Abstract
Several studies have been performed to develop effective neutralizing monoclonal antibodies. The Epstein–Barr virus (EBV) can efficiently immortalize B-cells to establish lymphoblastoid cell lines (LCL) and so it has been used extensively for transformation of B-cells to produce and secrete immunoglobulin. The present study addressed the effect of TLR7/8 agonist (R848), feeder cells layer and fluorescence-activated cell sorting (FACS) and magnetic-activated cell sorting (MACS) cell separation methods on the transformation efficiency of antibody-producing memory B-cells. For these studies, the antigen used for analyses of antibody formation was the tetanus neurotoxin (TeNT) derived from Clostridium tetani. The results here showed that employing an HFFF.PI6 feeder cell layer, R848 agonist and FACS-mediated purification of memory B-cells led to increased transformation efficiency. Altogether, the effects of the R848 and the feeder cells provided an efficient method for EBV transformation of human B-cells. Moreover, there was an advantage in using FACS sorting of B-cells over the MACS method in the context of EBV transformation and immortalization of precursors of antigen-specific B-cells.
Introduction
Due to the presence of xenogeneic antibodies, clinical application of mouse-derived monoclonal antibodies (mAb) is associated with several problems including human anti-mouse immune reaction (HAMA) (Tjandra et al. Citation1990). However, in the mid-1980s, chimerization and humanization provided state-of-the art techniques to avoid the side-effects associated with murine antibodies (Weiner et al. Citation2010). Phage display and Epstein–Barr virus (EBV) transformation have provided a great potential for selecting specific fully-human antibodies against various target antigens in vitro.
Transformation of human B-cells with EBV is a valuable method for the production of fully human mAb (Steinitz Citation2014). EBV is a member of B-lymphotropic gamma herpes virus family that infects B-cells (Middeldorp et al. Citation2003; Young and Rickinson Citation2004). EBV infection leads to proliferation and transformation of B-cells into immortalized lymphoblastoid cells (LCL) (Price and Luftig Citation2013). This unique ability of EBV is extensively used for the generation of immortalized B-cells that can produce antigen-specific human mAb (Traggiai Citation2012; Yousefi et al. Citation2013a,Citationb). However, the EBV life cycle and transformation of different B-cell sub-sets progress with various efficiencies.
While in vitro exposure of B-cells to EBV results in a high infection frequency, a small proportion of cells actually become transformed (∼1–3%) (Shokrgozar and Shokri Citation2001; Traggiai et al. Citation2004). Several methods have been reported to improve the efficiency of EBV transformation, including the use of γ-irradiated fibroblast cells or culture medium supplemented with human plasma to improve the survival and proliferation of EBV-infected B-cells (Pelloquin et al. Citation1986; Manor Citation2008). Although TLR agonists improve the efficiency of transformation in purified B-cells by a magnitude of 5–6 (Bourke et al. Citation2003; Iskra et al. Citation2010), the most evident enhancement of efficiency of transformation has been observed with memory B-cells treated with a TLR-9 agonist. In 2004, Traggiai et al. showed that TLR9 agonists (i.e. un-methylated CpG-containing oligonucleotides) enhanced the efficiency of EBV transformation of IgG+CD22+ memory B-cells that had been purified by a combination of magnetic and fluorescence-activated cell sorting. It was seen that stimulation of EBV-infected memory cells by CpG (as a polyclonal B-cell activator) increased the efficiency of transformation from 1–3% to 30–100% (Traggiai et al. Citation2004). A recent study from our laboratory showed that EBV infection of B-cells – in combination with a Toll-like receptor (TLR) 7/8 agonist – was more efficient than CpG in producing antigen-specific human mAb (Younesi et al. Citation2014). It seemed memory B-cells were the main source of EBV in vivo and probably the most competent sub-sets for EBV transformation (Babcock et al. Citation1998).
Other modifications to protocols have also been shown to affect EBV transformation and establishment of LCL. For example, CD19 and BCR co-ligation has been shown to boost EBV transformation and establishment of LCL (Hur et al. Citation2005). Nevertheless, little is known about the effect of choice of method by which B-cells are isolated on these parameters. There are two common methods for isolation and enrichment of sub-populations from peripheral blood cells, fluorescence-activated cell sorting (FACS) and magnetic-activated cell sorting (MACS) (Dainiak et al. Citation2007). To address the issue as to any preferable method, the present study investigated the effect of the two separation methods on efficiencies of EBV transformation among antibody producing memory B-cells. For this study, evaluation of efficiency was based upon responses against a specific antigen of interest, tetanus neurotoxin (TeNT). This antigen was selected due to the ongoing critical need to develop a neutralizing mAb against TeNT.
Tetanus neurotoxin is produced as a single polypeptide and cleaved to a two-chain active holotoxin, in which a 50 kDa amino (N)-terminal light chain (LC) and a 100 kDa carboxy (C)-terminal heavy (HC) chain are linked by a single disulfide bond. HC is composed of the amino terminal half (HN, ≈50 kDa) and the carboxy-terminal half (HC or fragment C, ≈50 kDa) which has a key role in binding to the neuron gangliosides (Yousefi et al. Citation2013a,Citationb). The humoral immune response plays a crucial role against tetanus and production and characterization of mAb against different parts of tetanus toxin (especially fragment C), each of which are important for ensuring success of passive immunization.
In this paper, the effects of use of a feeder layer, a TLR7/8 agonist (R848) and the FACS method upon EBV transformation efficiency and anti-TeNT-specific LCL production are reported. The current studies also determined the effects on specificity of TeNT-specific memory B-cells by employing this improved technique.
Materials and methods
Sample collection and PBMC preparation
To optimize EBV transformation, heparinized peripheral blood was obtained from eight healthy male subjects (age range of 26–32 years) that had been immunized with DT (Diphteria-Tetanus) vaccine (Razi Vaccine and Serum Research Institute, Karaj, Iran). All human samples were collected after Tehran University of Medical Sciences review board approval and patient written informed consent. Specifically, at 5–7 days post-immunization, 50 ml peripheral blood per subject was collected into heparinized tubes. Peripheral blood mononuclear cells (PBMC) were then isolated using Histopaque (Sigma, St Louis, MO) density-gradient centrifugation. Subsequently-purified PBMC were washed twice with RPMI 1640 culture medium and re-suspended in the same medium supplemented with 10% fetal bovine serum (FBS), penicillin (100 IU) and streptomycin (100 μg/ml) (all Sigma). Viability of isolated cells was routinely >95%, as assessed by trypan blue exclusion. The Ethical Committee of Tehran University of Medical Sciences approved all aspects of this study.
B-cell isolation using MACS
B-cells were isolated from the PBMC samples using MACS negative and positive selection kits (Miltenyi Biotec, Bergisch Gladbach, Germany), according to manufacturer instructions (Younesi et al. Citation2010). For naïve B-cell negative selection, PBMC were magnetically-labeled using kit-provided antibodies against non-B-cell markers (including CD2, CD14, CD16, CD27, CD36, CD43 and CD235). In brief, PMBC were washed with MACS buffer and re-suspended in 40 μl buffer (per 107 total cells). Subsequently, aliquots of the cells were combined with 10 μl B-cell biotin-antibody cocktail (per 107 total cells) and placed at 2–8 °C for 5 min. Thereafter, 30 μl MACS buffer and 20 μl B-cell microbead cocktail were added to each reaction tube. Each suspension was incubated for 10 min at 2–8 °C before MACS buffer (500 μl) was added and the B-cells then isolated by magnetic separation.
For B-cell positive selection, PBMC were magnetically-labeled with kit-provided antibody against only CD19. Here, PBMC were first washed twice with MACS buffer and then re-suspended in the buffer. An aliquot was removed and then combined with 10 μl anti-CD19 microbeads (per 107 total cells). The cells were then incubated for 15 min at 2–8 °C and then subsequently washed with kit buffer. The cells were ultimately re-suspended in 500 μl MACS buffer and B-cells then isolated by magnetic separation. CD27+ memory B-cells were isolated from the CD19+ negatively-selected B-cells using a MACS CD27 positive selection kit (Miltenyi Biotec) according to manufacturer instructions. Ultimately, purity of the MACS-isolated B-cells (including naïve B-cells [CD19+CD27−] and memory B-cells [CD19+CD27+]) was assessed by flow cytometry (Partec, Nuremberg, Germany); purity was routinely > 90%.
Memory B-cell isolation using FACS
To isolate CD19+CD27+ memory cells by FACS, samples of the PBMC cells were stained for 30 min on ice in a solution of phosphate-buffered saline (PBS, pH 7.4) containing 1% bovine serum albumin along with optimized amounts of fluorescent-conjugated antibodies (5–10 μl for staining 5–10 × 106 cells), according to manufacturer instructions (R&D Systems, Minneapolis, MN). After the incubation, the cells were washed twice to remove unbound antibodies and the cells then underwent FACS analysis in a FACS Aria II system (Becton Dickinson, San Jose, CA). Positive staining was assessed through comparison against isotype-matched stained cells to distinguish non-specific binding from the targeted binding by the test antibodies. Sorting gates were designed based on level of marker expression. To isolate memory B-cells from the PBMC, a total of 40 × 106 events/sample was acquired. All data were analyzed using FlowJo software (Tree Star, San Carlos, CA). A representative dot-plot from the memory B-cell isolation is shown in .
Figure 1. Representative dot-plots of CD19+CD27+ memory B-cells isolated by FACS. To isolate memory cells, PBMC were stained with FITC-conjugated anti-CD19 and PE-conjugated anti-CD27+ antibodies. The positive fraction was determined in comparison with isotype-matched control treated cells. Sorting gates were designed based on levels of marker expression.
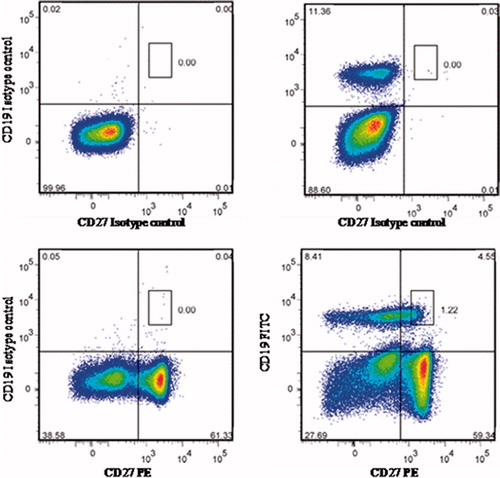
EBV transformation of memory B-cells and limiting dilution assay
An EBV stock was prepared as described previously (Younesi et al. Citation2010). In brief, cell-free supernatant of a 2-week culture of B95.8 cells (NCBI C-110; National Cell Bank, Pasteur Institute of Iran, Tehran, Iran) was passed through a 0.45 μm pore filter. The collected filtrate was centrifuged in an L8–80M ultracentrifuge (Beckmann, Palo Alto, CA) at 30 000 × g for 2 h at 4 °C. Subsequently, the pellets were re-suspended in a small volume of complete culture medium to yield a concentrated viral load and then stored at −80 °C.
For EBV transformation, an EBV aliquot was diluted 10-fold in complete culture medium prior to incubation with the isolated B-cells. A total of 5 × 104 B-cells were re-suspended in 200 μl B.95.8 cell supernatant and incubated for 90 min at 37 °C in a humidified 5% CO2 incubator. Subsequently, the isolated cells were stimulated with 1.25 μg/ml of R848 (TLR7/8 agonist; InvivoGen, San Diego, CA); the optimum concentration of R848 was determined in previous studies (Younesi et al. Citation2014). The EBV-infected cells were then seeded into 96-well microtiter plates at four different densities (10, 100, 250 and 1000 cells/well) on γ-irradiated (6000 rad) human fetal foreskin fibroblasts (HFFF-PI6; NCBI C-170; National Cell Bank – at 15 000/well) or γ-irradiated (3000 rad – at 150 000/well) heterologous PBMC that had been placed in the wells as feeder cells. These fibroblast and PBMC cells had been inactivated and plated at 15 000 and 150 000 cells/well, respectively, in the 96-well microtiter plates overnight before distribution of the EBV-infected B-cells.
In a parallel set of experiments, 1 ml B.95.8 cell supernatant was added to 106 purified memory B-cells (isolated by MACS or FACS) in microtubes and the cells were then incubated for 90 min at 37 °C in a humidified 5% CO2 incubator. Subsequently, the cells were washed twice with RPMI 1640 and then seeded into 96-well microtiter plates at 200, 50, 25 or 10 cells/well – in the presence of feeder layer cells. After 3 weeks, the frequency of transformation was evaluated based on the number of wells containing growing transformed B-cells.
Enumeration of anti-tetanus specific precursor memory B-cells
Frequencies of precursor B-cells that produced antibodies against tetanus neurotoxin (TeNT) were determined by Poisson analysis of limiting dilution microcultures. Following transformation with EBV and R848, different purified B-cells were plated at 10, 25, 50 or 200 cells/well in the presence of the irradiated HFFF.PI6 feeder cells and cultured at 37 °C in a 5% CO2 incubator. The supernatant of the growing B-cells was collected after 1 week and assessed for anti-tetanus antibodies using ELISA.
In brief, tetanus toxin (10 μg/ml; Razi Vaccine and Serum Research Institute) in PBS was coated onto wells of microtiter ELISA plates (Maxisorp, Nunc, Roskilde, Denmark) overnight at 4 °C. The wells were then washed and treated with blocking buffer (PBS-Tween-20 containing 3% non-fat skim milk) for 1.5 h at 37 °C. Aliquots (100 μl) of supernatants were added to wells and the plates incubated for 1.5 h at 37 °C. Thereafter, an appropriate dilution of horseradish peroxidase (HRP)-conjugated rabbit anti-human TeNT (prepared in our laboratory) was added to each well and the plate incubated at 1.5 h at 37 °C. After washing the wells, 3,3′,5,5′-tetramethylbenzidine (TMB) substrate (80 μl) was added to each well and the plate incubated in the dark at room temperature. The reaction in each well was stopped by addition of 50 μl of 1 M H2SO4 and the optical density (OD) in the well was measured at 450 nm using a Multiscan plate reader (Organon, Boxtel, Belgium). The frequency of anti-TeNT precursor-B-cells was calculated by Poisson analysis, as described by Taswell (Citation1981). Wells with OD values greater than the arithmetic mean of the control wells + 3 SD were scored as positive.
Anti-TeNT specific memory B-cells specificity determination
After optimizing the method, to investigate anti-TeNT specific memory B-cell specificity, microtiter polystyrene plates (Maxisorp, Nunc, Roskilde, Denmark) were coated with appropriate concentration (10 μg/ml) of tetanus toxoid, toxin, fragment C and light chain (Sigma) in phosphate-buffered saline (PBS, pH 7.2) and incubated overnight at 4 °C. Plates were then blocked with 1% BSA/PBS for 2 h at 37 °C. After blocking, 100 μl of undiluted anti-TeNT LCL supernatants were added to dedicated wells and the plates were incubated for 1.5 h at 37 °C. The plates were then washed four times, HRP-conjugated anti-human polyclonal antibody (produced in our laboratories) was then added to each well and the plate was incubated for 2 h at 37 °C. A presence of adherent antibodies was detected using TMB and the reaction was stopped by addition of 100 μl of 20% H2SO4. Thereafter, the optical density (OD) value in each well was measured at 450 nm in the plate reader.
Statistical analysis
Statistical analyses were conducted using an SPSS statistical package (v 15.0, SPSS Inc., Chicago, IL). Statistical comparisons of the data were made using Chi-Square and Fisher-Exact tests. p Values < 0.05 were considered significant.
Results
Effects of positive and negative selection on EBV transformation efficiency
Isolation of the B-cells using either the positive or negative selection modes had no effect on their viability (data not shown). Subsequently, B-cells that had been isolated by negative or positive MACS were infected with EBV, stimulated with R848 and serially diluted to 10, 100, 250 and 1000 cells/well in 96-well plates. HFFF.PI6 cells were used as a feeder layer and the transformation process was followed for 3 weeks. illustrates the EBV transformation efficiency for the different conditions. The efficiency of transformation was significantly higher among the negatively-selected cells than the positively-selected B-cells (p = 0.05). R848 caused significant increases in EBV transformation among the positively-selected B-cells (p = 0.007). However, R848 co-stimulation caused significant differences in transformation between the positively- and negatively-selected cells (p = 0.02). In the presence of feeder cells, EBV and EBV/R848 stimulation of the negatively-selected B-cells resulted in higher transformation efficiencies than among positively-selected cells (p < 0.05, ).
Figure 2. Enumeration of EBV-transformed B-cells isolated with negative and positive MACS selection in the presence of R848 and HFF.PI6 feeder layer cells.
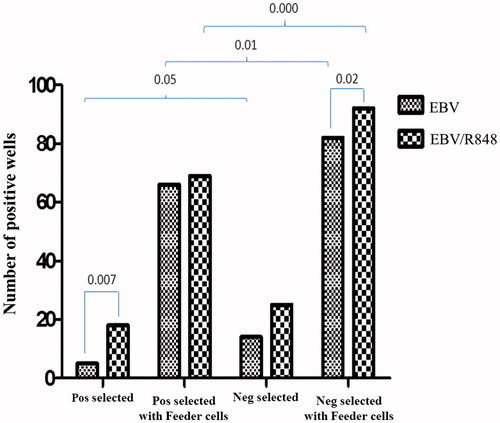
Table 1. Representative data for frequency of EBV transformation of B-cells isolated with negative and positive MACS selection in presence or absence of R848 and feeder layer cells.
Effect of isolation method on frequency of memory B-cell EBV transformation and anti-TeNT producing LCL
To compare the effect of FACS and MACS methods on the transformation efficiency and the frequency of anti-TeNT producing LCL, the purified memory B-cells were infected with EBV and stimulated with R848 and seeded at 10, 25, 50 and 200 cells/well in 96-well microplates. The frequency of EBV transformation was assessed through microscopic observation and the frequency of anti-TeNT producing LCL was assessed through ELISA. As shown in , the transformation efficiency was higher in the MACS-selected memory B-cells than in the FACS-selected memory cells (p < 0.001). However, the frequency of anti-TeNT producing CD19+CD27+ memory B-cells was significantly higher among the FACS-selected B-cells than in the MACS-selected cells (p < 0.0001). Some representative results of the effect of FACS and MACS methods on the transformation efficiency and the frequency of anti-TeNT producing LCL are summarized in .
Figure 3. Enumeration of LCL and TeNT-specific LCL-containing wells of MACS and FACS sorted memory B-cells. Results indicated significant increases in number of TeNT-specific LCL among memory B-cells isolated by FACS compared to among cells isolated by MACS.
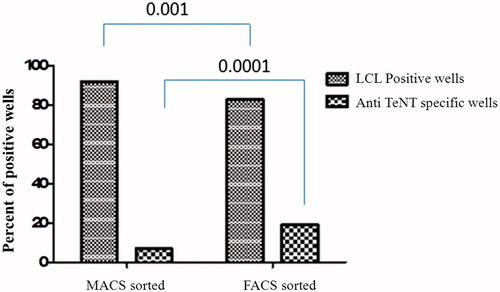
Table 2. Representative data for frequency of LCL and TeNT-specific LCL-containing wells of MACS/FACS sorted memory B-cells transformed with EBV.
Determination of human anti-TeNT-specific memory B-cell specificity
Supernatants from 45 human anti-TeNT-specific memory B-cell cultures that were positive for both anti-tetanus toxin and anti-toxoid were collected. Among these samples, 57.7% (26 out of 45 mAb) were positive for fragment C, 20.0% (nine anti-TeNT-specific memory B-cells) recognized epitopes located in the TeNT light chain, 8.8% (four mAb) were positive for both fragment C and light chain and 13.3% (six mAb) reacted with none of the fragments ().
Table 3. Reactivity of anti-TeNT-specific memory B-cells with different fragments of tetanus toxin.
Discussion
Human monoclonal antibodies (mAb) have emerged as a promising approach for the treatment of several kinds of cancers, autoimmune and infectious diseases (Weiner et al. Citation2010). Transformation of human B-cells with EBV and establishment of immortal antibody-producing B-cells is a valuable method for producing fully human mAb and by-passing time- and cost-consuming chimerization and humanization of murine mAb. However, a low frequency of EBV transformation of peripheral B-cells has been considered a bottleneck among the methods and several attempts have focused on ways to enhance the frequency of EBV-transformed B-cells and the subsequent efficient generation of specific mAb (Beck et al. Citation2001; Oh et al. Citation2003; Traggiai et al. Citation2004; Chen et al. Citation2009). Traggia et al. (Citation2004) had shown that use of a TLR9 agonist (CpG oligonucleotide) significantly increased the frequency of EBV transformation and the efficiency of selection of anti-SARS (severe acute respiratory syndrome) antibody-producing B-cells.
In the present study, the effect of feeder cells (HFFF.PI6) and TLR7/8 agonist (R848) on the transformation efficiency of memory B-cells isolated by different methods (FACS and MACS) was evaluated. The results showed that HFFF-PI6 and R848 significantly increased the transformation efficiency of isolated peripheral B-cells. The study also showed that the efficiency was significantly higher in B-cells purified using negative immunomagnetic selection than by those using positive selection. It could be postulated that, due to the attached CD19-conjugated magnetic beads on the B-cells, the positively-selected cells had less capability to undergo EBV transformation compared to negatively-selected counterparts. However, Hur et al. (Citation2005) reported that attachment of anti-CD19 polyclonal antibodies to B-cells during MACS-mediated positive selection increased expression of cytosolic (but not membranous) LMP-1 proteins that have a critical role in EBV transformation of B-cells. It is also possible the binding of CD19-beads may have induced conformational changes that disrupted the EBV ability to infect the cells via CD21, as both molecules are part of the B-cell receptor complex. This difference, due to introduction of the CD19-modified bead, remains to be thoroughly investigated.
The present study also examined the effects on EBV transformation efficiency of B-cells as a result of being isolated by FACS or MACS. The results showed that the transformation efficiency of peripheral total B-cells was significantly higher than that of memory B-cells isolated by FACS and MACS. However, the frequency of anti-TeNT-producing LCL was significantly higher in FACS-isolated memory B-cells than in MACS-isolated memory cells. Wrammert et al. (Citation2008) have shown that, 21 days after immunization with influenza virus, the frequencies of antibody-secreting (CD19+CD27highCD38high) and memory (CD19+IgG+CD27+) B-cells were increased from baseline and reached, respectively, 6.4% and 1% of the total B-cells. Those authors also showed that the frequency of influenza-specific B-cells was significantly higher in CD27high B-cells compared to among IgG+ B-cells and memory B-cells. In the current study, use of FACS compared to MACS led to CD27high B-cell isolation (see ). Thus, is was not unexpected that the frequency of antibody-secreting cells and of antigen-specific B-cells was more evident in the FACS isolated fractions. Furthermore, the current results showed that, with humans, the majority of memory response was directed against fragment C. Given that the receptor binding domain and critical residues for TeNT binding are located in fragment C, this portion of the tetanus toxin carboxy-terminal heavy chain (≈50 kDa) is considered an immunodominant and an immunoprotective domain of TeNT.
It is important to note for the readers that, although this improved method led to the production of anti TeNT-specific mAb, these methods have very low efficiency for large-scale antibody production. Occasionally, utilization of EBV transformation results in limited success in mAb production; therefore, other strategies have been developed – including antibody engineering, display technology and producing mAb using transgenic mice (Traggiai et al. Citation2004).
Conclusions
The present findings indicated that isolation of memory B-cells using the FACS technique, as well as the employment of feeder layers and TLR7/8 agonists, could significantly increase the efficiency of EBV transformation and result in a higher frequency of immortalized antigen-specific B-cells. Further investigations are needed to provide insight regarding the mechanisms by which FACS and MACS isolation methods might influence the biological properties of these human B-cells.
Acknowledgments
The authors would like to thank Mogam Biotechnology Institute (Yongin, South Korea) for their technical assistance.
Disclosure statement
The authors report no conflicts of interest. The authors alone are responsible for the content and writing of the paper.
References
- Babcock GJ, Decker LL, Volk M, Thorley-Lawson D. 1998. EBV persistence in memory B-cells in vivo. Immunity 9:395–404.
- Beck JC, Beiswanger CM, John EM, Satariano E, West D. 2001. Successful transformation of cryopreserved lymphocytes a resource for epidemiological studies. Cancer Epidemiol Biomarkers Prevent. 10:551–554.
- Bourke E, Bosisio D, Golay J, Polentarutti N, Mantovani A. 2003. The toll-like receptor repertoire of human B-lymphocytes: inducible and selective expression of TLR9 and TLR10 in normal and transformed cells. Blood 102:956–963.
- Chen C, Johnston T, Jeon H, Gedaly R, McHugh P, Ranjan D. 2009. Cyclosporine promotes Epstein–Barr virus-infected human B-cell transformation assayed by three correlated assay methods. Transplant Proc. 1:366–370.
- Dainiak MB, Kumar A, Galaev IY, Mattiasson B, editors. 2007. Methods in cell separation. Cell separation. London: Springer. p. 1–18.
- Hur DY, Lee MH, Kim JW, Kim JH, Shin YK, Rho JK, Kwack KB, Lee WJ, Han BG. 2005. CD19 signalling improves the Epstein-Barr virus‐induced immortalization of human B-cells. Cell Prolif. 38:35–45.
- Iskra S, Kalla M, Delecluse HJ, Hammerschmidt W, Moosmann A. 2010. Toll-like receptor agonists synergistically increase proliferation and activation of B-cells by EBV. J Virol. 84:3612–3623.
- Manor E. 2008. Human plasma accelerates immortalization of B-lymphocytes by Epstein-Barr virus. Cell Prolif. 41:292–298.
- Middeldorp JM, Brink AA, van den Brule AJ, Meijer CJ. 2003. Pathogenic roles for Epstein-Barr virus (EBV) gene products in EBV-associated proliferative disorders. Crit Rev Oncol Hematol. 45:1–36.
- Oh HM, Oh JM, Choi SC, Kim SW, Han WC, Kim TH, Park DS, Jun CD. 2003. An efficient method for the rapid establishment of Epstein‐Barr virus immortalization of human B-lymphocytes. Cell Prolif. 36:191–197.
- Pelloquin F, Lamelin JP, Lenoir GM. 1986. Human B-lymphocyte immortalization by Epstein-Barr virus in the presence of cyclosporin A. In Vitro Cell Devel Biol. 22:689–689.
- Price AM, Luftig MA. 2013. Dynamic Epstein–Barr virus gene expression on the path to B-cell transformation. Adv Virus Res. 88:279–313.
- Shokrgozar MA, Shokri F. 2001. Enumeration of hepatitis B surface antigen‐specific B-lymphocytes in responder and non‐responder normal individuals vaccinated with recombinant hepatitis B surface antigen. Immunology 104:75–79.
- Steinitz M, editor. 2014. Production of human monoclonal antibodies by the Epstein-Barr virus method. Human monoclonal antibodies. London: Springer. p. 111–122.
- Taswell C. 1981. Limiting dilution assays for the determination of immunocompetent cell frequencies. I. Data analysis. J Immunol. 126:1614–1619.
- Tjandra JJ, Ramadi L, McKenzie IF. 1990. Development of human anti-murine antibody (HAMA) response in patients. Immunol Cell Biol. 68:367–376.
- Traggiai E, editor. 2012. Immortalization of human B-cells: Analysis of B-cell repertoire and production of human monoclonal antibodies. Antibody methods and protocols. London: Springer. p. 161–170
- Traggiai E, Becker S, Subbarao K, Kolesnikova L, Uematsu Y, Gismondo MR, Murphy BR, Rappuoli R, Lanzavecchia A. 2004. An efficient method to make human monoclonal antibodies from memory B-cells: potent neutralization of SARS coronavirus. Nat Med. 10:871–875.
- Weiner LM, Surana R, Wang S. 2010. Monoclonal antibodies: Versatile platforms for cancer immunotherapy. Nat Rev Immunol. 10:317–327.
- Wrammert J, Smith K, Miller J, Langley T, Kokko K, Larsen C, Zheng NY, Mays I, Garman L, Helms C, et al. 2008. Rapid cloning of high-affinity human monoclonal antibodies against influenza virus. Nature 453:667–671.
- Younesi V, Nikzamir H, Yousefi M, Khoshnoodi J, Arjmand M, Rabbani H, Shokri F. 2010. Epstein Barr virus inhibits the stimulatory effect of TLR7/8 and TLR9 agonists but not CD40 ligand in human B-lymphocytes Microbiol Immunol. 54:534–541.
- Younesi V, Shirazi FG, Memarian A, Amanzadeh A, Jeddi-Tehrani M, Shokri F. 2014. Assessment of the effect of TLR7/8, TLR9 agonists and CD40 ligand on the transformation efficiency of Epstein-Barr virus in human B-lymphocytes by limiting dilution assay. Cytotechnology 66:95–105.
- Young LS, Rickinson AB. 2004. Epstein-Barr virus: 40 years on. Nat Rev Cancer. 4:757–768.
- Yousefi M, Khosravi-Eghbal R, Mahmoudi AR, Jeddi-Tehrani M, Rabbani H, Shokri F. 2013a. Comparative in vitro and in vivo assessment of toxin neutralization by anti-tetanus toxin monoclonal antibodies. Hum Vac Immunother. 10:16–15.
- Yousefi M, Tahmasebi F, Younesi V, Razavi A, Khoshnoodi J, Bayat AA, Abbasi E, Rabbani H, Jeddi-Tehrani M, Shokri F. 2013b. Characterization of neutralizing monoclonal antibodies directed against tetanus toxin fragment C. J Immunotoxicol. 11:28–34.