Abstract
Tetrabromobisphenol A (TBBPA) and hexabromocyclododecane (HBCD), flame retardant compounds used in epoxy resin circuit boards and upholstery, contaminate the environment and are found in human serum. Lymphocytes and monocytes are immune cells that, among other functions, secrete pro-inflammatory cytokines such as interleukin (IL)-1β, an important regulator of immune responsiveness and tissue growth and repair. Thus, if its levels are dysregulated, loss of proper immune function and increased invasiveness of tumors could ensue. This study examines whether exposures to varying concentrations (0.05–5.0 μM) of TBBPA and HBCD for 24 h, 48 h and 6 days interfere with the ability of immune cells to secrete IL-1β. The immune cell preparations examined were human natural killer (NK) cells, monocyte-depleted (MD) peripheral blood mononuclear cells (MD-PBMC) and PBMC. Both increased and decreased secretion of IL-1β from all three types of cell preparation were seen with TBBPA exposures and were dependent on concentration and length of exposure. TBBPA induced changes varied considerably from donor to donor. Exposure to HBCD from 0.5–5.0 μM caused increases in IL-1β secretion after all lengths of exposures in all cell preparations. The specific HBCD levels at which increases occurred varied among donors. Examinations of the signaling pathway(s) responsible for the elevated secretion of IL-1β after HBCD exposure were carried out in MD-PBMC cells. Results revealed that MAPK pathways (ERK1/2 and p38) appear to be the targets of HBCD that lead to increased IL-1β secretion from immune cells.
Introduction
The widespread industrial application of flame retardants such as tetrabromobisphenol A (TBBPA) and hexabromocyclododecanes (HBCD) has led to environmental contamination by these compounds. TBBPA is a brominated phenolic compound that has been utilized as a flame retardant in plastic, textile and paper industries (IPCS/WHO Citation1995; HSDB Citation2001). In several studies from Japan, France and Norway, TBBPA has been detected in human serum samples. A Japanese study showed serum levels of TBBPA at an average of 4.5 ng/g lipid (≈0.034 nM) (Nagayama et al. Citation2001), while a Norwegian study found serum levels of 0.24–0.71 ng/g lipid (≈0.0018–0.0053 nM) (Thomsen et al. Citation2002). Maternal serum showed average levels of 154 pg/g (0.283 nM), while cord blood had levels of 199 pg/g (0.366 nM) in a French study (Cariou et al. Citation2008). Human exposure of TBBPA is due to ingestion of contaminated foods, dust inhalation from households, schoolrooms and automobiles and dermal contact (Peterman et al. Citation2000; EFSA Citation2011). Toxicological experiments revealed that TBBPA could act as an endocrine disruptor by interacting with the thyroid hormone system through strong competitive binding to transthyretin (Meerts et al. Citation2000; Legler and Brouwer Citation2003).
Hexabromocyclododecanes (HBCD) are aliphatic brominated cyclic alkanes synthesized by the bromination of cyclododecatriene (Mack Citation2004). HBCD is generally used as additive flame retardant in polystyrene foam for thermal insulation in buildings, furniture fabrics and electrical apparatus (Birnbaun and Staskal, 2004; Covaci et al. Citation2006). HBCD was detected in fish, mussels, zooplankton, as well as in marine animals and several species of bird eggs, indicating accumulation/biomagnification in the food chain (Law et al. Citation2005; Bustnes et al. Citation2007; Polder et al. Citation2008). Toxicology experiments revealed that HBCD is an endocrine disruptor, developmental neurotoxicant, and causes alterations in the rat thyroid system (Palace et al. Citation2010; Saegusa et al. Citation2009). HBCD was found in human serum at 100 pg/g serum, which is ≈0.16 nM (Schecter et al. Citation2012). In Norwegian workers at an industrial plant that makes HBCD-treated polystyrene, serum levels of HBCD were as high as 856 ng/g of lipid (5 nM) (Thomsen et al. Citation2007).
Reduction in lytic activity of human natural killer (NK) cells has been detected after exposure to TBBPA and HBCD (Hinkson and Whalen Citation2009; Kibakaya et al. Citation2009). Additionally, TBBPA and HBCD decreased key cell surface proteins and activated MAPKs in NK cells (Hinkson and Whalen Citation2010; Hurd and Whalen Citation2011; Cato et al. Citation2014). Additionally, alterations of interferon (IFN)-γ secretion from increasingly re-constituted immune cell preparations such as NK cells, peripheral blood mononuclear cells (PMBC) and monocyte-depleted (MD) peripheral blood mononuclear cells (MD-PBMC) were seen after exposure to TBBPA and HBCD (Almughamsi and Whalen Citation2015).
Interleukin (IL)-1β is a pleiotropic pro-inflammatory cytokine encoded by the IL-1β gene (Balkwill and Coussens Citation2004). Increased production of IL-1β has been found in human cancer cells, including acute myelogenous leukemias, melanomas and multiple myeloma (Dinarello Citation1996; Jin et al. Citation1997; Arlt et al. Citation2002; Elaraj et al. Citation2006; Lewis and Varghese Citation2006; Muerkoster et al. Citation2006). IL-1β regulates expression of several pro-metastatic genes, including matrix metallo-proteinases, endothelial adhesion molecules, vascular endothelial cell growth factor (VEGF), as well as other growth factors (Dinarello Citation2010). IL-1β is the major cytokine during angiogenesis of multiple myeloma (Bar et al. Citation2004). IL-1β also advances tissue damage during pathogenesis of chronic inflammatory diseases (Apte et al. Citation2006) and expedites tumor invasiveness. Chronic inflammation also has a vital role in carcinogenesis. By activation of c-Jun N-terminal kinase and p38 mitogen-activated protein kinase (MAPK) pathways, IL-1β induces nitric oxide-mediated apoptosis (Mathias et al. Citation1993; Raingeaud et al. Citation1995). Increased secretion of IL-1β plays an important role in atherosclerosis, Type 2 diabetes and various autoimmune disorders, including rheumatoid arthritis, multiple sclerosis, Crohn’s disease and Alzheimer’s disease (Dinarello Citation1996; Allan et al. Citation2005; Simi et al. Citation2007; Church et al. Citation2008).
In the current study, different types of immune cell preparations, including human NK cells, monocyte-depleted (MD) peripheral blood mononuclear cells (MD-PBMC), and PBMC were used to observe the effects of TBBPA and HBCD on IL-1β secretion. Using increasingly reconstituted immune cell preparations helped us to examine the effect of TBBPA and HBCD on IL-1β secretion in ex vivo systems of various levels of complexity that may be more relevant to physiologic situations. Another objective of this study was to identify the intracellular signaling pathways involved in any flame retardant-induced increases of immune IL-1β secretion. Thus, studies examining MD-PBMC that were exposed to an ERK 1/2 pathway inhibitor (specifically a MEK inhibitor), JNK pathway inhibitor, p38 inhibitor, Caspase I inhibitor II, or an NF-κB inhibitor prior to exposure to flame retardant compounds were carried out.
Materials and methods
Preparation of NK cells
NK cells were prepared from buffy coats (source: leukocytes from healthy adult donors) purchased from Key Biologics LLC (Memphis, TN). Buffy coats (≈45 ml) were treated with 0.6–0.8 ml) RosetteSep human NK cell enrichment antibody cocktail (StemCell Technologies, Vancouver, BC, Canada) and each mixture was incubated for 25 min at room temperature (∼25°C). Then, 7–8 ml of the mixture was layered atop 4 ml Lymphosep lymphocyte separation media (ρ = 1.077 g/ml; MP Biomedicals, Irvine, CA) and centrifuged at 1200 × g for 30–50 min. NK cells were collected, washed twice with phosphate-buffered saline (PBS, pH 7.2), re-suspended in complete media (RPMI-1640 supplemented with 10% heat-inactivated bovine calf serum [BCS]), 2 mM l-glutamine and 50 U penicillin G with 50 μg streptomycin/ml (all from Fisher Scientific, Pittsburgh, PA) at 106 cells/ml and placed in a 5% CO2 atmosphere maintained at 37 °C.
Preparation of PMBC and monocyte-depleted (MD) PBMC
PBMC were isolated from Leukocyte filters (PALL-RC2D) obtained from the Red Cross Blood Bank Facility (Nashville, TN) as described in Meyer et al. (Citation2005). Leukocytes were retrieved from filters by back-flushing with elution medium (PBS containing 5 mM disodium EDTA and 2.5% sucrose [w/v] and then collecting the eluent. Eluent often contained leukocytes with erythrocyte contamination; to rectify this, eluent was layered atop Lymphosep (1.077 g/ml) and centrifuged as above. Granulocytes and erythrocytes pelleted out while PBMC remained atop the Lymphosep. The PBMC were collected and washed (250 × g, 10 min, 20–22°C) with PBS, then re-suspended in complete media. Monocyte-depleted PBMC (10–20% CD16+, 10–20% CD56+, 70–80% CD3+, 3–5% CD19+, 2–20% CD14+) were prepared by incubating aliquots of the isolated PMBC in glass Petri dishes (150 mm) at 37°C for 1 h.
Chemical preparation
TBBPA and HBCD were purchased from Sigma (St. Louis, MO). A stock solution was prepared by dissolving HBCD or HBCD in dimethyl sulfoxide (DMSO). Desired concentrations of either TBBPA or HBCD were then prepared by dilution of the stock in cell culture media.
Inhibitor preparation
Enzyme inhibitors were purchased from Fischer Scientific. Each inhibitor was prepared from stock solution in DMSO. Caspase I inhibitor II, MEK 1/2 inhibitor (PD98059), p38 inhibitor (SB202190), NF-κB inhibitor (BAY11-7085) and JNK inhibitor (JNK × BI78D3) were prepared by dilution of respective stock solutions in cell culture media.
Cell treatments
NK cells, MD-PBMC or PBMC (at concentration of 1.5 × 106 cells/ml) were treated with TBBPA or HBCD (with appropriate control) at concentrations of 0.05–5.0 μM for 24 h, 48 h or 6 days. Following the incubations, cells were pelleted and supernatants collected and frozen at −80°C until assayed for IL-1β. For pathway inhibitor experiments, MD-PBMC (at 1.5 × 106 cells/ml) were treated with enzyme inhibitors 1 h prior to adding the HBCD at concentrations of 0.5, 1.0 or 2.5 μM for 24 h. After these incubations, cells were pelleted and supernatants collected and stored at −80°C until assaying for IL-1β.
Cell viability
Cell viability was assessed at the beginning and end of each exposure period using the trypan blue exclusion method. Briefly, cells were mixed with 0.1% trypan blue solution and counted in a hemocytometer. Total number of cells and total number live cells were determined for both control and treated cells to determine the percentage of viable cells.
IL-1β secretion assay
IL-1β levels in isolated samples were assessed using an OptEIA enzyme-linked immunosorbent assay (ELISA) human IL-1β kit (BD Pharmingen, San Diego, CA). In brief, capture antibody diluted in coating buffer was applied to wells of 96-well flat-bottom micro-plates specifically designed for ELISA (Fisher Scientific). The plates were incubated overnight at 4°C and then excess capture antibody was removed by washing the plate three times with wash buffer (PBS containing 0.05% [v/v] Tween-20; PBST). The wells were then treated with blocking buffer to prevent non-specific binding and the plate was sealed and incubated at room temperature for 1 h. Blocking buffer was removed with three washes of PBST and cell supernatants and IL-1β standards were added to dedicated wells; the plate was re-sealed and incubated at room temperature for 2 h. Thereafter, the plate wells were thoroughly washed five times with PBST and each received 100 μl of a solution containing IL-1β detection antibody conjugated with horseradish peroxidase. After incubation for 1 h at room temperature, excess detection antibody in each well was removed by seven washes with PBST and a kit-provided substrate solution was added to each well for 30 min (room temperature) to produce a colored product. The reaction was ended by addition of 100 μl 1 N phosphoric acid. The absorbance in each well was then measured at 450 nm on a Multiskan MCC/340 plate reader (Fisher Scientific).
Statistical analysis
Statistical analysis of the data was carried out using an analysis of variance (ANOVA) and a Student’s t-test. Data were initially compared within a given experimental set-up via a one-way ANOVA. A significant ANOVA outcome was then followed by a pair-wise analysis of control vs exposed data using the Student’s t-test; p-values < 0.05 were considered significant.
Results
Viability of NK cells, MD-PBMC and PBMC exposed to TBBPA, HBCD and selective enzyme inhibitors
Exposure of NK cells, MD-PBMC and PBMC to 0.05–5.0 μM TBBPA for 24 h, 48 h and 6 days had no significant effect on viability. HBCD exposures (0.05–5.0 μM) also had no effects on viability of highly purified NK cells, MD-PBMC and PBMC after 24 h and 48 h exposures. However, exposure of MD-PBMC to 5 μM HBCD did slightly decrease cell viability after 6 days (control cell viability = 98.7 [± 2.2]%, 5 μM HBCD-treated cell viability = 86.2 [± 11.1]%). Exposure of MD-PBMC to pathway inhibitors + HBCD or pathway inhibitors alone for 24 h also had no significant effects on viability.
Effects of TBBPA exposure on NK cell IL-1β secretion
shows the effects of exposing NK cells to 0, 0.05, 0.1, 0.25, 0.5, 1, 2.5 and 5 μM for 24 h, 48 h or 6 days on IL-1β secretion from five donors tested (KB = Key Biologic Buffy Coat). Cells from three of five donors showed increased secretion when exposed to 0.05, 0.1 or 0.25 μM after 24- and 48-h exposures. Concentrations that caused increases varied from one donor to the next. For example, cells from donor KB129 treated with 0.05, 0.1 or 0.25 μM TBBPA showed significant increases of 13.6-, 13.3- and 4.3-fold, respectively, after 24 h; cells from donor KB181 showed increases of 5.3-, 5.4- and 4.0-fold at these same doses. When NK cells were exposed to TBBPA for 24 h, all five donors cells’ had significant decreases in IL-1β secretion at a 5 μM dose. shows the effects of TBBPA on IL-1β secretion at each timepoint for an individual donor (KB129).
Figure 1. Effects of exposures to TBBPA on IL-1β secretion from human NK cells, monocyte-depleted PBMC and PBMC. (a) NK cells (donor KB129). (b) Monocyte-depleted PBMC (donor F177). (c) PBMC (donor F188). All doses 0.05–5.0 μM TBBPA. Data shown are means ± SD. * Value significantly different from appropriate control (0 μM) at p < 0.05.
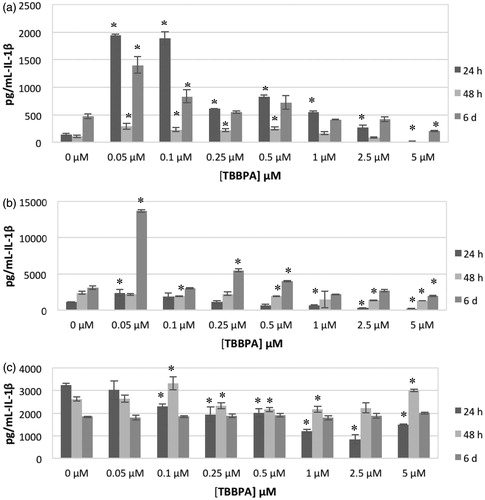
Table 1. Effects of TBBPA exposures on IL-1β secretion from highly purified human NK cells.
Effects of TBBPA exposure on MD-PBMC of IL-1β secretion
The effects of exposures to TBBPA on secretion of IL-1β from MD-PBMC after 24 h, 48 h and 6 days from four donors (F = filter obtained from Red Cross) are shown in . This preparation is mostly NK cells and T-cells. Cells from two of four donors showed substantial increases in IL-1β secretion when exposed to 0.05 μM TBBPA (F177 showed 2.2-fold while F184 showed 9.6-fold). Cells from all donors showed statistically significant increases in IL-1β secretion when exposed to at least one concentration of TBBPA at one or more length of exposure. Additionally, cells from all donors, with the exception of F184, also showed statistically significant decreases in secretion at one or more length of exposure to one or more concentration of TBBPA. shows the effects of TBBPA on IL-1β secretion at each timepoint for an individual donor (F177).
Table 2. Effects of TBBPA exposures to on IL -1β secretion from monocyte-depleted PBMC.
Effects of TBBPA exposure on PBMC IL-1β secretion
The effects of exposures to TBBPA on secretion of IL-1β from PBMC after 24 h, 48 h and 6 days from five donors (F = filter from Red Cross) are shown in . As with NK cells and MD-PBMC, the effects of TBBPA exposures varied considerably from one donor to the next. After a TBBPA 24-h exposure, cells from three donors showed statistically significant increases in IL-1β secretion at a minimum of one concentration of TBBPA. These same donors’ cells also had statistically significant decreases in secretion at a minimum of one TBBPA exposure level. IL-1β secretion was decreased upon exposure to most concentrations of TBBPA in cells from two donors after a 24 h exposure. These same patterns persisted after 48 h and 6 days exposures. shows effects of TBBPA on IL-1β secretion at each timepoint for an individual donor (F188).
Table 3. Effects of TBBPA exposures to TBBPA on IL-1β secretion from PBMC.
Effects of HBCD exposure on NK cell IL-1β secretion
The effects of exposing highly purified NK cells to 0, 0.05, 0.1, 0.25, 0.5, 1, 2.5 or 5.0 μM for 24 h, 48 h or 6 days on IL-1β secretion from three donors tested (KB = Key Biologic Buffy Coat) are shown in . There were significant increases in IL-1β secretion from NK cells from all donors examined after all lengths of exposure to HBCD. Concentrations that caused increases varied from one donor to the next at each timepoint. For instance, the cells from donor KB179 showed significant increases after a 24 h exposure to 0.1–1.0 μM HBCD, while NK cells from donor KB182 showed increased secretion of IL-1β when exposed to 0.05–5.0 μM HBCD.
Table 4. Effects of HBCD exposures to on IL -1β secretion from highly-purified human NK cells.
Cells from all donors showed significant increases in IL-1β secretion at all lengths of exposure when treated with 0.5, 1.0 or 2.5 μM HBCD. The magnitude of increases in secretion varied from one donor to the next. For instance, cells from donor KB 179 treated with 0.5, 1.0 or 2.5 μM HBCD showed significant increases of 1.3-, 1.4- and 1.4-fold, respectively, after 24 h; cells from donor KB181 showed significant 2.3-, 3.1- and 23.0-fold increases at these same doses. This same general pattern persisted after 48 h and 6 days exposures. shows the effects of HBCD on IL-1β secretion at each timepoint for an individual donor (KB181).
Figure 2. Effects of exposures to HBCD on IL-1β secretion from human NK cells, monocyte-depleted PBMC and PBMC. (a) NK cells (donor KB181). (b) Monocyte-depleted PBMC (donor F202). (c) PBMC (donor F189). All doses 0.05–5.0 μM HBCD. Data shown are means ± SD. * Value significantly different from appropriate control at p < 0.05.
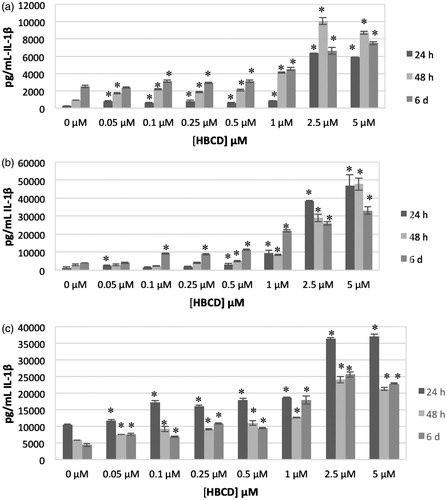
Effects of HBCD exposure on MD-PMBC IL-1β secretion
shows the effects of exposures to HBCD on secretion of IL-1β from MD-PBMC after 24 h, 48 h and 6 days from five donors (F = filter from Red Cross). There were significant increases in IL-1β secretion from MD-PBMC from all donors examined after all lengths of exposure to HBCD. Significant increases in IL-1β secretion were seen at the 2.5 μM concentration for all donors after all lengths of exposure. Additional concentrations of HBCD caused increases in IL-1β secretion, depending on the donor; magnitude of increase at a given concentration of HBCD also varied among donors. For instance, cells from donor F198 treated with 0.5, 1, 2.5 or 5 μM HBCD had significant increases of 1.7-, 5.0-, 48- and 57-fold, respectively, after 24 h; cells from donor F196 had 1.2-, 1.2-, 1.7- and 2.0-fold significant increases under the same conditions. shows effects of HBCD on IL-1β secretion at each timepoint for an individual donor (F202).
Table 5. Effects of HBCD exposures on IL -1β secretion from monocyte-depleted PBMC.
Effects of HBCD exposure on PBMC IL-1β secretion
The effects of exposing PBMC from six donors to HBCD are shown in . Cells from all donors showed increased secretion of IL-1β at multiple HBCD concentrations after all lengths of exposure. Cells from five of six donors showed increases in IL-1β secretion with 2.5 μM HBCD after 24 h and 6 days. All donors showed increased secretion of IL-1β at 2.5 μM after 48-h exposures. Cells from five of six donors also showed increased secretion of IL-1β with 5 μM HBCD after 24- and 48-h exposures. All donor cells showed increased secretion of IL-1β at 5 μM HBCD after 6 days of exposure. shows the effects of HBCD on IL-1β secretion at each timepoint for an individual donor (F189).
Table 6. Effects of HBCD exposures on IL -1β secretion from PBMC.
Effects of HBCD exposure on IL-1β secretion by MD-PBMC with selective enzyme inhibitors
IL-1β cleavage inhibitor (Caspase-1 inhibitor II)
The effects of exposures to 0.5, 1.0 and 2.5 μM HBCD on secretion of IL-1β from MD-PBMC whose Caspase-1 had been inhibited with Caspase-1 inhibitor II (50 μM) are shown in . Caspase I enzyme is essential for IL-1β synthesis; Caspase-1 inhibitor II inhibited baseline IL-1β production. Cells exposed to HBCD showed no change or higher fold-increase in IL-1β secretion in the presence of the inhibitor in cells from six of the nine donors. Cells from the other three donors had decreases in HBCD-stimulated secretion when caspase-1 activity was inhibited. shows the effects of inhibiting caspse-1 on HBCD-induced increases in IL-1β secretion from MD-PBMC from donor F267. There were 1.6-, 2.8- and 4.8-fold increases in IL-1β secretion when MD-PBMC were exposed to 0.5, 1.0 or 2.5 μM HBCD, respectively, in the absence of Caspase-I inhibitor. When inhibitor was present, these same HBCD exposures caused 1.9-, 2.9- and 10.5-fold increases in IL-1β secretion. These results suggested the Caspase I pathway was not a reproducible target for HBCD-induced increases in IL-1β secretion.
Figure 3. Effects of 24-h exposure to HBCD on IL-1β secretion from monocyte-depleted PBMC pre-treated with selective enzyme inhibitors. Data shown is based on cells from an individual donor. (a) Caspase I inhibitor II (Caspase I) (donor F 267). (b) NF-κB inhibitor (BAY 11-7085) (donor F275). (c) JNK inhibitor (donor F271). (d) MEK1/2 (ERK1/2 pathway) inhibitor (PD98059) (donor F267). (e) p38 inhibitor (SB202190) (donor F255). * Value significantly different from control cells with vehicle alone at p < 0.05. ** Value significantly different from control + inhibitor at p < 0.05.
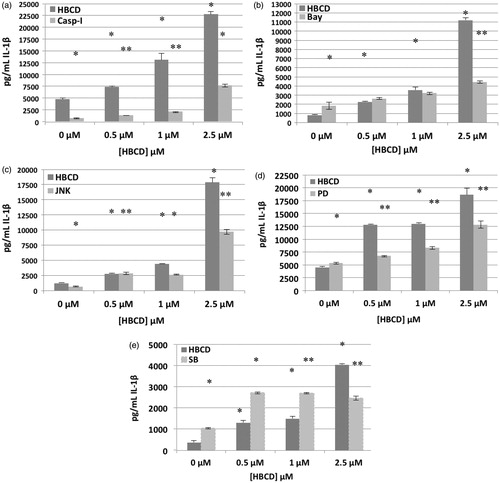
Table 7. Effects of 24 h exposure to HBCD ± pathway inhibitors on IL-1β secretion from MD-PBMC.
Table 8. Effects of 24 h exposure to HBCD ± pathway inhibitors on IL-1β secretion from MD-PBMC.
Table 9. Effects of 24 h exposure to HBCD ± pathway inhibitors on IL-1β secretion from MD-PBMC.
Table 10. Effects of 24 h exposure to HBCD ± pathway inhibitors on IL-1β secretion from MD-PBMC.
Table 11. Effects of 24 h exposure to HBCD ± pathway inhibitors on IL-1β secretion from MD-PBMC.
Nuclear factor-κB (NF-κB) inhibitor (BAY 11-7085)
The effects of exposures to 0.5, 1.0 or 2.5 μM HBCD on secretion of IL-1β from MD-PBMC whose NF-κB had been inhibited with Bay 11-7085 (0.325 μM) are shown in . BAY inhibited the ability of HBCD to increase IL-1β secretion from MD-PBMC from two of five donors. However, inhibiting the NF-κB pathway increased HBCD-induced IL-1β secretion by MD-PBMC from two of five donors and left secretion unchanged in cells from one donor. For example, MD-PBMC from donor F275 had 2.7-, 4.3- and 13.5-fold increases when exposed to 0.5, 1.0 and 2.5 μM HBCD, respectively, in the absence of inhibitor; in contrast, when exposed to the same concentrations in the presence of inhibitor, these cells had 1.4-, 1.8- and 2.4-fold increases. On the other hand, cells from donor F256 had 1.4-, 1.8- and 5.1-fold increases in secretion when exposed to 0.5, 1.0 and 2.5 μM HBCD, respectively, in the absence of an inhibitor, but values 3.4-, 3.9- and 12-fold higher in the presence of an inhibitor (). These results indicated that NF-κB pathways may be to some extent involved in HBCD-induced stimulation of IL-1β secretion, but the role(s) may vary depending on the status of the donor.
JNK inhibitor (BI78D3)
The effects of exposures to 0.5, 1.0 or 2.5 μM HBCD on IL-1β secretion by MD-PBMC whose JNK had been inhibited with BI78D3 are shown in . Cells from two of five donors continued to show an increase in IL-1β secretion in response to HBCD when a JNK inhibitor was present. In (representative data from donor F271) it can be seen there were 2.3-, 3.6- and 15-fold increases when MD-PBMC were exposed to 0.5, 1.0 or 2.5 μM HBCD in the absence of inhibitor. When an inhibitor was present, those same HBCD exposures were still able to cause 4.3-, 4.1- and 14.9-fold increases in IL-1β secretion. However, with cells from donor F278, while there were 2.2-, 1.9- and 7.6-fold increases when MD-PBMC were exposed to 0.5, 1.0 or 2.5 μM HBCS in the absence of an inhibitor, when the latter was present these same HBCD doses caused 1.0-, 1.2- and 3.1-fold increases in secretion. These results revealed that JNK pathways might be somewhat involved in HBCD-stimulated IL-1β secretion but, as with NF-κB, the role(s) in HBCD-induced increases in IL-1β release likely vary depending on the status of the donor.
MEK inhibitor (PD98059) (ERK1/2 pathway)
The effects of exposures to 0.5, 1.0 and 2.5 μM HBCD on IL-1β secretion by MD-PBMC whose MEK had been inhibited with PD98059 (50 μM) are shown in . As with the other inhibitors, PD98059 had variable effects on baseline IL-1β secretion. Cells exposed to HBCD showed a lower-fold increase in IL-1β secretion in the presence of inhibitor. In (representative data from donor F267), 2.8-, 2.9- and 4.1-fold increases in IL-1β secretion were noted when MD-PBMC were exposed to 0.5, 1.0 or 2.5 μM HBCD in the absence of an ERK1/2 inhibitor. When an inhibitor was present, these exposures caused 1.3-, 1.6- and 2.4-fold increases in secretion. The same pattern was observed in the majority of donors’ cells tested. This indicated the ERK1/2 pathway was a target of HBCD that led to increased secretion of IL-1β.
p38 inhibitor (SB202190)
The effects of exposures to 0.5, 1 and 2.5 μM HBCD on IL-1β secretion by MD-PBMC whose p38 had been inhibited with SB202190 are shown in . Cells exposed to HBCD showed a lower fold increase in IL-1β secretion in the presence of inhibitor. In (representative data from donor F255), 3.5-, 4.0- and 11-fold increases were noted with MD-PBMC exposed to 0.5, 1.0 and 2.5 μM HBCD in the absence of a p38 pathway inhibitor. When the inhibitor was present, the same exposures caused 2.6-, 2.6- and 2.6-fold increases in secretion (). The same trend was seen with cells from all donors tested. This indicated the p38 pathway was being utilized by HBCD to lead to increases in IL-1β secretion.
Discussion
IL-1β is a potent inflammatory cytokine that promotes tumor growth and invasiveness; IL-1β also produces a tumor microenvironment that promotes epithelial mesenchymal transition (Lee et al. Citation2015). TBBPA and HBCD are known to contaminate the environment due to their widespread use in electrical/electronic equipment, plastics, textiles and upholstery (Covaci et al. Citation2006; HSDB, 2001; IPCS/WHO Citation1995). TBBPA and HBCD can alter the lytic function of NK cells, cell surface protein expression and MAPK activation (Cato et al. Citation2014; Hinkson and Whalen Citation2009, Citation2010; Hurd and Whalen Citation2011; Kibakaya et al. Citation2009). Dysregulation of IL-1β levels changes a host defense capability and also has a role in tumor growth and progression (Dinarello Citation2009). TBBPA and HBCD have been shown to dysregulate secretion of interferon (IFN)-γ from human immune cells (Almughamsi and Whalen Citation2015). Experiments with the environmental contaminant tributyltin have shown that it is able to alter secretion of IL-1β from human immune cells (Brown and Whalen Citation2015). Thus, it is important to determine whether TBBPA and HBCD are able to alter secretion of IL-1β by immune cells.
The study reported here examined the effects of both TBBPA and HBCD on secretion of IL-1β by immune system cells in an ex vivo human model. The concentration ranges tested were based on those that had caused effects on NK lytic function, cell-surface protein expression and MAPK activity (Cato et al. Citation2014; Hinkson and Whalen Citation2009, Citation2010; Hurd and Whalen Citation2011; Kibakaya et al. Citation2009). Three distinct cell preparations (NK cells, MD-PBMC and PBMC) were used in this study. This type of study is important in order to determine if increasing the complexity of the cell population altered the effects of exposures to the compounds. As it is not possible to carry out studies of these compounds in humans, studies carried out in more re-constituted systems (such as the ones examined here) will more closely approximate effects that might be seen in vivo. Additionally, this study explored which signaling pathways might be involved in any HBCD-induced alterations of IL-1β levels noted in the secretion studies.
Baseline secretion of IL-1β varied among different donors as well as different cell preparations. Similar baseline secretion of IL-1β has been seen from NK cells and MD-PBMC. PBMC showed larger baseline secretion of IL-1β due to the presence of monocytes. Monocytes and macrophages are the foremost IL-1β-secreting immune cells (Dinarello Citation2009). TBBPA effects on IL-1β secretion varied from one donor to the next at each timepoint. In general, the secretion of IL-1β for all cell types showed a significant increase in at least one concentration and at least one length of exposure from each cell preparation. In most cases, 5 μM TBBPA caused decreased IL-1β secretion by all three different cell preparations. A previous study examining the effect of TBT on secretion of IL-1β from immune system cells showed that TBT blocked IL-1β secretion at the highest concentration (200 nM) while causing increases in secretion at lower doses (5–50 nM) (Brown and Whalen Citation2015).
Each cell preparation (NK cells, MD-PBMC, PBMC) had similar responses to HBCD. For instance, when highly purified NK cells were exposed to 0.05–5.0 μM HBCD, there were significant increases in IL-1β secretion. The ability of HBCD to increase IL-1β levels did not seem to change as the cell preparation complexity increased. Both MD-PBMC and PBMC had increased IL-1β secretion in response to HBCD – as did NK cells. The maximum fold increases in each cell preparation occurred over similar ranges of HBCD concentration after 24 h. NK cells (donor KB179) had maximal fold increases of IL-1β secretion with 0.5–2.5 μM HBCD, MD-PBMC (donor F204), over the 0.5–5.0 μM dose range and the most complex cell preparation, PBMC (donor F186), in the 0.5–5.0 μM HBCD range. The magnitude of maximum fold increase varied among the donors.
Immune cell IL-1β production/release is facilitated by Caspase I and several signaling pathways. These include NF-κB and MAPKs (ERK1/2, p38 and JNK) (Baldassare et al. Citation1999; Fantuzzi and Dinarello Citation1999; Kim et al. Citation2004) (). The present study showed that inhibition of Caspase I occasionally led to inhibition of HBCD-induced increases (cells from three of nine donors) in IL-1β secretion. However, in cells from six of nine donors, inhibition of Caspase I did not affect HBCD-induced increases in IL-1β secretion. Thus, the role of Caspase-I in HBCD-induced increased IL-1β secretion appears to vary depending on the cell donor. Although Caspase I is important for IL-1β secretion by immune cells, it does not appear essential for HBCD- induced increases in secretion of this cytokine.
IL-1β gene transcription is induced by NF-κB signaling pathway components (Scheibel et al. Citation2010). The results of this study revealed the same mixed effects as were seen with Caspase-I when NF-κB was inhibited by BAY11-7085. Previous studies have shown that TBT-induced elevations of IL-1β did not require NF-κB pathways (Brown and Whalen Citation2015). Other studies showed that the role of NF-κB in HBCD-induced increases in IFNγ secretion also varied among different donors (Almughamsi and Whalen Citation2015).
Three members of the MAP kinase pathway (JNK, ERK1/2 and p38) were examined in the current study. ERK1/2 has a vital role in cellular activities like cell proliferation, mitosis, gene expression, metabolism and apoptosis (Nishida and Gotoh Citation1993; Robinson and Cobb Citation1997). IL-1β gene activation can be expedited by ERK1/2 pathways – likely through the AP-1 transcription activator (Glauser and Schlegel Citation2007). Both JNK and p38 pathways also regulate IL-1β production in some cell types (Baldassare et al. Citation1999; Fantuzzi and Dinarello Citation1999; Kim et al. Citation2004), possibly via AP-1 and/or C/EBPβ/NFIL-6. The present study showed that inhibition of the JNK pathway sometimes led to inhibition of HBCD-induced increases in IL-1β secretion in cells from three of five donors and in two of five cases, slightly elevated (or did not affect) any HBCD-induced increases in IL-1β secretion. Thus, the role of JNK in HBCD-induced increases in IL-1β secretion appeared to vary depending on the cell donor. However, inhibition of both the ERK1/2 and p38 pathways caused a diminution in the HBCD-induced secretion of IL-1β. Thus, HBCD appears to utilize both the ERK1/2 and p38 pathways to stimulate increased IL-1β secretion. Previous studies have shown two MAPK family members (ERK1/2 and p38) seem to be targets for TBT-induced alterations in IL-1β levels (Brown and Whalen Citation2015). Inhibition of the p38 pathway also diminished HBCD-induced increases in secretion of IFNγ (Almughamsi and Whalen Citation2015).
In summary, the current study indicated that IL-1β secretion by three human immune cell preparations was altered by exposures to TBBPA or HBCD. HBCD treatment predominantly caused increases in IL-1β secretion. TBBPA also increased IL-1β secretion at least at one dose; however, 5 μM TBBPA generally inhibited secretion. These results suggested that both flame-retardant compounds were able to disrupt IL-1β secretion from increasingly complex human immune cell preparations, with the effects maintained even in the most complex preparation examined. This was significant in that this preparation was more physiologically relevant. Individuals who do not work with these compounds have levels that are well below the levels where effects were seen in these studies (Nagayama et al. Citation2001; Thomsen et al. Citation2002, Citation2008; Cariou et al. Citation2008; Schecter et al. Citation2012). However, occupationally-exposed workers experience considerably higher levels (Thomsen et al. Citation2007). For instance, a level of 5 nM HBCD was seen in the serum of a worker in a plant where HBCD was added to expandable polystyrene. This level is within 10-fold of the lowest level of HBCD that was tested here (50 nM), where increases in IL-1β secretion in many donor cells were seen. It may be worthwhile to examine even lower levels of these compounds, for effects on IL-1β in future studies, as many of the donors showed increased IL-1β production even at the lowest concentrations examined.
Thus, occupational exposure – most especially of workers producing these compounds – may be of concern. In general, levels in workers involved in the production of these compounds appear to have not been measured. Over-production of IL-1β is related to chronic inflammation in some diseases (like rheumatoid arthritis and multiple sclerosis) as well as playing an important role in tumor development (Choy and Panayi Citation2001; Lucas and Hohlfeld Citation1995). These studies also showed that ERK1/2 and p38 pathways were needed for HBCD-induced increases in IL-1β secretion by the cell populations studied here.
Acknowledgments
This work was supported by grant U54CA163066 from the National Institutes of Health.
Disclosure statement
The authors report no conflicts of interest. The authors alone are responsible for the content and writing of the paper.
References
- Allan SM, Tyrrell PJ, Rothwell NJ. 2005. IL-1 and neuronal injury. Nat Rev Immunol. 5:629–640.
- Almughamsi HM, Whalen MM. 2015. Hexabromocyclododecane and tetrabromobisphenol a alter secretion of IFNγ from human immune cells. Arch Toxicol. [Epub ahead of print]. doi: 10.1007/s00204-015-1586-6.
- Apte RN, Dotan S, Elkabets M, White MR, Reich E, Carmi Y, Song X, Dvozkin T, Krelin Y, Voronov E. 2006. The involvement of IL-1 in tumorigenesis, tumor invasiveness, metastasis and tumor-host interactions. Cancer Metastasis Rev. 25:387–408.
- Arlt A, Vorndamm J, Müerköster S, Yu H, Schmidt WE, Folsch UR, Schafer H. 2002. Autocrine production of IL–1β confers constitutive NF-κB activity and chemo-resistance in pancreatic carcinoma cell lines. Cancer Res. 62:910–916.
- Baldassare JJ, Bi Y, Bellone CJ. 1999. The role of p38 mitogen-activated protein kinase in IL-1β transcription. J Immunol. 162:5367–5373.
- Balkwill F, Coussens LM. 2004. Cancer: An inflammatory link. Nature. 431:405–406.
- Bar D, Apte RN, Voronov E, Dinarello CA, Cohen S. 2004. Continuous delivery system of IL-1 receptor antagonist reduces angiogenesis and inhibits tumor development. FASEB J. 18:161–163.
- Birnbaum LS, Staskal DF. 2004. Brominated flame retardants: Cause for concern? Environ Health Perspect. 112:9–17.
- Brown S, Whalen M. 2015. Tributylin alters secretion of IL-1β from human immune cells. J Appl Toxicol. 35:895–908.
- Bustnes JO, Yoccoz NG, Bangjord G, Polder A, Skaare JU. 2007. Temporal trends (1986–2004) of organochlorines and brominated flame retardants in tawny owl eggs from northern Europe. Environ Sci Technol. 41:8491–8497.
- Cariou R, Antignac JP, Zalko D, Berrebi A, Cravedi JP, Maume D, Marchand P, Monteau F, Riu A, Andre F, et al. 2008. Exposure assessment of French women and their newborns to tetrabromobisphenol A: Occurrence measurements in maternal adipose tissue, serum, breast milk, and cord serum. Chemosphere. 73:1036–1041.
- Cato A, Celada L, Kibakaya EC, Simmons B, Whalen MM. 2014. Brominated flame retardants, tetrabromobisphenol A and hexabromocyclododecane, activate mitogen-activated protein kinases (MAPKs) in human natural killer cells. Cell Biol Toxicol. 30:345–360.
- Choy EH, Panayi GS. 2001. Cytokine pathways and joint inflammation in rheumatoid arthritis. New Engl J Med. 344:907–916.
- Church LD, Cook GP, McDermott MF. 2008. Primer: Inflammasomes and IL-1β in inflammatory disorders. Nat Clin Pract Rheumatol. 4:34–42.
- Covaci A, Gerecke AC, Law RJ, Voorspoels S, Kohler M, Heeb NV, Allchin LH. 2006. Hexa-bromocyclododecanes (HBCD) in the environment and humans: a review. Environ Sci Technol. 40:3679–3688.
- Dinarello CA. 1996. Biologic basis for IL-1 in disease. Blood. 87:2095–2147.
- Dinarello CA. 2009. Immunological and inflammatory functions of the IL-1 family. Annu Rev Immunol. 27:519–550.
- Dinarello CA. 2010. Why not treat human cancer with IL-1 blockade? Cancer Metastasis Rev. 29:317–329.
- Elaraj DM, Weinreich DM, Varghese S, Puhlmann M, Hewitt SM, Carroll NM, Feldman ED, Turner EM, Alexander HR. 2006. Role of IL-1 in growth and metastasis of human cancer xenografts. Clin Cancer Res. 12:1088–1096.
- EFSA (European Food Safety Authority). 2011. Scientific opinion on tetrabromobisphenol A (TBBPA) and its derivatives in food. EFSA J. 9:2477.
- Fantuzzi G, Dinarello CA. 1999. IL-18 and IL-1β: two cytokine substrates for ICE (caspase-1). J Clin Immunol. 19:1–11.
- Glauser DA, Schlegel W. 2007. Sequential actions of ERK1/2 on AP-1 transcription factor allow temporal integration of metabolic signals in pancreatic β-cells. FASEB J. 21:3240–3249.
- Hinkson NC, Whalen MM. 2009. Hexabromocyclododecane decreases the lytic function and ATP levels of human natural killer cells. J Appl Toxicol. 29:656–661.
- Hinkson NC, Whalen MM. 2010. Hexabromocyclododecane decreases tumor-cell-binding capacity and cell-surface protein expression of human NK cells. J Appl Toxicol. 30:302–309.
- HSDB (Hazardous Substances Data Bank). 2001. 2,2′,6,6′-Tetrabromobisphenol A. Bethesda, MD: National Library of Medicine. Available at: http://www.toxnet.nlm.nih.gov/cgi-bin/sis/search [last accessed 2010].
- Hurd T, Whalen MM. 2011. Tetrabromobisphenol A decreases cell-surface proteins involved in human natural killer (NK) cell-dependent target cell lysis. J Immunotoxicol. 8:219–227.
- IPCS/WHO (International Program on Chemical Safety/World Health Organization). 1995. Environmental Health Criteria 172: tetrabromobisphenol A and Derivatives. Geneva: World Health Organization.
- Jin L Yuan RQ, Fuchs A, Yao Y, Joseph A, Schwall R, Schnitt SJ, Guida A, Hastings HM, Andres J, et al. 1997. Expression of IL-1β in human breast carcinoma. Cancer. 80:421–434.
- Kibakaya EC, Stephen K, Whalen MM. 2009. Tetrabromobisphenol A has immunosuppressive effects on human natural killer cells. J Immunotoxicol. 6:285–292.
- Kim SH, Smith CJ, Eldik LJ. 2004. Importance of MAPK pathways for microglial pro-inflammatory cytokine IL-1β production. Neurobiol Aging. 25:431–439.
- Law RJ, Kohler M, Heeb NV, Gerecke AC, Schmid P, Voorspoels S, Covaci A, Becher G, Janák K, Thomsen C. 2005. Hexabromocyclododecane challenges scientists and regulators. Environ Sci Technol. 39:281A–287A.
- Lee CA, Chang JS, Syu SH, Wong TS, Chan JY, Tang YC, Yang ZP, Yang WC, ChenCT, Lu SC, et al. 2015. IL-1β promotes malignant transformation and tumor aggressiveness in oral cancer. J Cell Physiol. 230:875–884.
- Legler J, Brouwer A. 2003. Are brominated flame retardants endocrine disruptors? Environ Intl. 29:879–885.
- Lewis A, Varghese S. 2006. IL-1 and cancer progression: Emerging role of IL-1 receptor antagonist as a novel therapeutic agent in cancer treatment. J Translat Med. 12:1–12.
- Lucas K, Hohlfeld R. 1995. Different aspects of cytokines in the immunopathology of multiple sclerosis. Neurology. 45:S4–S5.
- Mack AG. 2004. Flame retardants, halogenated. In: Seidel A, Bickford M, editors. Kirk-Othmer Encyclopedia of Chemical Technology. 5th ed. Hoboken (NJ): Wiley and Sons.
- Mathias S, Younes A, Kan CC, Orlow I, Joseph C, Kolesnick RN. 1993. Activation of the sphingomyelin signaling pathway in intact EL-4 cells and in a cell-free system by IL-1β. Science. 259:519–522.
- Meerts IA, van Zanden JJ, Luijks EA, van Leeuwen-Bol I, Marsh G, Jakobsson E, Bergman A, Brouwer A. 2000. Potent competitive interactions of some brominated flame retardants and related compounds with human transthyretin in vitro. Toxicol Sci. 56:95–104.
- Meyer TP, Zehnter I, Hofmann B, Zaisserer J, Burkhart J, Rapp S, Weinauer F, Schmitz J, Illert WE. 2005. Filter Buffy Coats (FBC): A source of peripheral blood leukocytes recovered from leukocyte depletion filters. J Immunol Meth. 307:150–166.
- Muerkoster SS Lust J, Arlt A, Häsler R, Witt M, Sebens T, Schreiber S, Fölsch UR, Schäfer H. 2006. Acquired chemo-resistance in pancreatic carcinoma cells: Induced secretion of IL-1β and NO lead to inactivation of caspases. Oncogene. 25:3973–3981.
- Nagayama J, Takasuga T, Tsuji H. 2001. Contamination levels of brominated flame retardants, dioxins, and organochlorine compounds in the blood of Japanese adults. Human Levels Trends. 2001:218–221.
- Nishida E, Gotoh Y. 1993. MAP kinase cascade is essential for diverse signal transduction pathways. Trends Biochem Sci. 18:128–131.
- Palace V, Park B, Pleskach K, Gemmill B, Tomy G. 2010. Altered thyroxine metabolism in rainbow trout (Oncorhynchus mykiss) exposed to hexabromocyclododecane (HBCD). Chemosphere. 80:165–169.
- Peterman PH, Orazio CE, Gale RW. 2000. Detection of tetrabromobisphenol A and formation of brominated [13C]-bisphenol A in commercial drinking water stored in reusable poly-carbonate containers. ACS Div Environ Chem. 40:431–433.
- Polder A, Venter B, Skaare JU, Bouwman H. 2008. Polybrominated diphenyl ethers and HBCD in bird eggs of South Africa. Chemosphere. 73:148–154.
- Raingeaud J, Gupta S, Rogers JS, Dickens M, Han J, Ulevitch RJ, Davis RJ. 1995. Pro-inflammatory cytokines and environmental stress cause p38 mitogen-activated protein kinase activation by dual phosphorylation on tyrosine and threonine. J Biol Chem. 270:7420–7426.
- Robinson MJ, Cobb MH. 1997. Mitogen-activated protein kinase pathways. Curr Opin Cell Biol. 9:180–186.
- Saegusa Y, Fujimoto H, Woo GH, Inoue K, Takahashi M, Mitsumori K, Hirose M, Nishikawa A, Shibutani M. 2009. Developmental toxicity of brominated flame retardants tetrabromobisphenol A and 1,2,5,6,9,10-hexabromocyclododecane in rat offspring after maternal exposure from mid-gestation through lactation. Reprod Toxicol. 28:456–467.
- Schecter A, Szabo DT, Miller J, Gent TL, Malik-Bass N, Petersen M, Paepke O, Colacino JA, Hynan LS, Harris TR, et al. 2012. EHP: Hexabromocyclododecane (HBCD) Stereoisomers in U.S. Food from Dallas, Texas. Environ Health Perspect. 120:1260–1264.
- Scheibel M, Klein B, Merkle H, Schulz M, Fritsch R, Greten FR, Arkan MC, Schneider G, Schmid RM. 2010. IκBβ is an essential co-activator for LPS-induced IL-1β transcription in vivo. J Exp Med. 207:2621–2630.
- Simi A, Tsakiri N, Wang P, Rothwell NJ. 2007. IL-1 and inflammatory neurodegeneration. Biochem Soc Trans. 35:1122–1126.
- Thomsen C, Lundanes E, Becher G. 2002. Brominated flame retardants in archived serum samples from Norway: A study on temporal trends and the role of age. Environ Sci Technol. 36:1414–1418.
- Thomsen C, Molander P, Daae HL, Janák K, Froshaug M, Liane VH, Thorud S, Becher G, Dybing E. 2007. Occupational exposure to hexabromocyclododecane at an industrial plant. Environ Sci Technol. 41:5210–5216.
- Thomsen C, Knutsen HK, Liane VH, Frøshaug M, Kvalem HE, Haugen M, Meltzer HM, Alexander J, Becher G. 2008. Consumption of fish from a contaminated lake strongly affects the concentrations of polybrominated diphenyl ethers and hexabromocyclododecane in serum. Mol Nutr Food Res. 52:228–237.