Abstract
The current study investigated the combinatorial effect of pravastatin (PRAV) and bone marrow mononuclear cells (BM-MNC) on acute myocardial infarction (AMI) induced experimentally in rats. After induction of MI, rats were given oral PRAV (20 mg/kg/day) for 28 days or a bolus intravenous injection (via lateral vein) of a total of 14 × 106 autologous BM-MNC or a combination of both. Serum brain natriuretic peptide (BNP) and histologic changes in cardiac tissues were assessed. Cardiac contents of lipid peroxides, superoxide dismutase (SOD) and inflammatory biomarkers including tumor necrosis factor (TNF)-α and interleukin (IL)-1β as well as vascular endothelial growth factor (VEGF) and nitric oxide (NO) were also measured. Combined PRAV and BM-MNC treatment significantly suppressed serum BNP. Cardiac cell apoptosis and inflammatory cell infiltration in heart tissue decreased significantly in both the PRAV and the PRAV + BM-MNC groups. Cardiac lipid peroxides along with TNFα and IL-1β levels were significantly reduced in both the PRAV and PRAV + BM-MNC hosts with an increase in SOD levels. However, the combined treatment increased cardiac NO levels and did not modify cardiac VEGF levels. The current results indicated that administration of BM-MNC improved the therapeutic efficacy of PRAV treatment by improving the morphology of infarcted hearts as well as decreasing inflammation in a host, but did not do so by inducing therapeutic angiogenesis.
Introduction
Cardiovascular disease (CVD) is the leading cause of morbidity and mortality, not only in the Western world, but also in developing countries, according to a report from the World Health Organization (Lopez and Murray Citation1998). Myocardial infarction (MI) is a common presentation of CVD (Liu et al. Citation2009). Some clinical trials established the benefits of statins after MI. Statins were reported to improve the endothelial function by mechanisms that are unrelated to a cholesterol-lowering effect. Some of these mechanisms involve prevention of inflammation, down-regulation of systemic inflammatory responses (Liu et al. Citation2009) and induction of angiogenesis (Hamelin and Turgeon Citation1998). In a previous study, therapy with pravastatin was found to decrease the risk of recurrent coronary events after MI in patients with average cholesterol levels; this outcome may have resulted in part from anti-inflammatory as well as lipid-lowering properties of the drug (Ridker et al. Citation1998). Nevertheless, current drug therapies do not compensate the loss of cells, a fundamental problem of heart failure (HF) and so are of limited long-term value. Heart transplantation, with functional tissue replacing dysfunctional myocardium, is currently the only radical treatment for end stage HF. However, the low number of donor hearts restricts the widespread use of heart transplantation (Wang and Sjoquist Citation2006).
Therapeutic angiogenesis and cardiac function were improved in experimental animal models with ischemic hearts after implantation of bone marrow mononuclear cells (BM-MNC) (Patel et al. Citation2010). However, the best cell type and optimal time/route for transplantation need to still be determined so more pharmacological strategies should be determined to optimize the therapeutic value of cell transplantation (Wang and Sjoquist Citation2006). Inflammatory responses and cytokine elaboration play integral rules in MI re-modeling. These events include mechanical deformation, ischemic stimulus, reactive oxygen species (ROS) formation and cytokine self-amplification pathways which contribute to survival or deaths of myocytes, modulation of cardiac contractility, alterations of vascular endothelium and recruitment of additional circulating cells of inflammation to the injured myocardium (Kocher et al. Citation2001).
Isoproterenol (ISO), a β-adrenoceptor agonist, has been found to produce MI in large doses; after auto-oxidation, ISO generates highly cytotoxic free radicals known to stimulate the peroxidation of membrane phospholipids, causing severe damage to myocardial membranes. Hence, it is widely used as a model to produce MI in rats (Nian et al. Citation2004; Panda and Naik Citation2009). Therefore, using a rat model of ISO-induced MI and based on the beneficial effects of statins, this study sought to investigate the effect of a combined treatment of pravastatin (PRAV) with BM-MNC in vivo to augment cardiac performance and modulate inflammatory responses.
Materials and methods
Animals
Wistar rats (male, 8-weeks-of-age, 150–200 g) were purchased from the animal facilities of the National Cancer Institute (NRC, Cairo, Egypt). All rats were housed in plastic cages under specific pathogen-free conditions with a 12-h light:dark cycle, constant temperature (25 ± 2 °C) and relative humidity (55 ± 5%), in the Animal House at Tanta University. All rats had ad libitum access to filtered water and standard pellet diet. Rats were acclimated for 1 week prior to the experiment. The Ethics Committee on Animal Research at the Faculty of Pharmacy, Tanta University approved all aspects of this study.
For the experiments, rats were randomly allocated into five groups (n = 10/group). In all cases, a period of 1 week was allowed to elapse between randomization and this would allow time for each rat to adapt to new cage mates and re-establish relationships and hierarchy and thereby reduce stresses to the animals that could potentially have an impact on the study outcomes. The groups were designated: (1) Control (vehicle only), in which rats were to receive two subcutaneous (SC) injections of saline (ISO vehicle) in a volume of 10 μl and only normal saline per os daily (100 μl/dosing) in the 28-day “post-MI induction” period; (2) ISO only, in which rats were to be given ISO (250 mg/kg, SC; Sigma, St. Louis, MO) dissolved in saline once daily for 2 successive days to induce MI (dose was chosen based on Kannan and Quine Citation2013) and then only normal saline in the post-MI induction period; (3) BM-MNC group, in which rats were to be given an IV injection of 14 × 106 newly-isolated BM-MNC in 10 μl PBS via the lateral tail vein on Day 1, 24 h after induction of MI by ISO and then normal saline per os daily in the 28-day “post-MI induction” period; (4) PRAV group, in which rats were to be given PRAV (20 mg/kg) per os daily in the 28-day post-MI induction period (dose was selected based on Kassan et al., Citation2009) on Day 1, 24 h after induction of MI; and (5) PRAV + BM-MNC group, in which rats were to be given both the IV injection of BM-MNC and daily oral PRAV as described above. As this study was meant to evaluate the effects of the drug ± BM-MNC on MI-related outcomes, there were no formal control groups assessed that solely measured effects of PRAV, BM-MNC or the two regimens in combination on the endpoints outlined below.
Monitoring of induction of MI
During and after the ISO injections, rats were housed in cages under close monitoring and then impaired cardiac function was verified by measuring serum Troponin I (TnI) level on Day 2. Specifically, blood samples were collected from the retro-orbital plexus 24 h after the second ISO injection from all rats, serum was isolated and the materials analyzed using a Tn-I ELISA kit (Life Diagnostics, West Chester, PA). Only rats with notable increases in serum TnI level were utilized for further study; in all cases, extra rats were initially enrolled in each group to assure that a minimum of 10 MI-positive rats could then be used in the treatment protocols.
Collection of BM-MNC
Bone marrow cells were collected from the femurs and tibias of naïve adult male rats and BM-MNC subsequently isolated by density gradient centrifugation. Cells were suspended in PBS and cell number/viability evaluated using trypan blue stain and a biomedical microscope. Thereafter, the cells were diluted with PBS to a density of 14 × 108 cells/ml for use in injections.
Blood sampling and processing of the heart
At the end of the study protocol (24 h after the final treatment, Day 29), rats were euthanized by ether asphyxia. At necropsy, a 5 ml blood sample was drawn from the heart for use in biochemical analysis. Thirty minutes after collection, the blood samples were processed by centrifugation (2000 rpm, 15 min, 4 °C), the serum were isolated and aliquots of the sera stored at −80 °C until use in various assays. Thereafter, each heart was removed, trimmed of connecting tissue and rinsed with ice-cold PBS before being cut (vertically) into two equal halves. One half was immediately placed at −80 °C, whereas the other was fixed in 10% phosphate-buffered paraformaldehyde solution (pH 7.4) for 18 h before being embedded with paraffin (for histopathology).
Determination of serum cardiac enzymes
Blood samples collected from the rats were assessed for serum brain natriuretic peptide (BNP) levels using an ELISA kit (Shionoria, Osaka, Japan). The level of sensitivity of the kit was 19 pg BNP/ml. All measures of absorbance were made in an automated plate reader (Europe S.A., Brussels, Belgium).
Determination of cardiac MDA, SOD and NO levels
Each frozen cardiac sample (0.2 g) was homogenized using a Teflon homogenizer (Glas Col, Vernon Hills, CA). Homogenization was carried out in 10 ml of ice-cold potassium chloride solution (1.15%, w/v). After disruption, the sample was centrifuged at 3000 rpm for 15 min at 4 °C and the resultant supernatant collected. Lipid peroxidation in the homogenates was estimated by determination of thiobarbituric acid-reactive substance (TBARS) content – reflective of malondialdehyde (MDA) end-product of membrane oxidation – using a commercial kit (Biodiagnostic, Giza, Egypt). Cardiac superoxide dismutase (SOD) as well as nitrite (reflecting NO) contents were also determined using commercial kits (Biodiagnostic).
Determination of cardiac TNFα, IL1β and VEGF levels
The tissue content of TNFα, IL-1β and VEGF were determined in samples of the above homogenates using ELISA kits (Ray Biotech, Inc., Norcross, GA), according to manufacturer protocols. Kit levels of sensitivity were 4 pg TNFα/ml, 80 pg IL-1β/ml and 1 pg VEGF/ml.
Histopathological examination of the heart tissues
Left ventricles were taken from the heart of rats from different groups and fixed in 10% buffered formalin solution prepared in normal (0.9%) saline (pH 7.4) for 24 h. Washing was done with tap water before serial dilutions of alcohol were applied for dehydration. Specimens were then cleared in xylene, embedded in paraffin for 24 h and then sections prepared at 4-μm using a microtome. Sections were then de-paraffinized and stained with H&E to permit histopathologic examination (in a blinded manner) using a Olympus BX 51 light microscope (Olympus America, Melville, NY) and photographed with an Olympus DP11 digital camera connected to the microscope. Observations were recorded from representative photomicrographs taken at 400× magnification.
Immunohistochemical staining for caspase 3 and image analysis
After de-paraffinization and rehydration, random slides of heart tissues from each rat were treated with 3% H2O2 (in PBS) for 10 min to remove nascent peroxidase activities and then with 5% naïve rat sera (in PBS) for 20 min. Sections were then incubated overnight at 4 °C with polyclonal rabbit anti-active caspase 3 (1:500 dilution in manufacturer-provided buffer; PharMingen, San Diego, CA). After washing gently with PBS, the slides were then incubated for 20 min with biotinylated goat anti-rabbit IgG (1:2000 dilution in manufacturer-provided buffer; Vector Laboratories, Burlingame, CA). After an additional rinsing with PBS, the slides were incubated with horseradish peroxidase (HRP)-streptavidin solution (Vector Laboratories) for 20 min. Antibody binding sites were visualized through reaction with diaminobenzoate (DAB)-H2O2 solution (Sigma, St. Louis, MO) before the sections were counterstained with 0.5% methyl green. Using a light microscope, Caspase 3+ cells were enumerated in 10 different microscopic fields/slide of at least three different sections of heart from each rat. The percentage of apoptotic cells from all cells evaluated was termed the apoptotic index.
Statistical analysis
All data were expressed as mean (± SD). Comparisons between means of different groups were carried out using a one-way analysis of variance (ANOVA). For comparison between means of two groups, a Student’s t-test was employed. The Minitab statistical software package (v.17, Minitab Inc., State College, PA) was used to carry out all analyses. Statistical significance was accepted at p < 0.05.
Results
Serum TnI and BNP levels
Fifty of sixty male rats survived the subcutaneous administration of ISO (250 mg/kg/day, for 2 days). ISO-treated rats showed a significant increase in serum TnI level compared to Control rats that were injected with saline only (p < 0.01) (). After the first dose of ISO, 10% of rats died, this percentage increased to 15% after the second dose of ISO. Treatment with oral PRAV (20 mg/kg) for 4 weeks, BM-MNC (14 × 106 cell) bolus dose or a combination of the two significantly modified the number of surviving rats compared to those in the ISO only group (p < 0.05) (data not shown).
Figure 1. Effect of ISO on serum levels of TnI. Rats were injected with ISO (250 mg/kg/day, subcutaneous) for 2 days to induce AMI. A significant increase in serum TnI level in ISO treated rats (p < 0.01) from Control rats. Results are expressed as mean (± SD; n = 10 control and 50 ISO).
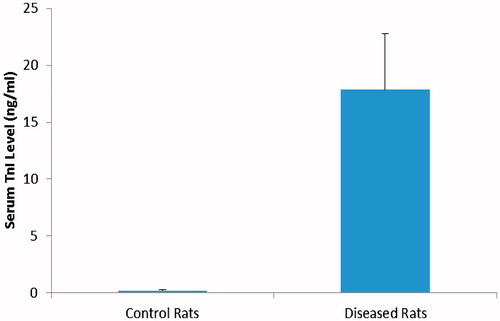
At 4 weeks after treatment, ISO-only rats showed a significant increase in serum BNP level (p < 0.01) vs levels in control rats (). Treatment with PRAV in combination with BM-MNC significantly decreased serum BNP levels by 47.2% (p < 0.05). Administration of PRAV or BM-MNC alone did not modify serum BNP levels, with reductions vs levels in ISO-only rats of just 28.8 and 5.0%, respectively.
Figure 2. Levels of serum BNP. Rats were injected twice with ISO to induce AMI. Oral PRAV for 4 weeks in combination with BM-MNC significantly decreased the serum BNP level in infarcted rats (p < 0.05). Results are expressed as mean (± SD; n = 10/group). *Value significantly different from Control rats; afrom ISO; bfrom BM-MNC; and cfrom PRAV-treated rats (all p < 0.05).
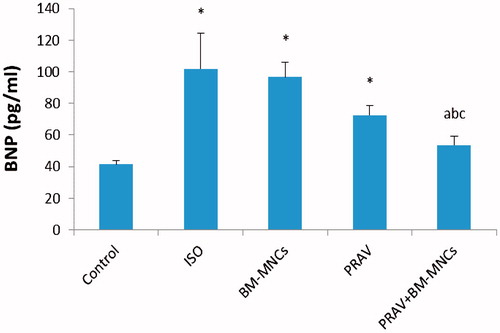
PRAV, BM-MNC and combination effects on cardiac NO, MDA, SOD, TNFα, IL1β and VEGF
Rats in the ISO only group had a significant increase in cardiac MDA levels (125.7%) and parallel decreases in SOD and NO content (81.0 and 56.2%, respectively) as compared to values in the tissues from the Control group rats (). Treatment with PRAV significantly decreased MDA cardiac content (31.6%) and significantly increased SOD and NO content (132.4 and 55.1%, respectively) compared to in the ISO only group. Treatment with BM-MNC significantly decreased MDA cardiac content (44.9%) and significantly increased SOD cardiac content (70.9%), but did not modify NO cardiac content compared to values for the ISO only rats. Treatment with PRAV + BM-MNC resulted in a significant decrease in MDA cardiac content (50.5%) and an increase in SOD and NO cardiac content (166.2 and 76.7%, respectively), as compared to tissues from the ISO only rats.
Figure 3. Effect of treatment with oral PRAV, BM-MNC or a combination on levels of MDA, SOD and NO in homogenates of cardiac tissue 4 weeks post-induction of MI in rats. (a–c) Results expressed as mean (± SD; n = 10/group). *Value significantly different from Control rats; afrom ISO; bfrom BM-MNC; and cfrom PRAV-treated rats (all p < 0.05).
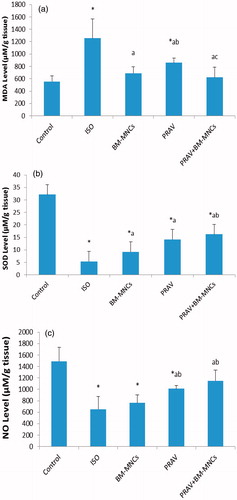
Cardiac content of TNFα, IL1β and VEGF () increased significantly in rats injected with ISO (664.4, 345.0 and 444.0%, respectively) compared to levels in tissues from rats in the Control group. Treatment of rats with PRAV produced a significant decrease in cardiac content of TNFα, IL1β and VEGF (65.5, 69.0, and 57.0%, respectively) as compared to in the ISO group. Treatment with BM-MNC significantly decreased cardiac content of TNFα (48.1%), but did not modify IL-1β or VEGF cardiac content as compared to levels in tissues from the ISO only rats. Treatment with PRAV combined with BM-MNC produced a significant decrease in cardiac content of TNFα, IL1β and VEGF (87.0, 78.3 and 72.7%, respectively) as compared to the ISO only rat tissues.
Figure 4. Effect of treatment with oral PRAV, BM-MNC or a combination on levels of TNFα, IL-1β and VEGF in homogenates of cardiac tissue 4 weeks post-induction of MI in rats (a–c). Results expressed as mean (± SD; n = 10/group). *Value significantly different from Control rats; afrom ISO; bfrom BM-MNC; and cfrom PRAV-treated rats (all p < 0.05).
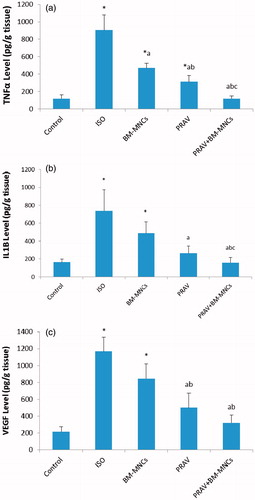
PRAV, BM-MNC and combination effects on histopathologic changes associated with MI
The histopathologic status of the isolated heart tissues was examined (). In the Control group, the myocardial fibers were arranged regularly with clear striations and no apparent degeneration or necrosis was observed. Histological sections of the ISO only rats showed multiple focal areas of myocardial cell degeneration with edema and inflammatory cells infiltration. A reversal of these inflammatory responses was noticed in rats treated with PRAV. On the other hand, treatment with BM-MNC or its combination with PRAV resulted in edematous myocardial stroma with minimal inflammatory infiltration and marked improvement of heart tissues as compared to the tissues from the ISO only group.
Figure 5. Histology of heart sections recovered from rats. (a) Control rat showing normal myocardial syncytial muscle fibers with normal arrangement, nuclei and vasculature of the heart. (b) ISO only rat showing early infarction, widely-dilated engorged blood vessels with areas of extravasation and inflammatory cells infiltration among apparently normal myocardial syncytial muscle fibers. (c) BM-MNC-treated rat showing infiltration with mononuclear (bone marrow) cells. (d) PRAV-treated rat showing apparently normal myocardial muscle fibers, dilated blood vessels and edematous stroma with minimum inflammatory infiltration. (e) PRAV + BM-MNC-treated rat showing bone marrow cells in edematous myocardial stroma with minimal inflammatory infiltration (as compared to the ISO only rats). H&E stain. Magnification = 400×. Representative sections are shown.
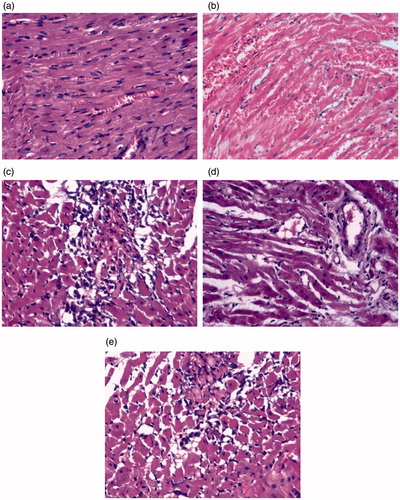
Immunohistochemistry and image analysis
Immunohistochemical staining for caspase 3, an important indicator of apoptotic cell death, was also performed on the isolated heart tissues (). The analyses revealed that, as compared with levels in control rat hearts, in hearts of infarcted rats there was an increase in caspase 3 immunoreactivity. Far less caspase-3 activity was noted in the tissues obtained from the PRAV-, PRAV + BM-MNC- or BM-MNC-treated rats.
Figure 6. Caspase-3 DAPI staining for apoptotic cells in heart tissues. (a) Section from Control rat showing positive staining for caspase 3. (b) ISO only rat tissue showing focal deeply-stained muscle fibers (heavily positive) for caspase 3; extravasated blood appears at periphery of section. (c) BM-MNC-treated; (d) PRAV-treated; and (e) PRAV + BM-MNC-treated rat tissue.
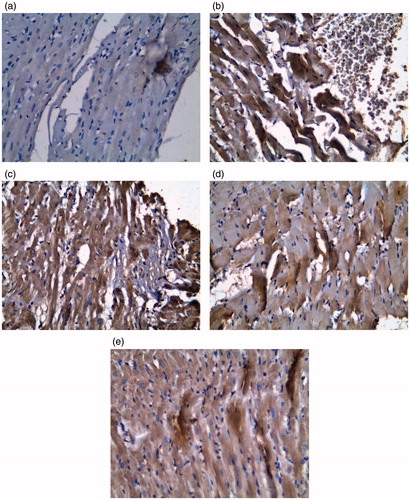
The apoptotic index of the infarcted region in the ISO only rats was increased significantly (p < 0.05) compared with the value for the Control rats (). In contrast, the index in the tissues from the PRAV or PRAV + BM-MNC rats was significantly lower than that in the ISO only hosts (p < 0.05), but there was no difference between outcomes for the PRAV alone vs PRAV + BM-MNC groups. BM-MNC rats had index values that were comparable to that of the ISO only hosts.
Figure 7. Apoptotic indices. Results are expressed as mean ± SEM (n = 10/group). Quantification shows that apoptotic myocytes were significantly reduced in the PRAV group vs ISO only group (*p < 0.05) and in the PRAV + BM-MNC group vs ISO only group (**p < 0.01). There was no significant difference between PRAV and PRAV + BM-MNC group outcomes.
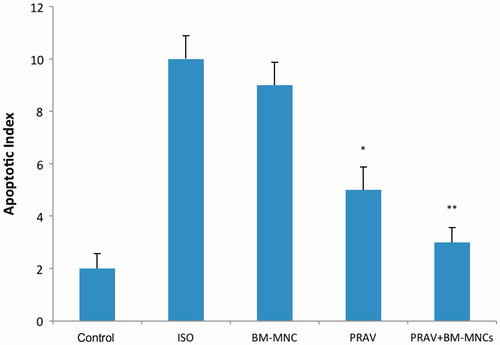
Discussion
The current study demonstrated the pharmacological enhancement of cell therapy with BM-MNC during PRAV treatment in experimentally-induced AMI in rats. Here, acute injection with ISO in a dose of 250 mg/kg for 2 days induced myocardial injury accompanied by elevated total serum TnI levels within 24 h of the final dose of ISO, in accordance with the findings of Lobo Filho et al. (Citation2011). It has been reported that ISO administration in high doses to animals produces infarction in the heart similar to MI in humans (Vennila and Pugalendi Citation2010). Mechanisms proposed to explain ISO induced cardiac damage are varied and include generation of highly cytotoxic free radicals, increased calcium overload and mitochondrial injury or dysfunction (Rathore et al. Citation1998). ISO-generated free radicals are known to initiate peroxidation of membrane-bound polyunsaturated fatty acids, leading to damage of the structural/functional integrity of the myocardium, with consequent changes in membrane permeability (Rathore et al. Citation1998).
The present study showed that a combined treatment of BM-MNC and PRAV, compared with treatment with PRAV or BM-MNC alone, resulted in a greater improvement in cardiac function in terms of decreases in serum BNP level and by a decrease in the degree of infiltration of inflammatory cells (along with decreased myocyte degeneration) and decreased cardiac cell death. However, these results were comparable to those obtained with statins; for example, atrovastatin alone produced a significant decrease in N-terminal pro-hormone brain natriuretic peptide level in a previous study (Stypmann et al. Citation2008).
It is worth noting that, unlike markers of myocardial necrosis, BNP is also useful for estimating the effectiveness of treatment (Maeda et al. Citation2000). In addition, BNP is not considered released from the necrotizing myocardium (Grybauskiene et al. Citation2007). Experimental studies have shown that the level of BNP secretion and BNP mRNA expression are high mainly in the boundary region between the infarcted and non-infarcted regions (Hama et al. Citation1995) that is stimulated by the increased wall stress that directly related to the infarction. Also it was found that BNP level following MI appears to be associated not only with left ventricle ejection fraction (LV-EF) deterioration, but also with the extent of myocardial injury as assessed by cardiac markers, supporting the opinion that elevation of BNP is a general indicator of reduced cardiac performance (Grybauskiene et al. Citation2007). In a previous study, high plasma BNP levels 3 months after optimized treatment for HF are risk factors for morbidity and mortality of patients with HF; moreover, plasma BNP levels 3 months after optimized treatment are more useful predictors than the levels of LV-EF (Maeda et al. Citation2000). Another study showed a strong relation between N-BNP, LV function and cardiovascular prognosis has been observed (Hama et al. Citation1995).
Thus, in the present study, the increase in cardiac MDA content (an indicator for lipid peroxidation) and decrease in cardiac SOD content in the ISO group could be expected. The current results are in line with the work of other investigators (Panda and Naik Citation2009; Gayathri et al. Citation2011; Kannan and Quine Citation2013). Reactive oxygen species (ROS) participate in cardiomyocyte apoptosis and inflammation, especially in AMI (Sun Citation2007). After AMI, ROS can cause nuclear factor-κB activation that stimulates gene expression of pro-inflammatory cytokines (including TNFα and interleukins) and initiates inflammatory reactions (Kabe et al. Citation2005). Several studies have shown that anti-oxidant treatment reduces infarct size in the rat AMI model (Hung et al. Citation2002; Sahna et al. Citation2002). The acute release of large amounts of ROS is directly related to cell death associated with AMI, whereas the chronic release of ROS may be associated with the development of left ventricular hypertrophy and HF (Sorescu and Griendling Citation2002; Shiomi et al. Citation2004). Anti-oxidant treatment appears to reduce cardiac fibrosis (Sia et al. Citation2002). The present data indicate that either administration of PRAV or BM-MNC alone or in combination may inhibit the oxidative stress supported by the decrease in levels of MDA and increasing the levels of SOD in the infarcted myocardium.
Cytokine expression is markedly up-regulated in healing AMI. The present data clearly showed a significant increase in cardiac TNFα and IL-1β in the ISO only-treated rats. This finding agrees with that of Hoch and Leach (Citation2015), who demonstrated marked elevations of various inflammatory mediators (as interleukins and TNFα) in cases of AMI and congestive heart failure. The source of TNFα following the myocardial ischemia is mainly from cardiac mast cell degranulation (Frangogiannis et al. Citation2002). Subsequently, the increased presence of TNFα results in increased risk of induction of apoptosis of cardiac myocytes and exerts negative inotropic effects – at least potentially – through an increased production of sphingosine (Das Citation2000). TNFα also induces cardiac myocyte expression of IL-6, which may have subsequent deleterious effects on myocyte function (Krishnaswamy et al. Citation1998). Furthermore, TNFα induces production of free radicals that, in turn, can further damage the myocardium (Das Citation2000). The present data showed that the administration of PRAV significantly decreased cardiac levels of inflammatory IL-1β and TNFα; such outcomes support an anti-inflammatory effect for PRAV post-infarction. In addition, treatment with PRAV in combination with BM-MNC significantly decreased TNFα and IL-1β in comparison with PRAV or BM-MNC alone, while treatment with BM-MNC alone did not modify IL-1β cardiac levels. These outcomes were in keeping with those of other studies that have reported on the anti-inflammatory effects of statins (Kureishi et al. Citation2000; Vasa et al. Citation2001).
The current results demonstrated that the level of NO increased in the PRAV-treated groups (including PRAV and PRAV + BM-MNC). Previous studies demonstrated the ability of statins to up-regulate eNOS activity and NO production by increasing eNOS mRNA stability (Kaesemeyer et al. Citation1999). Endothelial NOS plays a central role in maintaining cardiovascular homeostasis by controlling NO bioactivity. A previous study showed that simvastatin and lovastatin up-regulate eNOS expression almost 4-fold, which was reversed by the addition of mevalonate (Laufs et al. Citation1998). Another study showed that eNOS over-expression improved the post-ischemic neovascularization response in healthy, diabetic and atherosclerotic mice (Mees et al. Citation2011).
Previous studies have found that statins improve angiogenesis (Kureishi et al. Citation2000; Llevadot et al. Citation2001; Vasa et al. Citation2001; Assmus et al. Citation2003; Landmesser et al. Citation2004), but have no effect on coronary collateral flow in patients with coronary artery disease (Zbinden Citation2004). Conversely, in accordance with the present findings, PRAV decreased the production of VEGF and inhibited inflammation-induced angiogenesis. Also we found that combined treatment of PRAV and BM-MNC significantly decrease the level of VEGF, which indicates that the combination did not improve angiogenesis in infarcted rats.
Overall, the current study provided evidence that treatment with PRAV and BM-MNC together showed marked improvement in cardiac function reflected by a significant decrease in serum BNP level compared with PRAV or BM-MNC alone, coupled by a significant decrease of inflammatory markers. Histopathologic examination and caspase-3 staining of cardiac tissues correlated with the observed biochemical changes. Such effects can be explained by previously reported anti-inflammatory potentials of both pravastatin and BM-MNC that were more apparent upon their combination together.
Conclusion
The present study revealed that BM-MNC therapy potentiates the efficacy of PRAV treatment, possibly via decreasing inflammatory reactions as well as decreased tissue damage in ISO induced MI in rats. Further experimental and clinical studies may be needed to verify the enhancing effect of BM-MNC on PRAV treatment. The mechanisms underlying these effects will also be of interest in future studies.
Acknowledgments
The authors acknowledge the support of the Faculty of Pharmacy, Tanta University to perform this research.
Disclosure statement
The authors report no conflicts of interest. The authors alone are responsible for the content and writing of the paper.
References
- Assmus B, Urbich C, Aicher A, Hofmann WK, Haendeler J, Rössig L, Spyridopoulos I, Zeiher AM, Dimmeler S. 2003. HMG-CoA reductase inhibitors reduce senescence and increase proliferation of endothelial progenitor cells via regulation of cell cycle regulatory genes. Circ Res. 92:1049–1055.
- Das U. 2000. Free radicals, cytokines and nitric oxide in cardiac failure and myocardial infarction. Mol Cell Biochem. 215:145–152.
- Frangogiannis N, Smith CW, Entman M. 2002. Inflammatory response in myocardial infarction. Cardiovasc Res. 53:31–47.
- Gayathri V, Ananthi S, Chandronitha C, Ramakrishnan G, Lakshmisundaram R, Vasanthi H. 2011. Cardioprotective effect of Nerium oleander flower against isoproterenol-induced myocardial oxidative stress in experimental rats. J Cardiovasc Pharmacol Ther. 16:96–104.
- Grybauskiene R, Karciauskaite D, Brazdzionyte J, Janenaite J, Bertasiene Z, Grybauskas P. 2007. Brain natriuretic peptide and other cardiac markers predicting left ventricular remodeling and function two years after myocardial infarction. Medicina (Kaunas). 43:708–715.
- Hama N, Itoh H, Shirakami G, Nakagawa O, Suga S, Ogawa Y, Masuda I, Nakanishi K, Yoshimasa T, Hashimoto Y. 1995. Rapid ventricular induction of brain natriuretic peptide gene expression in experimental acute myocardial infarction. Circulation. 92:1558–1564.
- Hamelin B, Turgeon J. 1998. Hydrophilicity/lipophilicity: Relevance for pharmacology and clinical effects of HMG-CoA reductase inhibitors. Trends Pharmacol Sci. 19:26–37.
- Hoch A, Leach J. 2015. Concise review: Optimizing expansion of bone marrow mesenchymal stem/stromal cells for clinical applications. Stem Cells Transl Med. 4:412.
- Hung L, Su M, Chu W, Chiao CW, Chan WF, Chen JK. 2002. Protective effect of resveratrols on ischaemia-reperfusion injuries of rat hearts is correlated with anti-oxidant efficacy. Br J Pharmacol. 135:1627–1633.
- Kabe Y, Ando K, Hirao S, Yoshida M, Handa H. 2005. Redox regulation of NF-κB activation: Distinct redox regulation between the cytoplasm and the nucleus. Antioxid Redox Signal. 7:395–403.
- Kaesemeyer W, Caldwell R, Huang J, Caldwell R. 1999. Pravastatin sodium activates endothelial nitric oxide synthase independent of its cholesterol-lowering actions. J Am Coll Cardiol. 33:234–241.
- Kannan M, Quine S. 2013. Ellagic acid inhibits cardiac arrhythmias, hypertrophy, and hyperlipidaemia during myocardial infarction in rats. Metabolism. 62:52–61.
- Kassan M, Montero M, Sevilla M. 2009. Chronic treatment with pravastatin prevents early cardiovascular changes in spontaneously hypertensive rats. Br J Pharmacol. 158:541–547.
- Kocher A, Schuster M, Szabolcs M, Takuma S, Burkhoff D, Wang J, Homma S, Edwards NM, Itescu S. 2001. Neovascularization of ischemic myocardium by human bone-marrow-derived angioblasts prevents cardiomyocyte apoptosis, reduces remodeling, and improves cardiac function. Nat Med. 7:430–436.
- Krishnaswamy G, Smith J, Mukkamala R, Hall K, Joyner W, Yerra L, Chi DS. 1998. Multifunctional cytokine expression by human coronary endothelium and regulation by monokines and glucocorticoids. Microvasc Res. 55:189–200.
- Kureishi Y, Luo Z, Shiojima I, Bialik A, Fulton D, Lefer DJ, Sessa WC, Walsh K. 2000. HMG-CoA reductase inhibitor simvastatin activates protein kinase Akt and promotes angiogenesis in normo-cholesterolemic animals. Nat Med. 6:1004–1010.
- Landmesser U, Engberding N, Bahlmann F, Schaefer A, Wiencke A, Heineke A, Spiekermann S, Hilfiker-Kleiner D, Templin C, Kotlarz D, et al. 2004. Statin-induced improvement of endothelial progenitor cell mobilization, myocardial neovascularization, left ventricular function, and survival after experimental myocardial infarction requires endothelial nitric oxide synthase. Circulation. 110:1933–1939.
- Laufs U, La Fata V, Plutzky J, Liao J. 1998. Up-regulation of endothelial nitric oxide synthase by HMG-CoA reductase inhibitors. Circulation. 97:1129–1135.
- Liu M, Wang F, Wang Y, Jin R. 2009. Atorvastatin improves endothelial function and cardiac performance in patients with dilated cardiomyopathy: The role of inflammation. Cardiovasc Drugs Ther. 23:369–376.
- Llevadot J, Murasawa S, Kureishi Y, Uchida S, Masuda H, Kawamoto A, Walsh K, Isner JM, Asahara T. 2001. HMG-CoA reductase inhibitor mobilizes bone marrow–derived endothelial progenitor cells. J Clin Invest. 108:399–405.
- Lobo Filho H, Ferreira N, Sousa RB, Carvalho ER, Lobo PL, Lobo Filho JG. 2011. Experimental model of myocardial infarction induced by isoproterenol in rats. Rev Bras Cir Cardiovasc. 26:469–476.
- Lopez A, Murray C. 1998. The global burden of disease, 1990-2020. Nat Med. 4:1241–1243.
- Maeda K, Tsutamoto T, Wada A, Mabuchi N, Hayashi M, Tsutsui T, Ohnishi M, Sawaki M, Fujii M, Matsumoto T. 2000. High levels of plasma brain natriuretic peptide and IL-6 after optimized treatment for heart failure are independent risk factors for morbidity and mortality in patients with congestive heart failure. J Am Coll Cardiol. 36:1587–1593.
- Mees B, Recalde A, Loinard C, Tempel D, Godinho M, Vilar J, van Haperen R, Lévy B, de Crom R, Silvestre JS. 2011. Endothelial nitric oxide synthase over-expression restores efficiency of bone marrow mononuclear cell-based therapy. Am J Pathol. 178:55–60.
- Nian M, Lee P, Khaper N, Liu P. 2004. Inflammatory cytokines and post-myocardial infarction remodeling. Circ Res. 94:1543–1553.
- Panda V, Naik S. 2009. Evaluation of cardioprotective activity of Ginkgo biloba and Ocimum sanctum in rodents. Alt Med Rev. 14:161–171.
- Patel V, Upaganlawar A, Zalawadia R, Balaraman R. 2010. Cardioprotective effect of melatonin against isoproterenol induced myocardial infarction in rats: A biochemical, electrocardiographic, and histoarchitectural evaluation. Eur J Pharmacol. 644:160–168.
- Rathore N, John S, Kale M, Bhatnagar D. 1998. Lipid peroxidation and anti-oxidant enzymes in isoproterenol induced oxidative stress in rat tissues. Pharmacol Res. 38:297–303.
- Ridker P, Rifai N, Pfeffer M, Sacks FM, Moye LA, Goldman S, Flaker GC, Braunwald E. 1998. Inflammation, pravastatin, and the risk of coronary events after myocardial infarction in patients with average cholesterol levels. Circulation. 98:839–844.
- Sahna E, Acet A, Ozer M, Olmez E. 2002. Myocardial ischemia-reperfusion in rats: Reduction of infarct size by either supplemental physiological or pharmacological doses of melatonin. J Pineal Res. 33:234–238.
- Shiomi T, Tsutsui H, Matsusaka H, Murakami K, Hayashidani S, Ikeuchi M, Wen J, Kubota T, Utsumi H, Takeshita A. 2004. Over-expression of glutathione peroxidase prevents left ventricular remodeling and failure after myocardial infarction in mice. Circulation. 109:544–549.
- Sia Y, Parker T, Liu P, Tsoporis JN, Adam A, Rouleau JL. 2002. Improved post-myocardial infarction survival with probucol in rats: Effects on left ventricular function, morphology, cardiac oxidative stress and cytokine expression. J Am Coll Cardiol. 39:148–156.
- Sorescu D, Griendling K. 2002. Reactive oxygen species, mitochondria, and NAD(P)H oxidases in the development and progression of heart failure. Congest Heart Fail. 8:132–140.
- Stypmann J, Schubert A, Welp H, Schulte H, Assmann G, Breithardt G, Nofer JR. 2008. Atorvastatin therapy is associated with reduced levels of N-terminal pro-hormone brain natriuretic peptide and improved cardiac function in patients with heart failure. Clin Cardiol. 31:478–481.
- Sun Y. 2007. Oxidative stress and cardiac repair/remodeling following infarction. Am J Med Sci. 334:197–205.
- Vasa M, Fichtlscherer S, Adler K, Aicher A, Martin H, Zeiher AM, Dimmeler S. 2001. Increase in circulating endothelial progenitor cells by statin therapy in patients with stable coronary artery disease. Circulation. 103:2885–2890.
- Vennila L, Pugalendi K. 2010. Protective effect of sesamol against myocardial infarction caused by isoproterenol in Wistar rats. Redox Reports. 15:36–42.
- Wang Q, Sjoquist P. 2006. Myocardial regeneration with stem cells: Pharmacological possibilities for efficacy enhancement. Pharmacol Res. 53:331–340.
- Zbinden S. 2004. Effect of statin treatment on coronary collateral flow in patients with coronary artery disease. Heart. 90:448–449.