Abstract
Vanadium (V) is a transition metal often adhered to particulate matter and released into the atmosphere as vanadium pentoxide (V2O5) by the burning of fossil fuels. This air pollutant causes adverse effects in the immune system. Lymphocytosis and splenomegaly have been reported with increased white pulp in mice after V inhalation. The effect of V on the immune system as related to sex has been poorly investigated. This study sought to determine if V inhalation (a) produced lymphoproliferation that could explain the changes previously observed in the spleen and in peripheral blood lymphocyte counts and (b) whether any observed effects differed due to gender. Immunohistochemical analyses of Ki-67, a specific proliferation marker, was made in the spleens of CD-1 male and female mice exposed for 1 h, twice a week, over a 12-week period to V2O5 (at 1.4 mg V2O5/m3) by whole-body inhalation; similar analyses were performed on spleens of control mice exposed to vehicle (filtered air). The results showed that in male mice there was a significant increase in percentage of Ki-67 immunopositive lymphocytes starting from the second week and until the end of the exposure. The Ki-67 signal was cytoplasmic and nuclear in the exposed males, while in controls the signal was only nuclear. In female mice, V inhalation singificantly increased the percentage of proliferating lymphocytes only after 1 week of exposure. Ki-67 signal was observed only in the nucleus of lymphocytes from the control and exposed females. The results here help to explain the splenomegaly and lymphocytosis observed previously in male mice and support the lymphoproliferative effect induced by V. Lastly, the finding that there was a sex difference in the effect of vanadium on lymphocyte proliferation suggests a role for sex hormones in potential protection against V immunotoxicity; however, further studies are needed to support this hypothesis.
Introduction
Air pollution is an important health problem worldwide. Particulate matter (PM) is one of the most important air pollutants in urban centers and moreso in megacities (Jahn et al. Citation2011; Calderon-Garciduenas et al. Citation2015). PM is a complex mixture of small particles and liquid droplets made up of acids, nitrates, sulfates, elemental and organic carbon, organic chemicals (e.g. polycyclic aromatic hydrocarbons), biologics (e.g. endotoxins, cell fragments), metals (e.g. iron, copper, nickel, zinc and vanadium) and dust particles (Kim et al. Citation2015). Several studies have shown the relationship between air pollution/PM and pathologies that involve alterations in the immune system and related responses, including; asthma and others allergic diseases, airway inflammation, acute respiratory infections, chronic obstructive pulmonary diseases, lung cancer, among others (WHO Citation2014; Gass et al. Citation2015).
Vanadium is an important transition metal often found adhered to PM and released into the atmosphere from fuel products combustion as vanadium pentoxide (V2O5), the most toxic compound of vanadium (Hope Citation2008; Fortoul et al. Citation2014a,Citationb). Vanadium (V) is adsorbed into PM and by inhalation reaches the systemic circulation exerting its toxic effect in other organs and tissues (Dockery & Pope Citation1994; Barceloux Citation1999). Adverse effects by V exposure in reproductive, nervous, urinary, respiratory and hematopoietic systems have been reported (Aragon et al. Citation2005; Gonzalez-Villalva et al. Citation2009; Fortoul et al. Citation2011, Citation2014a; Rodriguez-Lara et al. Citation2013b; Walters et al. Citation2014). Nevertheless, the effects of V on the immune system are not very well studied and many of the reported results have often been contradictory. For example, V (NH4VO3) exposure reduces cell immune responses by inhibiting interferon (IFN)-γ production and/or release of other cytokines (Di Gioacchino et al. Citation2002), reducing binding to/or decreasing IFN-γ Class I receptors expression (Cohen et al. Citation1996) and inhibiting β-defensin gene expression (Klein-Patel et al. Citation2006); taken together, these outcomes contribute to the overall mechanism of V-induced immunosuppression (Zwolak Citation2014). In this context, dietary V (NH4VO3) in excess of 30 mg/kg was seen to reduce the number of the IgA+ cells and change the contents of the cytokines in the cecal tonsil (and so impact on local mucosal humoral immunity) in broilers (Deng et al. Citation2012). Moreover, this exposure reduced ileal T-lymphocyte populations and the percentages of T-lymphocyte sub-sets as well as host levels of interleukin (IL)-2, IL-6 and IFNγ. Taken together, this showed that the immune function of the local intestinal mucosa of broilers could be affected by vanadium (Wang et al. Citation2012). Conversely, other studies had shown that V could stimulate immune activity, including increasing production of inflammatory cytokines (IL-6, IL-8 and tumor necrosis factor [TNF]-α) that each can contribute to V-related inflammation/immunostimulation in exposed hosts (Zwolak Citation2014).
Studies from our laboratory previously showed that chronic inhalation of V (as V205) by CD1 male mice induced splenomegaly accompanied by histological changes such as poorly delimited and decreased red pulp size and increases in white pulp size (Pinon-Zarate et al. Citation2008). In these same hosts, splenic lymphatic nodules were increased in number and size and there were increases in hyperplastic germinal centers containing an increased number of CD19+ B-lymphocytes. These changes in the spleen after V exposure appeared to result in severe effects on the humoral immune response, since the avidity and antibodies levels to Hepatitis B-Ag in the exposed mice was lower compared to values in control counterpart mice (Pinon-Zarate et al. Citation2008).
With the same inhalation model, V also caused an increase in leukocyte, neutrophil and lymphocyte levels in the peripheral blood (Gonzalez-Villalva et al. Citation2009). Further, such exposures produced genotoxic damage (e.g. single-strand brakes and micronucleus formation) in peripheral blood lymphocytes of male mice (Fortoul et al. Citation2011). It was also noted that lymphocytes from the peripheral blood and lymphoid tissues (e.g. spleen and thymus) exhibited morphological nuclear changes (i.e. deep invaginations and evaginations, multilobulations, nuclear pseudoinclusions, chromatin redistribution, changes in euchromatin/heterochromatin patterns, increased nucleolar size/number) (Rodriguez-Lara et al. Citation2013a) similar to those often seen in malignant cells (Zink et al. Citation2004). It was also noted that the percentage of lymphocytes with nuclear changes increased with the length of V total exposure (Rodriguez-Lara et al. Citation2013a).
All those changes observed in the spleen (i.e. splenomegaly, increased white pulp) and pheriperal blood (lymphocytosis) suggested to us that V inhalation might induce lymphocyte activation and proliferation. This would present an interesting conundrum; what might be implications of increased activation and proliferation among such cells that are also increasingly exhibiting nuclear abnormalities and genetic damage.
Because V exposure appears to produce a lymphoproliferative disorder and due to structural and functional changes in spleen lymphocytes in our mouse model, this work sought to determine if V inhalation causes changes in lymphocyte proliferation in the spleen in situ, and, if so, if this effect could differ by sex. This latter aspect of the study was undertaken as it has been reported that immune responses could be different by gender in normal and in pathological conditions (Ngo et al. Citation2014; Vrachnis et al. Citation2014) and, more specifically, toxic effects of metals (including V) on the immune system of females (models) have been poorly investigated.
Materials and methods
Animals and inhalation protocol
CD-1 male and female mice (24 of each sex, 33–35 g, 8-weeks-of-age) were obtained from the Medical School at Universidad Nacional Autonoma de Mexico (México City, México). Upon arrival, all mice were housed separately by sex in hanging plastic cages (six mice/cage) under controlled light conditions (12-h light/dark) in a pathogen-free facility maintained at 21 °C with 55–60% relative humdity. All mice had ad libitum access to standart rodent lab chow 5001 LabDiet (Ralston Purina, St. Louis, MO) and filtered tap-water. The mice were managed according to the Mexican official norm NOM-062-ZOO-1999 for the production, care and use of laboratory animals. The experimental protocol was in accordance with the Animal Act of 1986 for Scientific Procedures.
Inhalation exposures were performed as described by Fortoul et al. (Citation2009). Twelve 1-month-old male and 12 1-month-old female mice were randomly placed (separately by sex) in an acrylic box (45 cm × 21 cm × 35 cm) and exposed (at 10 L/min flow rate) to an atmosphere containing V2O5 (99.99% purity, Sigma, St. Louis, MO) for 1 h/day, twice a week, for 12 weeks. At the same time, 12 1-month-old male and 12 1-month-old female mice were placed (separately by sex) in an acrylic box and exposed to only vehicle (saline) for the same period of time (control group). An ultra-nebulizer (DeVilbiss Ultraneb 99, Somerset, PA) was used to nebulize a V2O5 suspension (0.02 M) at a constant flow rate (10 L/min); based on information provided by the nebulizer manufacturer, it was expected that ≈80% of the aerosolized particles that reached the mice would have a mass median aerodynamic diameter (MMAD) of 0.5–5.0 μm.
Vanadium levels in the chamber were quantified based on measurements of V2O5 deposited on filters that had been positioned at the external outlet of the chamber during the exposure period. After each day’s exposure was completed, the filters (n = 6/exposure) were removed, weighed and evaluated using a Perkin Elmer Model 2380 graphite furnace Atomic Absorption Spectrometer (Perkin Elmer, Waltham, MA) (Fortoul et al. Citation2002) set at 318.4 nm; the detection limit was 0.37 ppm and the slit 0.7 nm. Formaldehyde and blanks were also analyzed to identify any metal contamination in the measurements. Accuracy was assured by three random determinations of different standard solutions. Each filter sample was analyzed in triplicate. Based on repeated measurements over the course of the study (and in trial runs), the final in the chamber exposure was calculated to be 1.4 mg V2O5/m3 (as in Fortoul et al. Citation2002).
For all subsequent analyses described herein, sets of three exposed mice and three controls were euthanized 24 h after the final exposure of the first week of inhalation exposures and also 24 h after the completion of a 4-, 8- or 12-week exposure series. At sacrifice, mice were injected with sodium pentobarbital (100 mg/kg, intraperitoneal) and then had their chest cavity opened. Each mouse was then perfused via the aorta with saline, followed by 4% paraformaldehyde in phosphate buffer (pH 7.4). In each case, the spleen was resected and placed in fixative solution for 2 h and subsequently processed for immunohistochemistry.
Immunohistochemistry
Tissue sections (5-μm) were placed in poly-l-lysine-coated slides (Sigma). Tissues were dewaxed at 56 °C for 30 min and placed in xylene for 5 min. Subsequently, tissues were hydrated in decreasing concentrations of ethanol (absolute and 96%) and distilled water. Antigen retrieval was achieved by incubation in Declere 10% (Cell Marque; Sigma, Rocklin, CA) at 15 psi for 3 min, after which the slides were washed in phosphate-buffered saline containing 10% Triton-X-100 (PBS-Triton) (Sigma). Endogenous peroxidase was blocked by placing the slides in 3% hydrogen peroxide solution (H2O2, J.T. Baker, Phillipsburg, NJ) for 30 min. The sections were then rinsed twice with PBS-Triton and placed for 10 min in a PBS-albumin-Triton (0.1% [w/v] albumin, 10% Triton-X) solution (MP Biomedicals, Santa Ana, CA). The sections were then incubated for 1 h at 37 °C in buffer containing rabbit polyclonal antibody to Ki-67 (Abcam, Cambridge, MA) diluted 1:200 in PBS-albumin-Triton. Ki-67 is a widely accepted proliferation marker; it is strictly associated with cell proliferation due to it being expressed during all active phases of the cell cycle (G1, S, G2 and mitosis), but absent in resting cells (G0) (Scholzen & Gerdes Citation2000; Muñoz et al. Citation2003).
The sections were then washed in PBS-Albumin-Triton and incubated for 30 min at 37 °C with biotynilated goat-anti-rabbit secondary antibody (Santa Cruz Biotechnology, Santa Cruz, CA) diluted 1:400 in PBS-albumin-Triton solution. Finally, the sections were rinsed several times in PBS-Triton and incubated for 30 min at 37 °C in horseradish-peroxidase-streptavidin complex (DAKO, Carpinteria, CA). Immunoreactivity was visualized by incubation of the slides in a solution of 0.05% [w/v] 3,3′-diaminobenzidine tetrahydrochloride (Zymed Laboratories Inc, San Francisco, CA). Sections were counterstained slightly with hematoxylin and then mounted. Samples lacking primary antibody were also included as negative controls.
Immunoreactivity evaluation
Tissue samples were observed in a BX51 light microscope (Olympus América, México City) coupled to a digital camera (Evolution MP Color, Media Cybernetics, Rockville, MD). Images were obtained from five randomly-selected tissue areas (76.241 mm2) at 100× magnification. Evaluation of Ki-67 immunoreactivity in lymphocytes was performed in the white pulp. The presence of an ochre color was considered positive as the developer for the reaction was diaminobenzidine. Ki-67 signal is usually observed in the nucleus of proliferating (during G1, S and G2 phases) but non-mitotic normal cells and on the chromosomal surface in mitotic cells (Sholzen & Gerdes Citation2000). In some exceptional conditions, Ki-67 signal has also been detected in the cytoplasm of proliferating cells during the interphase (Grzanka et al. Citation2000; Faratian et al. Citation2009). For this reason, here, both cytoplasmic and nuclear signals were considered as positive if stain was identified. For each field, the percentage of immunopositive cells for Ki-67 was calculated (based on counts of total cells and Ki-67+ cells) using the Image J program (NIH, Bethesda, MD). The mean of assesed cells was 7133 per group (control/exposed after 1, 4, 8 and 12 weeks).
Statistical analysis
Statistical analysis was conducted using an analysis of variance (ANOVA) to evaluate the differences in the effect of V on the Ki-67 immunopositive cells in the fields. A Tukey’s post-hoc test was applied to determine if differences between controls and exposed mice were statistically significant (p < 0.05). Data collected from 12 control individuals that were employed throughout the inhalation scheme were pooled and compared with those collected from the groups of individuals exposed to V (both females and male mice). Finally, an ANOVA and a Holm-Sídák multiple comparations post-hoc test was used to determinate if V effects on lymphocyte proliferation was different among sexes during the exposure time. All analyses were performed using a Prism software package (v.4.0c, Graph-Pad, La Jolla, CA).
Results
To investigate potential mechanisms by which atmospheric V might produce inmmunodysfunction and building upon our previous observations about effects of V on the immune system, effects of inhalation of V (as V2O5) on in situ lymphocyte proliferation were evaluated by Ki-67 positive signal by immunohistochemistry. In general, the results showed that V inhalation produced lymphoproliferation in the spleen of the mice and that the effect was dependent of length of exposure time and differed by gender.
In control male mice, a basal percentage of lymphocytes immunopositive to the proliferation marker Ki-67 was observed (23.05%). The Ki-67 signal was observed mainly in the cell nucleus (see ). After 1 week of V inhalation, this percentage slightly increased (28.21%) (); however, after 4, 8 and 12 weeks of exposure, there was a significant increase in proliferating splenic lymphocytes compared with the tissues of the control mice. The percentages of immunopositive cells at these exposure times were, respectively, 38.86, 41.75 and 41.91% (). For male mice, the lymphoproliferative effect produced by V was dependent on the length of total exposure ().
Figure 1. Effect of inhaled vanadium on percentage of Ki-67 immunopositive lymphocytes in male mice. Representative figures are presented. (A) Control mouse spleen shows slight signal to Ki67, mainly in the nucleus. Ki-67 signal increases with exposure time – after 1, 4, 8 and 12 weeks of exposure – as shown in, respectively (B, C, D and E). The signal in exposed groups is apparent both in the nucleus and cytoplasm. (F) The increase in lymphoproliferation was significant after 4 weeks and then until the end of exposure (*p < 0.05 ANOVA, Tukey’s post-hoc test).
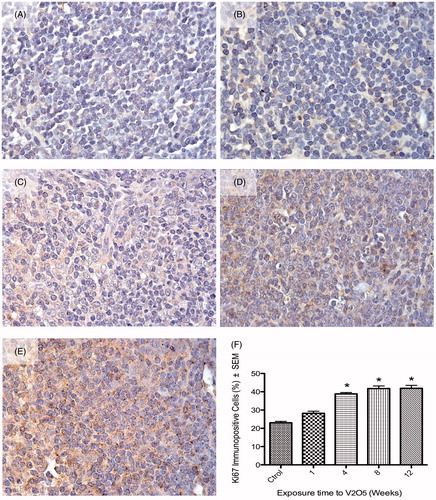
It was interesting to note that in male V-exposed mice, the Ki-67 signal was frequently exhibited in the nucleus and cytoplasm of proliferating (G1, S or G2), but non-mitotic lymphocytes. In control mice, Ki-67 signal was observed only in the nucleus of proliferating (G1, S or G2), but non-mitotic lymphocytes. Female mice displayed a different behavior compared to the males. Control mice had a basal percentage of Ki-67+ lymphocytes of 30.55% (). After 1 week of exposure, there was a significant increase to 34.87% Ki-67+ lymphocytes (). However, subsequent continuing exposures did not produce significant changes in the percentage of proliferating cells. The percentage Ki-67+ lymphocytes after 4, 8 and 12 weeks of exposure was 29.75, 32.27 and 32.5%, respectively (). The Ki-67 signal in the female splenic lymphocytes was always nuclear in non-mitotic proliferating cells (G1, S or G2) (in control and V-exposed hosts; ). Representative images of nuclear and cytoplasmic positive signals for Ki-67 in lymphocytes from controls and exposed mice are shown in .
Figure 2. Effect of inhaled vanadium on the percentage of Ki67 immunopositive lymphocytes in female mice. Representative figures are presented. (A) Control mouse spleen shows Ki-67 nuclear signal in a low percentage of lymphocytes. (B) After 1 week of exposure, the percentage of Ki-67+ lymphocytes significatively increased (compared to control) After 4, 8 and 12 weeks of exposures, the percentage of immunopositives lymphocytes did not significantly increase (C, D, E and F) (*p < 0.05 ANOVA, Tukey’s post-hoc test). Ki-67 signal was only seen in nuclear areas in both control and exposed mice.
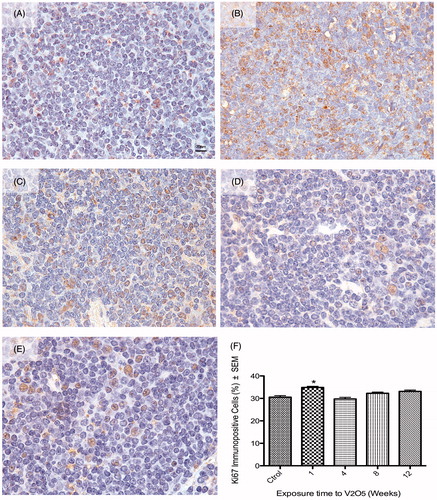
Figure 3. Differences between nuclear and cytoplasmic Ki-67 signal in lymphocytes from male and female mice. (A) Lymphocytes from exposed male mice (4 week exposure) show a cytoplasmic (arrowhead) positive signal (ochre color) to Ki-67, while the nucleus (purple) from these cells were negative for this marker. (B) Lymphocyte from exposed female mice (4 week exposure) shows a positive nuclear staining to Ki-67 (arrowhead) while the cytoplasm was clearly negative for the marker.
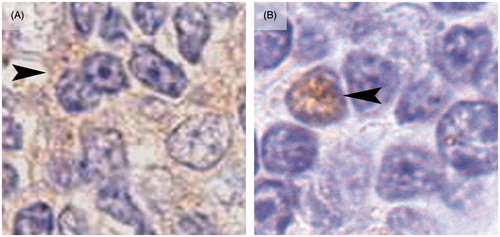
Vanadium effects on the proliferation of spleen lymphocytes clearly differed between sexes (). By comparing and , it can also be seen that the spleens from control females had a higher percentage of proliferating lymphocytes compared to spleens of control male mice. Further, after 1 week of V exposure, female mice had a significant increase in lymphocyte proliferation compared to male mice. Oddly, while the V effect on cell proliferation was observed earlier in females than in male mice, the effect was gone regardless of exposures longer than this. In contrast, male mice showed a significant increase in lymphocyte proliferation after 4 weeks of exposure and this continued until the end of the exposure protocol.
Figure 4. Lymphoproliferative effect of V inhalation in male vs female mice. Spleens from control female (F) mice had a significantly higher percentage of proliferative lymphocytes compared to control male (M) mice. Male mice exhibited a significant increase in spleen lymphoproliferation from week 4 of exposure until the end of the experiment. In comparison, female (F) mice only presented a significant increased in proliferation after 1 week of V exposure. (*p < 0.05 ANOVA, Holm-Sídák’s post hoc test).
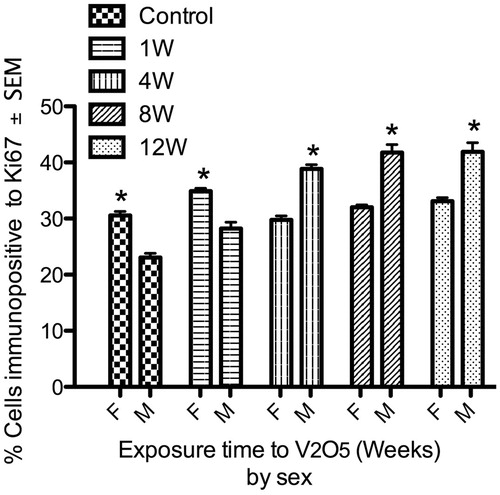
Discussion
Inhalation of air pollution particulate matter (PM) has been associated with immune system dysfunction. Vanadium (V), a transition metal often found adhered to PM, has been shown to have “contradictory” effects on the immune system; furthermore, the mechanisms of these effects are not well understood and the responses as a function of host sex have been poorly investigated. Our previous studies suggested that V produced a lymphoproliferative disorder in the spleen. Results from the present study demonstrated that V inhalation produced an increase in in situ lymphocyte proliferation in the spleens of male mice. It was also observed that the signal distributions for Ki-67 in the spleens were affected by the exposures. Interestingly, and in contrast, female mice appeared to be less susceptible to these proliferative effects of V compared to the males; a significant increase in lymphocyte proliferation only began to occur after 1 week of exposure and then increased no further over the 12-week exposure period. Further, the Ki-67 signal in spleens of female mice remained localized in the cell nucleus, regardless of whether the hosts were exposed to V or not.
As previously reported (Gonzalez-Villalva et al. Citation2011; Ustarroz-Cano et al. Citation2012) the concentration used in this experimental model was in the range of that found (0.01–60 mg/m3) in many workplaces according to World Health Organization (WHO Citation2000). However, the value here was higher than that reported in workplaces (ranging from 0.2–0.5 mg/m3) by IARC (Citation2006). As stated by the Occupational Safety and Health Administration (OSHA), the Threshold Limit Value (TWA) for V2O5 exposure is 1 mg/m3, the Threshold Limit Value Short-term Exposure Limit (TLV-STEL) is 3 mg/m3 and the Permissible Exposure Limit (PEL) is 0.1mg/m3. For the National Institute of Occupational Safety and Health (NIOSH), the Recommended Exposure Limit (REL) for V2O5 exposure is 0.05 mg/m3 (15 min) (OSHA Citation2012). However, in some workplaces exposure limits are exceeded mainly in boiler cleaning places (60 mg/m3) (WHO Citation2000).
Outside of occupational settings, V is released into the air by combustion of V-rich fuel oils and from petroleum processing, particularly materials from Kuwait, Iran, Iraq, Venezuela and Mexico (Fortoul et al. Citation2002). High V concentrations (> 600 mg/kg) have been detected in soils in the vicinity of petrochemical plants in Mexico as a consequence of airborne particulate emissions generated by extensive of oil/fuel burning (Hernandez & Rodriguez Citation2012). V is also present in products released by burning of residual (heavy) fuel oils and V can be encountered at concentrations of 10–50 mg/g fly ash particulate (IARC Citation2006). Thus, the concentration of V used in the present experimental model would reflect V exposures that occur routinely among citizens of many urban centers, including Mexico City (Fortoul et al. Citation2002) and in some occupational settings.
The present results support a proliferative effect from V observed by others. Qureshi et al. (Citation1999) showed that oral administration of ammonium metavanadate (NH4VO3 at 10, 25 or 50 mg/kg) to chicks produced an increment of CD4+ cells in the blood and spleen. This same compound at 0.15 mg V/mL in drinking water caused a significant increase in circulating neutrophil and lymphocyte counts in 2-month-old Wistar rats of both sexes (Zaporowska & Wasilewski Citation1992). In earlier studies that used the same V inhalation model as employed here, an increase in leukocytes, neutrophils and lymphocytes in the peripheral blood (Gonzalez-Villalva et al. Citation2009), as well as an increased megakaryocyte proliferation (Fortoul et al. Citation2008; Gonzalez-Villalva et al. Citation2014) were noted.
The potential mechanisms by which V might cause lymphocyte proliferation are diverse. Vanadium produces an inflammatory microenvironment, as it triggers an increase in the local production of cytokines such as tumor necrosis factor (TNF)-α and of interleukins (IL)-1, -2 and IL-6 (Huang et al. Citation2001). Several of these same cytokine are also important in the process of lymphocyte proliferation. Some vanadium salts (e.g. VOSO4) in vitro also can up-regulate genes associated with proliferation, including: AP-1, Ras, C-Raf-1, MAPK, p53 and NF-κB (Pandey et al. Citation1999; Ye et al. Citation1999; Mukherjee et al. Citation2004; Li et al. Citation2005). Due to the chemical/structural similarity between vanadate and phosphate ions, V is also able to stimulate cell cycle pathways by activating mitogens and kinases such as Syk family, ERK1/2, MAPK and PI3K (Krejsa et al. Citation1997; Morinville et al. Citation1998; Wang & Bonner Citation2000). Of these, ERK 1/2 is a significant pathway in lymphocyte activation processes (Miyaji et al. Citation2009). Moreover, V can also induce NFAT activation through calcium-/H2O2-generation-related pathways. This is important in that NFAT is a pivotal transcription factor responsible for regulation of IL-2 growth factor necessary for lymphocyte clonal expansion (Huang et al. Citation2001). Signaling through the IL-2 receptor (IL-2R) involves phosphorylation of several proteins, including some components of the JAK/STAT, JAK3 and STAT5 families. Activation of JAK/STAT (which promotes proliferation) has been reported in megakaryocytes in mice exposed to V2O5 inhalation (Gonzalez-Villalva et al. Citation2014). It is quite probable that V might activate the JAK/STAT pathway in lymphocytes and stimulate proliferation; while this has also been noted in megakaryocytes in the same inhalation model, this hypothesis still remains to be validated.
Some V compounds, such as sodium oxodiperoxo (1,10-phenanthroline) vanadate (V) (pV(phen)), bis(maltolato)-oxovanadium(IV) (BMOV), sodium orthovanadate (Na3VO4) and bisperoxovanadium (bpV) act as phosphotyrosine phosphatase (PTP) inhibitors; this class of inhibitors are known to be potent activators of T-lymphocytes (Krejsa et al. Citation1997; Barat & Tremblay Citation2003). BMOV and pV(phen) strongly activated NF-κB, outcomes that are both essential for T-lymphocyte activation and B-lymphocyte differentiation.
Inhalation of V2O5 also causes nuclear changes and chromatin redistribution in splenic lymphocytes (Rodriguez-Lara et al. Citation2013a); these result from reduced expression of nucleoskeletal proteins (i.e. nuclear lamins A/C, B1 and B2) (Rodriguez-Lara Citation2008), as has been reported for their cytoplasmic counterpart (Rodriguez-Lara et al. Citation2013b). Moreover, our laboratory has previuosly reported increases in genotoxicity within peripheral blood lymphocytes of V-exposed hosts (Fortoul et al. Citation2014a). These nuclear and chromatin changes, as well as DNA damage, could result in alterated genetic expression patterns that could guide uncontrolled cell proliferation.
Apart from these observations about effects from the inhaled V on the proliferation of splenocytes in situ, a key outcome of the present study was the observation that the effects of V differed by sex, i.e. male mice were more susceptible than the females to the proliferative effect. Among the various means by which V could induce these effects, as noted above, is through induction of oxidative stress and of transcription factors (i.e. NF-κB) activated by oxidative stress (Nandakumar et al. Citation2008; Morgan & Liu Citation2011). Based upon a variety of studies, it appears that female hosts tend to have stronger anti-oxidant defense responses than their male counterparts (Borras et al. Citation2003; Badeau et al. Citation2005; Vina et al. Citation2011; Mancini et al. Citation2013). For example, it has been reported that mitochondria from Wistar rats of control females produced only half of the levels of peroxides compared with males and ovariectomized females (Borras et al. Citation2003). Interestingly, when estrogens were administered daily for a month subcutaneosly (at 1 μg/kg body weight) to male and ovariectomized females, protective effects against mitochondrial free radical production were noted. In addition, control females had higher levels of endogenous antioxidants such as Mn-superoxide dismutase and glutathione peroxidase compared with the males and ovariectomized females. These results support a protective activity of estrogens against oxidative stress. Based on their chemical structure, i.e. the presence of a phenolic ring that is ready to donate electrons (Amantea et al. Citation2005; Badeau et al. Citation2005), estrogens can function as free radical “scavengers”. This suggests to us that the presence of the estrogen anti-oxidant defense in females could underlay the differences in effects on lymphocyte proliferation seen after V exposure.
It was of interest that, under normal conditions, control female mice exhibited a higher percentage of lymphocyte proliferation in situ compared to control males. It has been observed in the past that the immune response differed by sex and only more recently has the role of sex hormones been investigated (Straub Citation2007; Scotland et al. Citation2011). For example, in pathological states such as severe sepsis, this difference in immune response confers a survival advantage in females over males (Scotland et al. Citation2011). Estrogens could modulate the immune response and the proliferation of lymphocytes due to a mitogen-like effect. Further, it is known that 17β-estradiol can directly influence the synthesis and signal transduction of multiple cytokines in vitro, including IL-8, IL-12, IL-1, IL-4 IL-10 and TNFα, and that such activation or inhibition depends on the 17β-estradiol concentration and cell type impacted (Straub Citation2007). The current observations provide further support for the hypothesis about differences in immune response by sex, in this case in normal hosts and those undergoing the stress of V exposures.
Finally, this study documented shifts in the expression site for Ki-67 in V-exposed hosts. In control males, the protein was seen to reside mainly in the cell nucleus of proliferating (G1, S or G2) but non-mitotic lymphocytes as it has been reported in normal conditions for this proliferation marker (Scholzen & Gerdes Citation2000). In contrast, in exposed males, the signal of Ki-67 was found often in both the nucleus and cytoplasm of proliferating non-mitotic cells. Ki-67 is a nuclear protein expressed strictly in proliferating cells, with expression levels altering throughout the cell cycle. In healthy tissues, during interphase, Ki-67 can be exclusively detected in the nucleus, whereas in mitosis most of it is relocated to the chromosome surface. That Ki-67 is present during all active phases (G1, S, G2 and mitosis) of the cell cycle, but absent in resting cells (G0), makes it an excellent marker for determining the so-called growth fraction of a cell population (Scholzen & Gerdes Citation2000). Recently, membranous and cytoplasmic Ki-67 expression was described in studies of pathological conditions such as hyalinizing trabecular adenoma of the thyroid, sclerosing hemangioma of the lung, pleomorphic adenoma of the salivary gland, and in invasive ductal carcinomas of the breast (Hirokawa & Carney Citation2000; Hattori Citation2002; Tashiro et al. Citation2002; Faratian et al. Citation2009). While a relationship between membranous/cytoplasmic Ki-67 expression with other proteins has been noted (Faratian et al. Citation2009), the mechanism of subcellular Ki-67 relocalization remains unclear. To date, mutations in the Ki-67 gene have not been described in order to explain an abnormal protein product with altered localization properties (Faratian et al. Citation2009). However, Grzanka et al. (Citation2000) observed that cells from laryngeal squamous cell carcinoma with cytoplasmic and nuclear signal to Ki-67 were also positive for the Proliferating Cell Nuclear Antigen (PCNA), another proliferation marker; therefore, Ki-67 cytoplasmic relocalization does not alter its attribute as a marker of proliferation.
In the present study, we report for the first time that V exposure produced an altered localization of this nuclear protein in a manner akin to the above-mentioned pathologic state. It is interesting that these changes in localization were observed only in exposed male mice but not in control males or in either control or V-exposed females. The biologic significance of the relocation of Ki-67 in lymphocytes from V exposed mice remains to be discerned. For now, it is suggested that estrogen could have some protective effect against this particular V effect. Clearly, further studies are warranted to clarify this newly-found toxicity from inhaled V.
Conclusions
Vanadium inhalation increased the spleen lymphocyte proliferation in male mice in a length-of-exposure-dependent manner. Lymphocyte proliferation in the spleen could explain the splenomegaly and increase in white pulp size observed in earlier studies using this same exposure model. The importance of this induced lymphocyte proliferation induced by V inhalation, as well as noted changes in nuclear morphology and in the patterns of distribution of chromatin in splenic lymphocytes, remains to be investigated. While it is unlikely these changes could give rise to malignant disorders (i.e. leukemias/lymphomas), the potential contribution to induction of autoimmune diseases in V-exposed hosts reamins a possibility. Lastly, the effect of V on lymphocyte proliferation was seen to differ here by sex. Estrogen could be providing a protective effect against these immunotoxic effects of V. Only now, for V and for air pollutants in general, is it clear that there are likely sex-related differences in susceptiblity to these toxicants.
Acknowledgments
The authors wish to thank Veronica Rodriguez Mata and Raquel Guerrero Alquicira for their technical assistance and Francisco Pasos Nájera and Armando Zepeda Rodriguez for their photographic assistance.
Disclosure statement
The authors report no conflicts of interest. The authors alone are responsible for the content and writing of the paper.
References
- Amantea D, Russo R, Bagetta G, Corasaniti MT. 2005. From clinical evidence to molecular mechanisms underlying neuroprotection afforded by estrogens. Pharmacol Res. 52:119–132.
- Aragon MA, Ayala ME, Fortoul TI, Bizarro P, Altamirano-Lozano M. 2005. Vanadium-induced ultrastructural changes and apoptosis in male germ cells. Reprod Toxicol. 20:127–134.
- Badeau M, Adlercreutz H, Kaihovaara P, Tikkanen MJ. 2005. Estrogen A-ring structure and anti-oxidative effect on lipoproteins. J Steroid Biochem Mol Biol. 96:271–278.
- Barat C, Tremblay MJ. 2003. Treatment of human T-cells with bis-peroxovanadium phos-photyrosyl phosphatase inhibitors leads to activation of cyclooxygenase-2 gene. J Biol Chem. 278:6992–7000.
- Barceloux DG. 1999. Vanadium. J Toxicol Clin Toxicol. 37:265–278.
- Borras C, Sastre J, Garcia-Sala D, Lloret A, Pallardo FV, Vina J. 2003. Mitochondria from females exhibit higher anti-oxidant gene expression and lower oxidative damage than males. Free Radic Biol Med. 34:546–552.
- Calderon-Garciduenas L, Kulesza RJ, Doty RL, D'Angiulli A, Torres-Jardon R. 2015. Megacities air pollution problems: Mexico City Metropolitan Area critical issues on the central nervous system pediatric impact. Environ Res. 137:157–169.
- Cohen MD, Yang Z, Qu Q, Schlesinger RB, Zelikoff JT. 1996. Vanadium affects macrophage IFNγ-binding and -inducible responses. Toxicol Appl Pharmacol. 138:110–120.
- Cui W, Cui HM, Peng X, Zuo Z, Liu X, Wu B. 2011. Effect of vanadium on the subset and proliferation of peripheral blood T-cells, and serum IL-2 content in broilers. Biol Trace Elem Res. 141:192–199.
- Deng Y, Cui H, Peng X, Fang, J, Zuo, Z, Wang, K, Cui, W, Wu, B. 2012. Changes of IgA+ cells and cytokines in the cecal tonsil of broilers fed on diets supplemented with vanadium. Biol Trace Elem Res. 147:149–155.
- Di Gioacchino M, Sabbioni E, Di Giampaolo L, Schiavone C, Di Sciascio MB, Reale M, Nicola V, Qiao N, Paganelli R, Conti P, et al. 2002. In vitro effects of vanadate on human immune functions. Ann Clin Lab Sci. 32:148–154.
- Dockery DW, Pope CA 3rd. 1994. Acute respiratory effects of particulate air pollution. Annu Rev Public Health 15:107–132.
- Faratian D, Munro A, Twelves C, Bartlett JM. 2009. Membranous and cytoplasmic staining of Ki67 is associated with HER2 and ER status in invasive breast carcinoma. Histopathology 54:254–257.
- Fortoul TI, González-Villalva A, Pinon-Zarate G, Rodriguez-Lara V, Montano LF, Saldivar-Osorio L. 2009. Ultrastructural megakaryocyte modifications after vanadium inhalation in spleen and bone marrow. J Electron Microsc. (Tokyo) 58:375–380.
- Fortoul TI, Piñón-Zarate G, Diaz-Bech ME, González-Villalva A, Mussali-Galante P, Rodriguez-Lara V, Colin-Barenque L, Martinez-Pedraza M, Montaño LF. 2008. Spleen and bone marrow megakaryocytes as targets for inhaled vanadium. Histol Histopathol. 23:1321–1326.
- Fortoul TI, Quan-Torres A, Sánchez I, López IE, Bizarro P, Mendoza ML, Osorio LS, Espejel-Maya G, Avila-Casado Mdel C, Avila-Costa MR, et al. 2002. Vanadium in ambient air: Concentrations in lung tissue from autopsies of Mexico City residents in the 1960s and 1990s. Arch Environ Health 57:446–449.
- Fortoul TI, Rodriguez-Lara V, González-Villalva A, Rojas-Lemus M, Cano-Gutiérrez G, Ustarroz-Cano M, Colín-Barenque L, Bizarro-Nevaresa P, García-Pealeza I, Montaño LF, et al. 2014a. Inhalation of vanadium pentoxide and its toxic effects in a mouse model. Inorg Chim Acta 420:8–15.
- Fortoul TI, Rodriguez-Lara V, González-Villalva A, Rojas-Lemus M, Cano-Gutierrez G, Ustarroz-Cano M, Colin-Barenque L, Montaño LF, García-Pelez I, Bizarro-Nevares P, et al. 2011. Vanadium inhalation in a mouse model for the understanding of air-suspended particle systemic repercussion. J Biomed Biotechnol. 2011:11.
- Fortoul TI, Rojas-Lemus M, Rodriguez-Lara V, Gonzalez-Villalva A, Ustarroz-Cano M, Cano-Gutierrez G, Gonzalez-Rendon SE, Montaño LF, Altamirano-Lozano M, et al. 2014b. Overview of environmental and occupational vanadium exposure and associated health outcomes. J Immunotoxicol. 11:13–18.
- Gass K, Balachandran S, Chang HH, Russell AG, Strickland MJ. 2015. Ensemble-based source apportionment of fine particulate matter and emergency department visits for pediatric asthma. Am J Epidemiol. 181:504–512.
- Gonzalez-Villalva A, Pinon-Zarate G, Falcon-Rodriguez C, Lopez-Valdez N, Bizarro-Nevares P, Rojas-Lemus M, Rendon-Huerta E, Colin-Barenque L, Fortoul TI. 2014. Activation of Janus kinase/signal transducers and activators of transcription pathway involved in megakaryocyte proliferation induced by vanadium resembles some aspects of essential thrombocythemia. Toxicol Ind Health. [Epub ahead of print]. DOI: 10.1177/0748233713518600.
- González-Villalva A, Piñón-Zárate G, de la Peña Díaz A, Flores-García M, Bizarro-Nevares P, Rendón-Huerta EP, Colín-Barenque L, Fortoul TI. 2011. The effect of vanadium on platelet function. Environ Toxicol Pharmacol. 32:447–456.
- Gonzalez-Villalva A, Rodriguez-Lara V, Montano LF, Lima-Melo A, Ramirez G, Fortoul T. 2009. Blood changes generated after vanadium inhalation. Curr Topics Toxicol. 6:31–38.
- Grzanka A, Sujkowska R, Janiak A, Adamska M. 2000. Immunogold labelling of PCNA and Ki-67 antigen at the ultrastructural level in laryngeal squamous cell carcinoma and its correlation with lymph node metastasis and histological grade. Acta Histochem. 102:139–149.
- Hattori H. 2002. Sclerosing hemangioma of the lung is positive for MIB-1 in cell membrane and cytoplasmic staining pattern. Histopathology. 40:291–293.
- Hernandez H, Rodriguez, R. 2012. Geochemical evidence for the origin of vanadium in an urban environment. Environ Monit Assess. 184:5327–5342.
- Hirokawa M, Carney JA. 2000. Cell membrane and cytoplasmic staining for MIB-1 in hyalinizing trabecular adenoma of the thyroid gland. Am J Surg Pathol. 24:575–578.
- Hope B. 2008. A dynamic model for the global cycling of anthropogenic vanadium. Global Biogeochem Cycles. 22:16.
- Huang C, Ding M, Li J, Leonard SS, Rojanasakul Y, Castranova V, Vallyathan V, Ju G, Shi X. 2001. Vanadium-induced nuclear factor of activated T-cells activation through hydrogen peroxide. J Biol Chem. 276:22397–22403.
- IARC (International Agency for Research on Cancer). 2006. Vanadium pentoxide. IARC Monographs on the Evaluation of Carcinogenic Risks to Humans; 86:227–292.
- Jahn HJ, Schneider A, Breitner S, Eissner R, Wendisch M, Kramer A. 2011. Particulate matter pollution in megacities of the Pearl River Delta, China: A systematic literature review and health risk assessment. Intl J Hyg Environ Health. 214:281–295.
- Kim KH, Kabir E, Kabir S. 2015. A review on human health impact of airborne particulate matter. Environ Intl. 74:136–143.
- Klein-Patel ME, Diamond G, Boniotto M, Saad S, Ryan LK. 2006. Inhibition of β-defensin gene expression in airway epithelial cells by low doses of residual oil fly ash is mediated by vanadium. Toxicol Sci. 92:115–125.
- Krejsa CM, Nadler SG, Esselstyn JM, Kavanagh TJ, Ledbetter JA, Schieven GL. 1997. Role of oxidative stress in the action of vanadium phosphotyrosine phosphatase inhibitors. Redox independent activation of NF-κB. J Biol Chem. 272:11541–11549.
- Li Z, Stonehuerner J, Devlin RB, Huang YC. 2005. Discrimination of vanadium from zinc using gene profiling in human bronchial epithelial cells. Environ Health Perspect. 113:1747–1754.
- Mancini A, Raimondo S, Persano M, Di Segni C, Cammarano M, Gadotti G, Silvestrini A, Pontecorvi A, Meucci E. 2013. Estrogens as anti-oxidant modulators in human fertility. Intl J Endocrinol. 2013:607939.
- Miyaji M, Kortum RL, Surana R, Li W, Woolard KD, Simpson RM, Samelson LE, Sommers CL. 2009. Genetic evidence for the role of ERK activation in a lymphoproliferative disease of mice. Proc Natl Acad Sci USA. 106:14502–14507.
- Morgan MJ, Liu ZG. 2011. Crosstalk of reactive oxygen species and NF-κB signaling. Cell Res. 21:103–115.
- Morinville A, Maysinger D, Shaver A. 1998. From Vanadis to Atropos: Vanadium compounds as pharmacological tools in cell death signaling. Trends Pharmacol Sci. 19:452–460.
- Mukherjee B, Patra B, Mahapatra S, Banerjee P, Tiwari A, Chatterjee M. 2004. Vanadium: An element of atypical biological significance. Toxicol Lett. 150:135–143.
- Muñoz E, Gomez F, Paz JL, Casado I, Silva JM, Alonso MJ. 2003. Ki-67 immunolabeling in pre-malignant lesions and carcinoma of the prostate. Histological correlation and prognostic evaluation. Eur J Histochem. 47:123–128.
- Nandakumar DN, Koner BC, Vinayagamoorthi R, Nanda N, Negi VS, Goswami K, Bobby Z, Hamide A. 2008. Activation of NF-κB in lymphocytes and increase in serum immunoglobulin in hyperthyroidism: Possible role of oxidative stress. Immunobiology. 213:409–415.
- Ngo ST, Steyn FJ, McCombe PA. 2014. Gender differences in autoimmune disease. Front Neuroendocrinol. 35:347–369.
- OSHA (Occupational Safety and Health Administration). 2012. Chemical Sampling Information. Vanadium fume (as V2O5). [Internet]. Washington DC. [cited 2012 June 9] Online from: https://www.osha.gov/dts/chemicalsampling/data/CH_275000.html.
- Pandey SK, Theberge JF, Bernier M, Srivastava AK. 1999. Phosphatidylinositol 3-kinase requirement in activation of the ras/C-raf-1/MEK/ERK and p70(s6k) signaling cascade by the insulinomimetic agent vanadyl sulfate. Biochemistry. 38:14667–14675.
- Pinon-Zarate G, Rodriguez-Lara V, Rojas-Lemus M, Martinez-Pedraza M, Gonzalez-Villalva A, Mussali-Galante P, Fortoul TI, Barquet A, Masso F, Montano LF. 2008. Vanadium pentoxide inhalation provokes germinal center hyperplasia and suppressed humoral immune responses. J Immunotoxicol. 5:115–122.
- Qureshi MA, Hill CH, Heggen CL. 1999. Vanadium stimulates immunological responses of chicks. Vet Immunol Immunopathol. 68:61–71.
- Rodriguez-Lara V. 2008. Alteraciones morfológicas del núcleo de linfocitos de ratones expuestos a la inhalación aguda, subagúda y crónica de V2O5: Participación de las láminas nucleares. [Internet]. Master, Universidad Nacional Autónoma de México. UNAM. Thesis. [cited 2012 Jan 06] Available from: http://132.248.9.195/ptd2008/septiembre/0632177/Index.html.
- Rodriguez-Lara V, Aleman Muench G, Soldevila G, García Zepeda E, Fortoul T. 2013a. Ultrastructural nuclear changes in mice spleen lymphocytes after vanadium inhalation. Clin Exp Pharmacol. S4:003.
- Rodriguez-Lara V, Morales-Rivero A, Rivera-Cambas AM, Fortoul TI. 2013b. Vanadium inhalation induces actin changes in mice testicular cells. Toxicol Ind Health. [Epub ahead of print]. DOI: 10.1177/0748233713501364.
- Scotland RS, Stables MJ, Madalli S, Watson P, Gilroy DW. 2011. Sex differences in resident immune cell phenotype underline more efficient acute inflammatory responses in female mice. Blood. 118:5918–5927.
- Scholzen T, Gerdes J. 2000. The Ki-67 protein: From the known and the unknown. J Cell Physiol. 182:311–322.
- Straub RH. 2007. The complex role of estrogens in inflammation. Endocrinol Rev. 28:521–574.
- Tashiro T, Hirokawa M, Harada H, Yokoyama S, Sano T. 2002. Cell membrane expression of MIB-1 in salivary gland pleomorphic adenoma. Histopathology. 41:559–561.
- Ustarroz-Cano M, Garcia-Pelaez I, Pinon-Zarate G, Herrera-Enriquez M, Soldevila G, Fortoul TI. 2012. CD11c decrease in mouse thymic dendritic cells after vanadium inhalation. J Immunotoxicol. 9:374–380.
- Vina J, Gambini J, Lopez-Grueso R, Abdelaziz KM, Jove M, Borras C. 2011. Females live longer than males: Role of oxidative stress. Curr Pharm Des. 17:3959–3965.
- Vrachnis N, Zygouris D, Iliodromiti Z, Daniilidis A, Valsamakis G, Kalantaridou S. 2014. Probing the impact of sex steroids and menopause-related sex steroid deprivation on modulation of immune senescence. Maturitas. 78:174–178.
- Walters DM, White KM, Patel U, Davis MJ, Veluci-Marlow RM, Solomon Raju BS, Bonner JC, Martin JR, Gladwell W, Kleeberger SR. 2014. Genetic susceptibility to interstitial pulmonary fibrosis in mice induced by vanadium pentoxide (V2O5). FASEB J. 28:1098–1112.
- Wang K, Cui H, Peng X, Zuo Z, Fang J, Deng J, Deng Y, Cui W, Wu B. 2012. Effect of dietary vanadium on the ileac T-cells and contents of cytokines in broilers. Biol Trace Elem Res. 147:113–119.
- Wang YZ, Bonner JC. 2000. Mechanism of extracellular signal-regulated kinase (ERK)-1 and ERK-2 activation by vanadium pentoxide in rat pulmonary myofibroblasts. Am J Respir Cell Mol Biol. 22:590–596.
- WHO (World Health Organization). 2014. News release: 7 Million premature deaths annually linked to air pollution. Geneva: WHO Publications.
- WHO (World Health Organization). Regional Office for Europe. 2000. Vanadium. WHO Regional Publications, European Series No. 91. Copenhagen: WHO.
- Ye J, Ding M, Zhang X, Rojanasakul Y, Nedospasov S, Vallyathan V, Castranova V, Shi X. 1999. Induction of TNFα in macrophages by vanadate is dependent on activation of transcription factor NF-κB and free radical reactions. Mol Cell Biochem. 198:193–200.
- Zaporowska H, Wasilewski W. 1992. Hematological results of vanadium intoxication in Wistar rats. Comp Biochem Physiol. C 101:57–61.
- Zink D, Fischer AH, Nickerson JA. 2004. Nuclear structure in cancer cells. Nat Rev Cancer 4:677–687.
- Zwolak I. 2014. Vanadium carcinogenic, immunotoxic and neurotoxic effects: A review of in vitro studies. Toxicol Mech Meth. 24:1–12.