatomyositis, but are also seen in autoimmune patients with pulmonary manifestations (Ingegnoli et al., Citation2012; Marie et al., Citation2013). Jo-1 antibodies were also shown to be predominant in rats exposed to amphibole asbestos (Salazar et al., Citation2012). Antibodies to the antigen RNP are not correlated to a specific autoimmune disease, but they are found in a variety of autoimmune diseases. Anti-SSB/La is linked with Sjögren’s Syndrome and antibodies to Scl-70 are found in patients with progressive systemic sclerosis. All of the ENA antibodies found in this mouse study have also been reported in humans exposed to Libby 6-Mix, including high frequencies of RNP, Sm, Scl-70, and Jo-1 (Pfau et al., Citation2005, Citation2009). It has been suggested that all of these antibodies are consistent with the hypothesis that the source of self-antigen in silicate-induced autoantibodies includes apoptotic cells (Brown et al., Citation2005; Casciola-Rosen et al., Citation1994; Cooper et al., Citation2008; Hall et al., Citation2004; Pfau et al., Citation2004).
Also consistent with our previous work, there were no significant changes in the major immunoglobulin isotypes in mice treated with either fiber. Both fibers led to a reduction in the amount of IgG1 in the serum, leading to a more IgG2 dominated response which has been characterized as a more inflammatory or TH1 profile and similar to what has been seen in mice after silica exposure (Brown et al., Citation2004). Overall, the serum cytokine profile with both fibers supports a shift to an inflammatory response, with two key differences between the two exposure types. First, only 6-Mix induced serum IL-17, and the combination of cytokines strongly suggests a TH1/TH17 profile in the 6-Mix-exposed mice. IL-17 has been found in the synovium of people with rheumatoid arthritis, and over-production of IL-17 has been linked to patients with SLE, multiple sclerosis, and chronic inflammatory bowel disease (Afzali et al., Citation2007). However, 6-Mix also induced more serum IL-10 (although not statistically significant due to variability), normally considered either a TH2 or immunosuppressive cytokine. We were interested in studying IL-10 primarily because B-suppressor cells have been shown to help regulate autoimmune responses in mice and IL-10 is highly implicated as a mechanism for that regulation (Kalampokis et al., Citation2013; Watanabe et al., Citation2010). We, therefore, hypothesized that there would be higher levels of IL-10 in chrysotile-exposed mice, especially in view of the increased percentages of suppressive B-cells found only in the chrysotile-exposed mice. It will be necessary to follow-up on the local environment of the suppressive B-cells to determine whether they are playing an active role in the relatively suppressed immune changes in the chrysotile mice. Interestingly, in addition to the suppressive B-cells, we also saw differences in the percentages of B1a B cells in the lungs of exposed mice. These cells are concentrated in the peritoneal and pleural cavities, secrete ‘natural antibodies’, are implicated in autoimmunity, and have been shown to traffic to the spleen and lymph nodes in response to amphibole asbestos (Duan and Morel, Citation2006; Pfau et al., Citation2013; Yang et al., Citation2007). Again, their specific role in the amphibole-induced autoimmunity requires further study.
In conclusion, it was found that exposure to amphibole 6-Mix, but not chrysotile, could affect the long-term outcome of autoimmunity in C57BL/6 mice. Exposure to amphibole 6-Mix caused an increase in presence of ANA in mouse serum significantly more frequently than did exposure to chrysotile. Amphibole 6-Mix caused an increase in antibodies to Sm and Jo-1, both of which are linked to autoimmune diseases. While exposure to amphibole 6-Mix and chrysotile both caused a decrease in IgG1 production and an increase in levels of inflammatory cytokines -- suggesting an inflammatory response -- only amphibole 6-Mix caused an increase in serum IL-17. From these findings, it can be concluded that exposure to amphibole 6-Mix increases the risk for autoimmunity in mice, while exposure to chrysotile asbestos does not. One hypothesis that needs to be explored is the possibility that lack of association of autoimmunity with chrysotile exposure was related to a long-term immunosuppression (Nishimura et al., Citation2013; Otsuki et al., Citation2007); this seems to be suggested here by the increased frequency of a regulatory sub-set of B-cells (B-suppressor cells) in both the lungs and spleen of chrysotile-exposed mice. In addition, repetition of this study in other mouse strains and with larger numbers of animals would help clarify the relevance to humans, and also help explore the possible contribution of genetic susceptibility. Clearly, this work will require confirmation by comparing the serology of populations exposed to chrysotile vs amphibole; that work is currently underway. A better understanding of their potential role in modulating asbestos-induced autoimmunity could have very important implications for exposed populations.
Declaration of interest
The authors report no conflicts of interest. The content of this manuscript is solely the responsibility of the authors and does not necessarily represent the official views of the National Institutes of Health.
Acknowledgements
This work was funded in part by NIH Grant #R15 ES-21884. Core facilities at Idaho State University (Advanced Imaging and MRCF) that supported this work are funded in part by NIH Grant #P20 GM103408 (INBRE). The authors gratefully acknowledge the intellectual contributions of the Libby Epidemiology Research Program (ATSDR/CDC TS000099) team, including Curtis Noonan (PhD), University of Montana; Roger Diegel (MD), Kalispell, MT; Brad Black (MD), Libby, MT; Stephen Levin (MD), Jaime Szeinuk (MD), and Raja Flores (MD), Icahn School of Medicine at Mt Sinai, New York.
References
- Afzali, B., Lombardi, G., Lechler, R. I., and Lord, G. M. 2007. The role of T-helper 17 (TH17) and regulatory T-cells (Treg) in human organ transplantation and autoimmune disease. Clin. Exp. Immunol. 148:32–46
- Aust, A. E., Cook, P. M., and Dodson, R. F. 2011. Morphological and chemical mechanisms of elongated mineral particle toxicities. J. Toxicol. Environ. Health 14:40–75
- Bernstein, D., Rogers, R., and Smith, P. 2005. The biopersistence of Canadian chrysotile asbestos following inhalation: Final results through 1 year after cessation of exposure. Inhal. Toxicol. 17:1–14
- Bernstein, D., Dunnigan, J., Hesterberg, T., et al. 2013. Health risk of chrysotile revisited. Crit. Rev. Toxicol. 43:154–183
- Brown, J. M., Archer, A. J., Pfau, J. C., and Holian, A. 2003. Silica accelerated systemic autoimmune disease in lupus-prone New Zealand mixed mice. Clin. Exp. Immunol. 131:415–421
- Brown, J. M., Pfau, J. C., and Holian, A. 2004. Immunoglobulin and lymphocyte responses following silica exposure in New Zealand mixed mice. Inhal. Toxicol. 16:133–139
- Brown, J. M., Pfau, J. C., Pershouse, M. A., and Holian, A. 2005. Silica, apoptosis, and autoimmune. J. Immunotoxicol. 1:177–187
- Bunderson-Schelvan, M., Pfau, J. C., Crouch, R., and Holian, A. 2011. Non-pulmonary outcomes of asbestos exposure. J. Toxicol. Environ. Health 14:122–152
- Casciola-Rosen, L. A., Anhalt, G., and Rosen, A. 1994. Autoantigens targeted in systemic lupus erythematosus are clustered in two populations of surface structures on apoptotic keratinocytes. J. Exp. Med. 179:1317–1330
- Case, B. W., Abraham, J. L., Meeker, G., et al. 2011. Applying definitions of “asbestos” to environmental and “low-dose” exposure levels and health effects, particularly malignant mesothelioma. J. Toxicol. Environ. Health 14:3–39
- Cooper, G. S., Gilbert, K. M., Greidinger, E. L., et al. 2008. Recent advances and opportunities in research on lupus: Environmental influences and mechanisms of disease. Environ. Health Perspect. 116:695–702
- Craighead, J. E., Mossman, B. T., and Bradley, B. J. 1980. Comparative studies on the cytotoxicity of amphibole and serpentine asbestos. Environ. Health Perspect. 34:37–46
- Donaldson, K., Murphy, F. A., Duffin, R., and Poland, C. A. 2010. Asbestos, carbon nanotubes and the pleural mesothelium: A review of the hypothesis regarding the role of long fibre retention in the parietal pleura, inflammation and mesothelioma. Particle Fibre Toxicol. 7:5--21
- Duan, B., and Morel, L. 2006. Role of B-1a cells in autoimmunity. Autoimmun. Rev. 5:403–408
- Gwinn, M. R. 2011. Multiple modes of action of asbestos and related mineral fibers. J. Toxicol. Environ. Health 14:1–2
- Gwinn, M. R., Devoney, D., Jarabek, A. M., et al. 2011. Meeting report: Mode(s) of action of asbestos and related mineral fibers. Environ. Health Perspect. 119:1806–1810
- Hall, J. C., Casciola-Rosen, L., and Rosen, A. 2004. Altered structure of autoantigens during apoptosis. Rheum. Dis. Clin. North Am. 30:455–471
- Hillegass, J. M., Shukla, A., Macpherson, M. B., et al. 2010. Mechanisms of oxidative stress and alterations in gene expression by Libby six-mix in human mesothelial cells. Part. Fibre Toxicol. 7:26--40
- Ingegnoli, F., Lubatti, C., Ingegnoli, A., et al. 2012. Interstitial lung disease outcomes by high-resolution computed tomography (HRCT) in Anti-Jo1 antibody-positive polymyositis patients: A single center study and review of the literature. Autoimmun. Rev. 11:335–340
- Kalampokis, I., Yoshizaki, A., and Tedder, T. F. 2013. IL-10-producing regulatory B-cells (B10 cells) in autoimmune disease. Arthritis Res. Ther. 15 (Suppl 1):1--5
- Kumagai-Takei, N., Maeda, M., Chen, Y., et al. 2011. Asbestos induces reduction of tumor immunity. Clin. Dev. Immunol. 2011:481–489
- Kumagai-Takei, N., Nishimura, Y., Maeda, M., et al. 2013. Effect of asbestos exposure on differentiation of cytotoxic T-lymphocytes in MLR of human PBMCs. Am. J. Respir. Cell Mol. Biol. 49:28--36
- Lenters, V., Vermeulen, R., Dogger, S., et al. 2011. A meta-analysis of asbestos and lung cancer: Is better quality exposure assessment associated with steeper slopes of the exposure-response relationships? Environ. Health Perspect. 119:1547–1555
- Li, M., Gunter, M. E., and Fukagawa, N. K. 2012. Differential activation of the inflammasome in THP-1 cells exposed to chrysotile asbestos and Libby “six-mix” amphiboles and subsequent activation of BEAS-2B cells. Cytokine 60:718–730
- Marie, I., Josse, S., Hatron, P. Y., et al. 2013. Interstitial lung disease in anti-Jo-1 patients with anti-synthetase syndrome. Arthritis Care Res. (Hoboken) 65:800–808
- Meeker, G. P., Bern, A. M., Brownfield, I. K., et al. 2003. The composition and morphology of amphiboles from the Rainy Creek Complex, Near Libby, Montana. Am. Minerologist 88:1955–1969
- Mossman, B. T., Lippmann, M., Hesterberg, T. W., et al. 2011. Pulmonary endpoints (lung carcinomas and asbestosis) following inhalation exposure to asbestos. J. Toxicol. Environ Health 14:76–121
- Nishimura, Y., Maeda, M., Kumagai-Takei, N., et al. 2013. Altered functions of alveolar macrophages and NK cells involved in asbestos-related diseases. Environ. Health Prev. Med. 18:198–204
- Noonan, C. W., and Pfau, J. C. 2011. Asbestos exposure and autoimmune disease. In: Encyclopedia of Environmental Health (Nriagu, J., Ed.). Burlington, MA: Elsevier, pp. 193–203
- Noonan, C. W., Pfau, J. C., Larson, T. C., and Spence, M. R. 2006. Nested case-control study of autoimmune disease in an asbestos-exposed population. Environ. Health Perspect. 114:1243–1247
- NTP (National Toxicology Program). 1990. Lifetime Carcinogenesis Studies of Chrysotile Asbestos (CAS No. 12001-29-5) in Syrian Golden Hamsters (Feed Studies). NTP Technical Report. Research Triangle Park, NC: U.S. Department of Health and Human Services
- Otsuki, T., Maeda, M., Murakami, S., et al. 2007. Immunological effects of silica and asbestos. Cell. Mol. Immunol. 4:261–268
- Pfau, J. C., Blake, D. J., and Fritzler, M. J. 2009. Autoantibody profiles of an asbestos-exposed population. In: Autoimmunity: Role, Regulation, and Disorders (Vogel, F. L., and Zimmer-mann, L. F., Eds.). New York: Nova Science Publishers, pp. 245–268
- Pfau, J. C., Brown, J. M., and Holian, A. 2004. Silica-exposed mice generate autoantibodies to apoptotic cells. Toxicology 195:167–176
- Pfau, J. C., Hurley, K., Peterson, C., et al. 2013. Activation and trafficking of peritoneal B1a B-cells in response to amphibole asbestos. J Immunotoxicol. (Epub ahead of print)
- Pfau, J. C., Sentissi, J. J., Li, S., et al. 2008. Asbestos-induced autoimmunity in C57BL/6 mice. J. Immunotoxicol. 5:129–137
- Pfau, J. C., Sentissi, J. J., Weller, G., and Putnam, E. A. 2005. Assessment of autoimmune responses associated with asbestos exposure in Libby, Montana, USA. Environ. Health Perspect. 113:25–30
- Salazar, K. D., Copeland, C. B., Wood, C. E., et al. 2012. Evaluation of anti-nuclear antibodies and kidney pathology in Lewis rats following exposure to Libby amphibole asbestos. J. Immunotoxicol. (Epub ahead of print)
- Sanchez, V. C., Pietruska, J. R., Miselis, N. R., et al. 2009. Biopersistence and potential adverse health impacts of fibrous nanomaterials: What have we learned from asbestos? Wiley Interdiscip Rev. Nanomed. Nanobiotech. 1:511–529
- Smartt, A. M., Brezinski, M., Trapkus, M., et al. 2010. Collagen accumulation over time in the murine lung after exposure to crocidolite asbestos or Libby amphibole. Environ. Toxicol. 25:68–76
- Sporn, T. A. 2011. Mineralogy of asbestos. Recent Results Cancer Res. 189:1–11
- Spyratos, D., Chloros, D., Haidich, B., et al. 2012. Chest imaging and lung function impairment after long-term occupational exposure to low concentrations of chrysotile. Arch. Environ. Occup. Health 67:84–90
- Sullivan, D. E., Ferris, M., Pociask, D., and Brody, A. R. 2008. The latent form of TGFβ1 is induced by TNFα through an ERK specific pathway and is activated by asbestos-derived reactive oxygen species in vitro and in vivo. J. Immunotoxicol. 5:145–149
- Tamura, M., Tokuyama, T., Kasuga, H., et al. 1996. Study on correlation between chest X-P course findings and change in anti-nuclear antibody in asbestos plant employees. Sangyo Eiseigaku Zasshi 38:138–141
- van der Bij, S., Koffijberg, H., Lenters, V., et al. 2013. Lung cancer risk at low cumulative asbestos exposure: Meta-regression of the exposure-response relationship. Cancer Causes Control 24:1–12
- Wang, X., Yano, E., Lin, S., et al. 2013. Cancer mortality in chinese chrysotile asbestos miners: Exposure-response relationships. PLoS One 8:e71899
- Watanabe, R., Ishiura, N., Nakashima, H., et al. 2010. Regulatory B-cells (B10 cells) have a suppressive role in murine lupus: CD19 and B10 cell deficiency exacerbates systemic autoimmunity. J. Immunol. 184:4801–4809
- Yang, Y., Tung, J. W., Ghosn, E. E., et al. 2007. Division and differentiation of natural antibody-producing cells in mouse spleen. Proc. Natl. Acad. Sci. USA 104:4542–4546