Abstract
Gasoline exhaust particles (GEP) and diesel exhaust particles (DEP) are considered to be some of the most important air pollutants. Among the many constituents in these pollutant particles, 4-pentylphenol (PP) and 3-methyl-4-nitrophenol (PNMC) are considered important phenolics in GEP and DEP, respectively. The aim of this study was to investigate the effect of in vitro exposure to commercially-supplied PP and PNMC on populations of, and production of interleukin (IL)-2, IL-4 and granzyme-B by, mouse splenic lymphocytes. After in vitro exposure to PP or PNMC for 48 h, splenocyte viability was measured, cell phenotypes, e.g. B-cell (CD19), T-cells (CD3), T-cell subsets (CD4 and CD8), were quantified by flow cytometry and production of IL-2, IL-4 and granzyme-B was assessed via ELISA. The oxidative toxicity of PP and PNMC toward the splenocytes was also evaluated using measures of hydroxyl radical and malondiadehyde production and changes in glutathione peroxidase and superoxide dismutase activities. Results showed that in vitro exposure to PP and PNMC inhibited splenic cell parameters in a dose-related manner. Exposure to PP and PNMC decreased splenic T-lymphocyte populations and splenocyte production of cytokines and granzyme B, as well as induced oxidative stress in the splenocytes. The results also showed that the percentages of CD3+ T-cells overall and of CD4+ and CD8+ T-cells therein, among exposed splenocytes, were reduced; neither compound appeared to affect levels of CD19+ B-cells. Overall, the suppressive effects of PP were stronger than PNMC. The data here provide support for the proposal that PP-/PNMC-induced toxicity in splenocytes may be due at least in part to oxidative damage and that PP and PNMC – as components of GEP and DEP – might significantly impact on splenic T-cell formation/release of cytokines/granzymes in situ.
Introduction
Air pollution has become a major worldwide health issue in part due to increasing vehicle emissions containing gasoline exhaust particles (GEP) and/or diesel exhaust particles (DEP) (Nakamura et al. Citation2012; Alexis & Carlsten Citation2014; Claxton Citation2015). Accumulating evidence has indicated that GEP exposure increases the risk of pulmonary diseases (Sureshkumar et al. Citation2005) and induces reproductive toxicity (Guo et al. Citation2004). DEP contain thousands of compounds that can have hazardous effects on human health, causing a variety of diseases, including lung cancer.
4-pentylphenol (PP) derived from GEP matter (Murahashi et al. Citation2003) has been shown to significantly affect the endocrine and reproductive systems as well as the redox status of Atlantic cod (Satoh et al. Citation2005; Meier et al. Citation2007). In vivo exposure to polycyclic aromatic hydrocarbons, an important class of GEP, caused inhibitory effects on T-cell proliferation (Karakaya et al. Citation2004). However, there have been no reports on immune effects from PP itself so far. Many studies have also implicated DEP in the disruption of the endocrine, reproductive and immune systems (Singh et al. Citation2005; Hemmingsen et al. Citation2009; Ema et al. Citation2013; Müller et al. Citation2013). In vitro DEP exposure causes T-regulatory cell dysfunction and this impairment is believed to lead to uncontrolled inflammation in exposed hosts (Chiang et al. Citation2009). DEP also has been shown to suppresses T-helper Type 1 immune responses in vitro (Sasaki et al. Citation2009) and suppress cytokine release from alveolar macrophages (Amakawa et al. Citation2003). Benzo[a]pyrene (BaP, a major aromatic hydrocarbon in DEP) causes reductions in both Thy 1.2+ T-cells and CD45R+ B-cells in the spleens of treated mice (Holladay & Smith Citation1995).
Four nitrophenol ingredients, e.g. 4-nitrophenol, 2-methyl-4-nitrophenol, 4-nitro-3-phenylphenol and 3-methyl-4-nitrophenol (PMNC) have been detected in DEP (Mori et al. Citation2003). PNMC has been shown to have estrogenic activity in vitro and in vivo (Furuta et al. Citation2004, Citation2005), induce reproductive toxicity in male rats (Li et al. Citation2006, Citation2007; Yue et al. Citation2011) and cause immunotoxicity. Specifically, PNMC appeared to affect splenic T-cells in rats in vivo (Li et al. Citation2010). However, the precise mechanism of this toxicity remains to be elucidated.
Numerous reports have shown that exposure to DEP may affect immunological function. Recent studies have shown that DEP exposure may help to generate reactive oxygen species (ROS) (Chao et al. Citation2012; Vattanasit et al. Citation2014) and decrease specific activities of superoxide dismutase (SOD), glutathione peroxidase (GSHPX) and glutathione reductase (GSHRX) in cells. DEP is also known to cause increases in lipid peroxidation, oxidative stress and DNA damage in human A549 lung epithelial cells and murine RAW 264.7 macrophages (Durga et al. Citation2014). Because it is suspected that PP and PNMC induce toxicity potentially via oxidative damage (Aam & Fonnum Citation2007), this study was undertaken to investigate the effect of exposure to PP and PNMC on splenic lymphocytes and to evaluate the relationship between any PP- and PNMC-induced toxicities and oxidative stress.
Materials and methods
Materials
PP was purchased from Sigma (St. Louis, MO) and PNMC from TCI Chemicals (Tokyo, Japan). RPMI 1640 medium and phosphate-buffered saline (PBS, pH 7.4) was obtained from Mediatech (Manassas, VA). Pharmingen Stain Buffer (BSA) was obtained from BD (Becton Dickinson, San Diego, CA). 3-(4,5-dimethylthiazol-2-yl)-2,5-diphenyl tetrazolium bromide (MTT) was purchased from Sigma. ELISA kits for IL-2, IL-4 and Granzyme B were purchased from Cusabio Biotech (Wuhan, China). Assay kits of Hydroxyl Free Radical (•OH), superoxide dismutase (SOD), glutathione peroxidase (GSHPX) and malondialdehyde (MDA) were bought from the Nanjing Jiancheng Bioengineering Institute (Nanjing, PR China).
The following antibodies purchased from Biogen (San Diego, CA) were used in the phenotypic analysis studies: fluorescein isothiocyanate (FITC)-labeled anti-mouse CD3 (IgG2b) to stain T-cells, FITC-anti-mouse CD4 (IgG2b) and FITC-anti-mouse CD8 (IgG2b) to stain T-cell subsets and FITC-anti-mouse CD19 (IgG2a) to stain B-cells. FITC-labeled rat IgG2a and IgG2b were used as negative isotype controls.
Experimental animals
Specific-pathogen-free Kunming mice (male, 8-weeks-of-age) were purchased from the Military Academy of Medical Sciences Laboratory Animal Center (Beijing, PR China) to serve as the source of cells for use in all assays herein. The mice were housed in a pathogen-free facility maintained at a temperature of 23–25 °C and a relative humidity at 57–60% with a 12-h light–dark cycle. All mice had ad libitum access to standard sterilized rodent chow and filtered water. All procedures here were carried out in accordance with the Policy on the Care and Use of Animals established by the Ethical Committee of the Beijing Forestry University and approved by the Department of Agriculture of Hebei Province, PR China (JNZF11/2007).
Preparation of splenocytes
Based on the protocols of Benencia et al. (Citation2000), naïve mice were euthanized by cervical dislocation and their spleens were removed. Single cell suspensions were prepared by mincing and tapping spleen fragments on a stainless 200-mesh held in RPMI 1640 medium. Thereafter, erythrocytes present were lysed by incubating the cells in ammonium chloride (0.8%, w/v) solution on ice for 2 min. After centrifugation (380 × g, 5 min), the pelleted cells were washed three times with RPMI-1640 and finally re-suspended in RPMI-1640 supplemented with 10% fetal bovine serum, 2 mM L-glutamine, 100 U penicillin/ml and 100 μg streptomycin/ml (all products from Mediatech). Cell number and viability were determined using a hemocytometer and trypan blue dye. Cell viability always exceeded 95%.
Cell viability assay
Measurement of cell survival was assayed as previously described (Sun et al. Citation2005). In brief, splenocytes were seeded into 96-well flat-bottom microtiter plates at 5 × 106 cell/ml in 100 μl complete medium. After 4 h, 100 μl PP (10−6∼10−4 M final concentration in well) or PNMC (10−6∼10−4 M final concentration in well) or RPMI 1640 medium was added to designated wells. The plates were then incubated at 37 °C in a humidified atmosphere with 5% CO2. After 48 h, 20 μl MTT solution (5 mg/ml) was added to each well and the plates incubated for a further 4 h. After carefully removing the culture supernatant, 200 μl DMSO was added to each well. After 20 min, the absorbance in each well was measured at 570 nm in an ELISA plate reader (BioRad, Hercules, CA). Viability was determined as relative absorbance to the value for the control cells.
Cell staining/flow cytometry for phenotypic analysis
Cell staining for phenotypic analysis was carried out as described previously (Li et al. Citation2000, Citation2010). In short, the treated spleen cells were harvested, then washed with PBS and re-counted. Aliquots of cells for staining were diluted to 2.5 × 107 cells/ml in PBS, re-centrifuged and then re-suspended in 50 μl Ab Block (BD Pharmingen Stain Buffer [BSA]). After blocking for 5 min at 4 °C, the cells were then transferred to test tubes, to which a given specific FITC-labeled antibody at 1 μg/ml (optimal dose as determined by preliminary experiments) was then added. All samples were then incubated for 30 min at 4 °C in the dark. After staining, the cells were washed three times with PBS and centrifuged. After the final washing, the cells were placed into FACS tubes (in PBS) in a total volume of 1 ml. Flow cytometric analysis was then performed in a BD FACS Calibur flow cytometer (Becton Dickinson, San Diego, CA) equipped with a 488 nm argon laser and detectors for measures of forward scatter (FSC), 90 °C light scatter (side-scatter, SSC) and FL1 (530 nm), FL2 (585 nm) and FL3 (650 nm) fluorescence emission in the green, red/orange and long-red parts of the spectra, respectively. Splenocytes stained with FITC-labeled antibodies were detected on FL1. A minimum of 10 000 events were acquired and stored for each analysis. Splenocytes were identified by their characteristic appearance on a dot-plot of FSC vs SSC and electronically gated to exclude any residual platelets, red cells or dead cell debris. The gate was the same for both exposed and control splenocytes. The results were indicated as the percentage of positive cells within a gate. All analyses were performed with FloJo software (Emerald Biotech, Hangzhou, PR China).
Measurement of cytokine/granzyme production and determination of •OH, SOD, GSH-Px and MDA levels
For assessment of the formation/release of IL-2, IL-4, granzyme-B, splenocytes were cultured for 48 h at a density of 5 × 106 cells/ml in 96-well plates in the presence/absence of the test agents. Culture supernatant was then collected and IL-2, IL-4 and granzyme-B levels quantified using commercial ELISA kits. The lower detection limit of the kits was 3.9 pg IL-2/ml, 0.4 pg IL-4/ml and 3.1 pg Granzyme B/ml. Culture supernatants were also assessed for SOD, GSH-Px, •OH and MDA using commercial assay kits for each. The lower detection limit of the kits was 0.5 U SOD/ml, 0.5 U GSH-Px/ml, 0.04 U •OH/ml and 0.01 mmol MDA/ml.
Statistical analysis
All data were expressed as means ± SD. The significance of any differences due to treatment was evaluated using a Student’s t-test (as part of SPSS software package [IBM, Armonk, NY]). Significance was accepted at a p value < 0.05.
Results
Effects of PP and PNMC on splenocyte viability
To determine the effect of PP and PNMC on splenocyte, cell viability was determined using an MTT assay. As shown in , PP and PNMC both inhibited cell growth. The suppressing effects of PP and PNMC appeared to occur in a concentration-related fashion. Both agents significantly inhibited splenocyte survival compared to the control (untreated group) at 10−4 M; viability after treatment with PP and PNMC (10−4 M) decreased to 66.3% and 88.7%, respectively, of control. As such, a concentration of 10−4 M of PP and of PNMC was chosen for use in all subsequent experiments herein.
Effects of PP and PNMC on splenic T- and B-cell phenotypes
To investigate the effects of PP and PNMC on splenic T- and B-cell sub-populations, splenocytes stained with FITC-labeled antibodies were evaluated by flow cytometry after the 48-h treatments. The results showed that the percentages of CD3+ T-cells in the exposed splenocytes were smaller than in the control splenocyte mixture (). The results also showed that the percentages of CD4+ and CD8+ T-cells among the exposed splenocytes were lower than in the controls (). However, neither agent significantly impacted on CD4+ T-cell (T-helper [TH] cell) percentages, but PP did have a significant effect on CD8+ T-cell percentages. Neither compound appeared to affect levels of CD19+ B-cells ().
Figure 2. Percentages of various lymphocyte cell types as determined using flow cytometric analysis. (A) CD3+ T-cells, (B) CD4+ T-cells, (C) CD8+ T-cells and (D) B-cells in cells exposed to medium only (control), PP (10−4 M) or PNMC (10−4 M) for 48 h. Results shown are means ± SD of three separate experiments. *p < 0.05 vs untreated control.
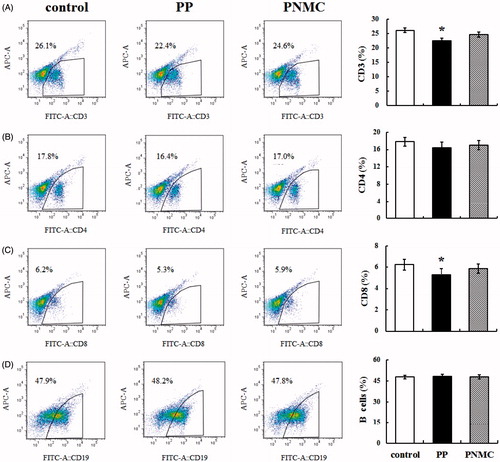
Effects of PP and PNMC on splenic T-cell cytokine/granzyme production
To assess effects of PP and PNMC on splenocyte IL-2, IL-4 and granzyme B production, after 48 h in the presence/absence of PP and PNMC, the quantities of each protein in the cultures were measured using an ELISA. CD4+ TH cells can be subdivided into TH1 and TH2 phenotypes. Here, it was expected that IL-2, IL-4 and granzyme B were to have been secreted primarily by resident CD4+ TH1 cells, CD4+ TH2 cells and CD8+ T-cells, respectively.
It is important to note for the Readers there is also a possibility of contributions to the two interleukin levels by resident TH0 cells that might have been (or activated to become) present in the cell mixtures during the culture period. TH0 cells produce IL-4, IL-2 and IFNγ (and likely also IL-5) when naive CD4 cells are exposed to a mixture of exogenous/endogenous IL-4 and IFNγ over a few days. As the magnitude of TH1-like/TH2-like cytokines produced by these cells is regulated by IL-4 and IFNγ, this means TH0 cells could evolve in the cultures that differ in abilities to secrete varying levels of these/any individual cytokine. While the current study did not analyze TH0 cell levels in the test populations, it is important to note that it is accepted that TH0 cells are not rarely generated (and, thus, are transitory in nature) in the spleen (Miner & Croft Citation1998). Therefore, the possibility remains that the TH0 phenotype could represent another fully differentiated sub-population of cells that could have been impacted on by the PP or PMNC.
Regardless of specific cell (subtype) source, the results shown in indicate that incubation with PP or PNMC caused significant decreases in IL-2, IL-4 and granzyme B secretion by the splenocytes. IL-2 formation by the cells due to PP was reduced to 58.0% (), IL-4 to 52.3% () and granzyme B to 27.2% () of control levels. IL-2 formation by the cells due to PNMC was reduced (relative to control) to 36.4% (), IL-4 to 77.4% () and granzyme B to 51.7% () of control levels.
Figure 3. Effects of PP and PNMC on select splenocyte cytokine/granzyme production. Naïve host splenocytes were cultured for 48 h in the presence of medium only or medium with PP or PNMC (10−4 M). Levels of (A) IL-2, (B) IL-4 and (C) granzyme B released into culture media were then measured by ELISA. Results shown are means ± SD of three separate experiments. *p < 0.05 or **p < 0.01 vs untreated control.

Oxidative effect of PP and PNMC on splenocytes
To investigate the potential oxidative effect of PP or PNMC on splenocytes, levels of SOD, GSH-Px, OH and MDA associated with the cultured cells were evaluated. As shown in , •OH content and MDA levels were significantly enhanced by PP; PNMC only caused a significant increase in •OH content relative to in control cultures. •OH content was increased to 923.0 U/ml (p < 0.01) and 852.6 U/ml (p < 0.05), respectively, by PP and PNMC (), while MDA content increased to 0.63 mmol/ml (p < 0.01) and 0.60 mmol/ml (p < 0.05), respectively (). In contrast, there were marked decreases in SOD and GSH-Px activity in the treated cells; SOD activity was reduced by PP and PNMC, respectively, to 76.8% (p < 0.01) and 84.8% of control (p < 0.05) (). In these same cells, GSHPX activity was reduced by PP and PNMC, respectively, to 54.6% (p < 0.01) and 72.7% (p < 0.05) of control ().
Figure 4. Hydroxyl free radical, anti-oxidant enzymes activities and MDA content in splenocytes exposed to PP or PNMC (10−4 M) for 48 h. Changes in (A) hydroxyl free radical (•OH) activity, (B) superoxide dismutase (SOD) activity, (C) glutathione peroxidase (GSH-Px) activity and (D) malonaldehyde (MDA) content in the cells were measured using specific assay kits. Results shown are means ± SD of three separate experiments. *p < 0.05 or **p < 0.01 vs untreated control.
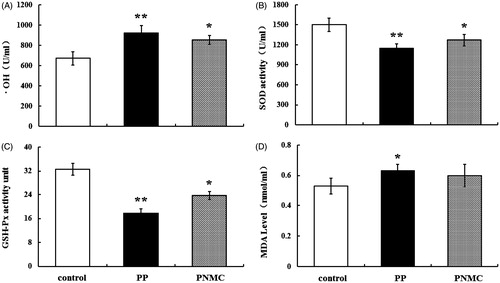
Discussion
In this study, immunotoxicities of PP and PNMC were investigated using a mouse splenocyte culture model. Cell viability assays illustrated that PP and PNMC inhibited mouse splenocyte viability and that the cytotoxicity of PP was more significant than that of PNMC. Analysis of cell phenotypes showed that PP and PNMC affected levels of splenic CD3+, CD4+ and CD8+ T-cells. In addition, assays here indicated that production of IL-2, IL-4 and granzyme B were significantly decreased after treatment with PP and PNMC. Furthermore, changes in levels of •OH, SOD, GSHPX and MDA suggested that cellular oxidative balance was disrupted by the PP or PNMC treatments. Taken together, these findings could suggest that PP and PNMC might impact on splenic T-lymphocyte populations and cytokine production in situ, that the toxicity of PP was more significant than that of PNMC and that the immunotoxicity of PP and PNMC was closely related to oxidative damage to the splenic cells. In regard to this latter point, this type of effect could also implicate necrotic cell cytotoxicity or apoptosis as contributing factors to the observed effects on the treated cells. While the present study did not analyze for the incidence of apoptosis/necrosis among the various cell populations, this is an area under ongoing investigation in our current studies to determine if this is an important mechanism of toxicity for these phenolics.
While PP has been reported to be estrogenic (Satoh et al. Citation2005) and cause reproductive disorders in fish (Meier et al. Citation2011), there have been no reports about the immunotoxicity of PP so far. Here, it was seen that PP reduced splenocyte viability in a concentration-related manner. Previously, 4-tert-octylphenol (OP, a molecule similar to PP) was shown to induce vacuolization and edema in splenic tissues of male and female Wistar rats (Barlas & Aydoğan Citation2009) and of the rabbitfish (Siganus oramin; Du et al. Citation2008). Similarly, it was seen here that PNMC reduced splenocyte viability. Previous studies have repeatedly shown that DEP induces significant decreases in splenic and thymic indices, atrophy of the thymus and suppression of various immune functions in mice and rats (Moon et al. Citation1986; Nakashima et al. Citation2002). In vivo studies revealed that PNMC exposure also significantly decreased the weight of the spleen and body weight (Li et al. Citation2010). In addition, apoptotic lymphocytes in the spleen were noted in PNMC-exposed rats (Li et al. Citation2010). Although it is difficult to interpret directly and make any direct link to those in vivo studies based on our results with PP or PNMC at 10−4 M, those past results – when taken together with the present findings – nevertheless indicate that that PP and PNMC can exert negative effects on splenic cell populations.
The flow cytometric analysis here revealed that PP and PNMC impacted on the presence of splenic T-cells but had no apparent effect on B-cells. A similar observation was reported in the previous study in Wistar rats that showed that subchronic oral exposure to PNMC at 10 mg/kg BW for 9 weeks induced a significant decrease in the percentages of splenic CD3+ and CD8+ T-cells in rats (Li et al. Citation2010). However, PNMC-exposure did not affect splenic CD4 T-cells, B-cells, NK cells, macrophages and granulocytes; this indicated that PNMC might have selectively affected splenic CD3+ and CD8+ T-cells (Li et al. Citation2010). In addition, in the mouse lung, DEP is known to cause increases in the numbers of CD3+ and CD8+ T-cells in the parenchyma, while CD4+ T-cell and CD20+ B-cell numbers were unaltered (Yoshizaki et al. Citation2015). In the mouse, host exposure to DEP resulted in a severe thymic involution accompanied by a reduction in number of thymocytes, especially in cortical CD4+CD8+ double-positive and double-negative subsets (Tomita et al. Citation2006). Similarly, DEP have been shown activate naive splenocytes by significantly affecting the surface expression of molecules related to T-cells in atopy-prone animals (Inoue et al. Citation2011). Therefore, the present results were in general agreement with many previous observations about how DEP may selectively affect T-cells.
Cytokines play substantial regulatory roles in immune cell development, differentiation and function (Yoshimura et al. Citation2007) and DEP/its constituents have been shown to impact on their formation/release by immune/non-immune cells. In a previous study, DEP exposure led to complex and diverse effects on cytokine production by mouse alveolar macrophages (AM) and that long in vitro exposures (> 8 h) led to suppressed cytokine production by AM (Saito et al. Citation2002). Similarly, benzo[a]pyrene (BaP) caused a stimulatory effect on cytokine release by airway epithelial cells (Ohtoshi et al. Citation1998). In rat spleens, DEP injection of the host not only altered the function of CD4+ cells but also that of CD8+ T-cell subsets with regard to synthesis of cytokines (Fujimaki et al. Citation2001). Mice injected with DEP also manifest decreased IL-2 and IL-4 production (Finkelman et al. Citation2004). DEP was also shown to enhance the manifestations of allergic asthma by causing increased local expression of IL-5 and also by modulating expression of IL-2, IL-4 and granulocyte macrophage-colony stimulating factor (GM-CSF) (Takano et al. Citation1997). DEP was shown earlier to significantly inhibit secretion of cytokines by NK cells (Müller et al. Citation2013). As IL-2 is mainly produced by activated CD4+ TH1 cells, IL-4 by CD4+ TH2 cells and granzyme B by CD8+ CTL cells, the present results showing that use of PP and PNMC significantly reduced measurable levels of IL-2, IL-4 and granzyme B in the cultures indicated that PP and PNMC might also significantly impact on splenic T-cell formation/release of cytokines/granzymes in situ.
The cause for the GEP and DEP induced immunotoxicity seen here is not clear. However, it is well known that ROS plays a crucial role in immune damage progression (Bakunina et al. Citation2015). Metabolic activation of GEP and DEP may lead to generation of ROS that are, in turn, responsible for their immunotoxicity (Yin et al. Citation2004; Ohyama et al. Citation2007). To see in fact if ROS/oxidative damage could play a role in the GEP- and DEP-induced effects here, the status of the cellular enzymatic defense system against free radical attacks was assessed through measures of their associated SOD and GSHPX activities. It was seen here that SOD and GSHPX activities were significantly decreased in the PP- and PNMC-treated splenocytes. It was unsurprising then that levels of •OH, one of the most toxic and harmful ROS to an organism, were concurrently increased. Similarly, levels of malondialdehyde (MDA) – an end-product of lipid peroxidation on cells also were increased due to the treatments. These results, when taken together, clearly indicated that PP and PNMC disrupted the redox status balance in the host splenocytes/immune cells. This data is consistent with previous studies that established the effect of PNMC on oxidative stress parameters in several models (Mi et al. Citation2009; Bu et al. Citation2012). The data also provide support for the proposal that PP-/PNMC-induced toxicity in splenocytes may be due at least in part to oxidative damage.
Conclusion
The present study showed that exposure to PP and PNMC affected splenic lymphocyte viability/spontaneous populations and cytokine/granzyme production in vitro and that PP had a stronger effect than PNMC. In-depth animal studies should be carried out to further clarify these effects and discern mechanisms by which these constituents of vehicle emissions might impact on immune function in vivo.
Acknowledgments
This study was supported by the Beijing Natural Science Foundation (8142029).
Disclosure statement
The authors report no conflict of interests. The authors alone are responsible for the content and writing of the paper.
References
- Aam BB, Fonnum F. 2007. ROS scavenging effects of organic extract of diesel exhaust particles on human neutrophil granulocytes and rat alveolar macrophages. Toxicology. 230:207–218.
- Alexis NE, Carlsten C. 2014. Interplay of air pollution and asthma immunopathogenesis: A focused review of diesel exhaust and ozone. Intl Immunopharmacol. 23:347–355.
- Amakawa K, Terashima T, Matsuzaki T, Matsumaru A, Sagai M, Yamaguchi K. 2003. Suppressive effects of diesel exhaust particles on cytokine release from human and murine alveolar macrophages. Exp Lung. Res. 29:149–164.
- Bakunina N, Pariante CM, Zunszain PA. 2015. Immune mechanisms linked to depression via oxidative stress and neuroprogression. Immunology. 144:365–373.
- Barlas N, Aydoğan M. 2009. Histopathologic effects of maternal 4-tert-octylphenol exposure on liver, kidney and spleen of rats at adulthood. Arch Toxicol. 83:341–349.
- Benencia F, Courrèges MC, Coulombié FC. 2000. In vivo and in vitro immunomodulatory activities of Trichilia glabra aqueous leaf extracts. J Ethnopharmacol. 69:199–205.
- Bu TL, Jia YD, Lin JX, Mi YL, Zhang CQ. 2012. Alleviative effect of quercetin on germ cells intoxicated by 3-methyl-4-nitrophenol from diesel exhaust particles. J Zhejiang Univ Sci. 13:318–326.
- Chao MW, Po IP, Laumbach RJ, Koslosky J, Cooper K, Gordon MK. 2012. DEP induction of ROS in capillary-like endothelial tubes leads to VEGF-A expression. Toxicology. 297:34–46.
- Chiang D, Sanjanwala B, Nadeau KC. 2009. Diesel exhaust particles impair regulatory T- cell function. American Thoracic Society, International Conference, San Diego, CA; Abstract 4295.
- Claxton LD. 2015. The history, genotoxicity, and carcinogenicity of carbon-based fuels and their emissions. Part 3: Diesel and gasoline. Mutation Research/Reviews in Diesel and gasoline. Mutat Res Rev. 763:30–85.
- Du YB, Li YY, Zhen YJ, Hu CB, Liu WH, Chen WZ, Sun ZW. 2008. Toxic effects in Siganus oramin by dietary exposure to 4-tert-octylphenol. Bull. Environ. Contam Toxicol. 80:534–538.
- Durga M, Nathiya S, Rajasekar A, Devasena T. 2014. Effects of ultrafine petrol exhaust particles on cytotoxicity, oxidative stress generation, DNA damage and inflammation in human A549 lung cells and murine RAW 264.7 macrophages. Environ Toxicol Pharmacol. 38:518–530.
- Ema M, Naya M, Horimoto M, Kato H. 2013. Developmental toxicity of diesel exhaust: A review of studies in experimental animals. Reprod Toxicol. 42:1–17.
- Finkelman FD, Yang M, Orekhova T, Clyne E, Bernstein J, Whitekus M, Diaz-Sanchez D, Morris SC. 2004. Diesel exhaust particles suppress in vivo IFNγ production by inhibiting cytokine effects on NK and NKT cells. J Immunol. 172:3808–3813.
- Fujimaki H, Ui N, Ushio H, Nohara K, Endo T. 2001. Roles of CD4+ and CD8+ T-cells in adjuvant activity of diesel exhaust particles in mice. Intl Arch Allergy Immunol. 124:485–496.
- Furuta C, Li CM, Taneda S, Suzuki AK, Kamata K, Watanabe G, Taya K. 2005. Immunohistological study for estrogenic activities of nitrophenols in diesel exhaust particles. Endocrine. 27:33–36.
- Furuta C, Suzuki AK, Taneda S, Kamata K, Hayashi H, Mori Y, Li CM, Watanabe G, Taya K. 2004. Estrogenic activities of nitrophenols in diesel exhaust particles. Biol Reprod. 70:1527–1533.
- Guo J, Kauppinen T, Kyyrönen P, Heikkilä P, Lindbohm ML, Pukkala E. 2004. Risk of esophageal, ovarian, testicular, kidney and bladder cancers and leukemia among Finnish workers exposed to diesel or gasoline engine exhaust. Int J Cancer 111:286–292.
- Hemmingsen JG, Hougaard KS, Talsness C, Wellejus A, Loft S, Wallin H, Møller P. 2009. Prenatal exposure to diesel exhaust particles and effect on the male reproductive system in mice. Toxicology. 264:61–68.
- Holladay SD, Smith BJ. 1995. Benzo[a]pyrene-induced alterations in total immune cell number and cell-surface antigen expression in the thymus, spleen and bone marrow of B6C3F1 mice. Vet Human Toxicol. 37:99–104.
- Inoue S, Fujitani Y, Kiyono M, Hirano S, Takano H. 2011. In vitro effects of nanoparticle-rich diesel exhaust particles on splenic mononuclear cells. Immunopharm Immunot. 33:519–524.
- Karakaya A, Ates I, Yucesoy B. 2004. Effects of occupational polycyclic aromatic hydro-carbon exposure on T-lymphocyte functions and natural killer cell activity in asphalt and coke oven workers. Human Exp Toxicol. 23:317–322.
- Li CM, Takahashi S, Taneda S, Furuta C, Watanabe G, Suzuki AK, Taya K. 2006. Impairment of testicular function in adult male Japanese quail (Coturnix japonica) after a single administration of 3-methyl-4-nitrophenol in diesel exhaust particles. J Endocrinol. 189:555–564.
- Li CM. Takahashi S, Taneda S. Furuta C, Watanabe G, Suzuki AK, Taya K. 2007. Effects of 3-methyl-4-nitrophenol in diesel exhaust particles on the regulation of testicular function in immature male rats. J Androl. 28:252–258.
- Li Q, Hirata Y, Piao S, Minami M. 2000. Immunotoxicity of N, N-diethylaniline in mice: Effect on natural killer activity, cytotoxic T-lymphocyte activity, lymphocyte proliferation response, and cellular components of the spleen. Toxicology. 150:179–189.
- Li Q, Kobayashi M, Inagaki H, Hirata Y, Sato S, Ishizaki M, Okamura A, Wang D, Nakajima T, Kamijima M, Kawada T. 2010. Effect of oral exposure to fenitrothion and 3-methyl-4-nitrophenol on splenic cell populations and histopathological alterations in spleen in Wistar rats. Human Exp Toxicol. 30:665–674.
- Meier S, Andersen TE, Norberg B, Thorsen A, Taranger GL, Kjesbu OS, Dale R, Morton HC, Klungsøyr J, Svardal A. 2007. Effects of alkylphenols on the reproductive system of Atlantic cod (Gadus morhua). Aquat Toxicol. 81:207–218.
- Meier S, Morton HC, Andersson E, Geffen AJ, Taranger GL, Larsen M, Petersen M, Djurhuus R, Klungsøyr J, Svardal A. 2011. Low-dose exposure to alkylphenols adversely affects sexual development of Atlantic cod (Gadus morhua): Acceleration of onset of puberty and delayed seasonal gonad development in mature female cod. Aquat Toxicol. 105:136–150.
- Mi YL, Zhang CQ, Li CM, Taneda S, Watanabe G, Suzuki AK, Taya K. 2009. Quercetin protects embryonic chicken spermatogonial cells from oxidative damage intoxicated with 3-methyl-4-nitrophenol in primary culture. Toxicol Lett. 190:61–65.
- Miner KT, Croft M. 1998. Generation, persistence, and modulation of TH0 effector cells: Role of autocrine IL-4 and IFNγ. J Immunol. 160:5280–5287.
- Moon CK, Yun YP, Lee SH. 1986. Effects of some organophosphate pesticides on the murine immune system following subchronic exposure (II). Arch Pharm Res. 9:183–187.
- Mori Y, Kamata K, Toda N, Hayashi H, Seki K, Taneda S, Yoshino S, Sakushima A, Sakata M, Suzuki AK. 2003. Isolation of nitrophenols from diesel exhaust particles (DEP) as vasodilatation compounds. Biol Pharm Bull. 26:394–395.
- Müller L, Chehrazi C, Henderson M, Noah T, Jaspers I. 2013. Diesel exhaust particles modify natural killer cell function and cytokine release. Part Fibre Toxicol. 10:1–10.
- Murahashi T, Sasaki S, Nakajima T. 2003. Determination of endocrine disruptors in automobile exhaust particulate matter. J Health Sci. 49:72–75.
- Nakamura R, Inoue K, Fujitani Y, Kiyono M, Hirano S, Takano H. 2012. Effects of nanoparticle-rich diesel exhaust particles on IL–17 production in vitro. J Immunotoxicol. 9:72–76.
- Nakashima K, Yoshimura T, Mori H, Kawaguchi M, Adachi S, Nakao T, Yamazaki F. 2002. Effects of pesticides on cytokines production by human peripheral blood mononuclear cells – fenitrothion and glyphosate. Chudoku Kenkyu. 15:159–165.
- Ohtoshi T, Takizawa H, Okazaki H, Kawasaki S, Takeuchi N, Ohta K, Ito K. 1998. Diesel exhaust particles stimulate human airway epithelial cells to produce cytokines relevant to airway inflammation in vitro. J Allergy Clin Immun. 10:778–785.
- Ohyama M, Otake T, Adachi S, Kobayashi T, Morinaga K. 2007. A comparison of the production of reactive oxygen species by suspended particulate matter and diesel exhaust particles with macrophages. Inhal Toxicol. 19:157–160.
- Saito Y, Azuma A, Kudo S, Takizawa H, Sugawara I. 2002. Effects of diesel exhaust on murine alveolar macrophages and a macrophage cell line. Exp Lung Res. 28:201–217.
- Sasaki Y, Ohtani T, Ito Y, Mizuashi M, Nakagawa S, Furukawa T, Horii A, Aiba S. 2009. Molecular events in human T-cells treated with diesel exhaust particles or formaldehyde that underlie their diminished IFNγ and IL-10 production. Intl Arch Allergy Immunol. 148:239–250.
- Satoh K, Nonaka R, Ohyama K, Nagai F. 2005. Androgenic and anti–androgenic effects of alkylphenols and parabens assessed using the reporter gene assay with stably transfected CHO-K1 Cells (AR-EcoScreen System). J Health Sci. 51:557–568.
- Singh P, Madden M, Gilmour MI. 2005. Effects of diesel exhaust particles and carbon black on induction of dust mite allergy in Brown Norway Rats. J Immunotoxicol. 2:41–49.
- Sun HX, Qin F, Pan YJ. 2005. In vitro and in vivo immunosuppressive activity of Spica Prunellae ethanol extract on the immune responses in mice. J Ethnopharmacol. 101:31–36.
- Sureshkumar V, Paul B, Uthirappan M, Pandey R, Sahu AP, Lal K, Prasad AK, Srivastava S, Saxena A, Mathur N, et al. 2005. Pro-inflammatory and anti-inflammatory cytokine balance in gasoline exhaust induced pulmonary injury in mice. Inhal Toxicol. 17:161–168.
- Takano H, Yoshikawa T, Ichinose T, Miyabara Y, Imaoka K, Sagai M. 1997. Diesel exhaust particles enhance antigen-induced airway inflammation and local cytokine expression in mice. Am J Respir Crit. Care Med. 156:36–42.
- Tomita S, Maekawa SI, Rahman M, Saito F, Kizu R, Tohi K, Ueno T, Nakase H, Gonzalez FJ, Hayakawa K, et al. 2006. Thymic involution produced by diesel exhaust particles and their constituents in mice. Toxicol Environ Chem. 88:113–124.
- Vattanasit U, Navasumrit P, Khadka MB, Kanitwithayanun J, Promvijit J, Autrup H, Ruchirawat M. 2014. Oxidative DNA damage and inflammatory responses in cultured human cells and in humans exposed to traffic-related particles. Int J Hyg Environ Health. 217:23–33.
- Yin XJ, Ma JY, Antonini JM, Castranova V, Ma JK. 2004. Roles of reactive oxygen species and HO-1 in modulation of alveolar macrophage-mediated pulmonary immune responses to Listeria monocytogenes by diesel exhaust particles. Toxicol Sci. 82:143–153.
- Yoshimura A, Naka T, Kubo M. 2007. SOCS proteins, cytokine signaling and immune regulation. Nat Rev Immunol. 7:454–465.
- Yoshizaki K, Brito J, Moriya HT, Toledo AC, Ferzilan S, Ligeiro de Oliveira AP, Machado ID, Farsky SHP, Silva LFF, Martins MA, et al. 2015. Chronic exposure of diesel exhaust particles induces alveolar enlargement in mice. Respir Res. 16:1–10.
- Yue Z, She R, Bao H, Li W, Wang D, Zhu J, Chang L, Yu P. 2011. Exposure to 3-methyl-4-nitrophenol affects testicular morphology and induces spermatogenic cell apoptosis in immature male rats. Res Vet Sci. 91:261–268.