Abstract
Research in the treatment of gastric ulcer has involved the investigation of new alternatives, such as anti-depressant drugs. The present study was designed to investigate the gastroprotective effects of fluoxetine against indomethacin and alcohol induced gastric ulcers in rats and the potential mechanisms of that effect. Fluoxetine (20 mg/kg) was administered IP for 14 days. For comparative purposes, other rats were treated with ranitidine (30 mg/kg). Thereafter, after 24 h of fasting, INDO (100 mg/kg) or absolute alcohol (5 ml/kg) was administered to all rats (saline was administered to naïve controls) and rats in each group were sacrificed 5 h (for INDO rats) or 1 h (for alcohol rats) later. Macroscopic examination revealed that both fluoxetine and ranitidine decreased ulcer scores in variable ratios, which was supported by microscopic histopathological examination. Biochemical analysis of fluoxetine- or ranitidine-pre-treated host tissues demonstrated reductions in tumor necrosis factor (TNF)-α and myeloperoxidase (MPO) levels and concomitant increases in gastric pH, nitric oxide (NO) and reduced glutathione (GSH) contents. Fluoxetine, more than ranitidine, also resulted in serotonin and histamine levels nearest to control values. Moreover, immuno-histochemical analysis showed that fluoxetine markedly enhanced expression of cyclo-oxygenases COX-1 and COX-2 in both models; in comparison, ranitidine did not affect COX-1 expression in either ulcer model but caused moderate increases in COX-2 expression in INDO-induced hosts and high expression in alcohol-induced hosts. The results here indicated fluoxetine exhibited better gastroprotective effects than ranitidine and this could be due to anti-secretory, anti-oxidant, anti-inflammatory and anti-histaminic effects of the drug, as well as a stabilization of gastric serotonin levels.
Introduction
When the aggressive factors like gastric acid, pepsin and reactive oxygen species (ROS) overwhelm protective factors such as adequate mucosal blood flow, the mucosal bicarbonate barrier, endothelial cell regeneration and continuous prostaglandin production, this unbalance can lead to the generation of ulcers (Li et al. Citation2006). Cyclo-oxygenase enzymes, COX-1 and COX-2, both play an important role in mucosal defense. COX-1 is critical to the synthesis of prostaglandins such as prostacyclin (PGI2) and PGE2 that have protective effects for the gastric mucosa, i.e. they maintain gastric blood flow, turnover of mucosal cells and the synthesis and secretion of mucus (Pawlik et al. Citation2002). COX-2 inhibits leukocyte adherence and also helps to maintain gastric blood flow (Wallace & Miller Citation2000).
There are many endogenous and exogenous factors that can give rise to gastric ulcers. For example, the central nervous system (CNS) neurotransmitter serotonin (5-HT) is synthesized by enterochromaffin (EC) cells in the gastrointestinal tract. Abnormal regulation of 5-HT in the gut has been implicated in some GI disorders, including inflammatory bowel disease (Magro et al. Citation2002; Coates et al. Citation2004). Similarly, TNFα is a pro-inflammatory cytokine that is involved in the pathogenesis of gastric ulcers; it can cause apoptosis of gastric parietal cells (Neu et al. Citation2003) and act as an inhibitor of constitutive nitric oxide synthase (cNOS) (Bauer et al. Citation1997). This is important in that this could lead to reductions in local levels of NO, a vasodilator that maintains adequate blood flow to gastric mucosa (Pique et al. Citation1989). Lastly, neutrophils (PMN) and macrophages can generate reactive oxygen species (ROS) and stimulate the release of proteolytic enzymes and leukotrienes that also cause tissue damage (Whittle Citation2003; Jimenez et al. Citation2004) and so can contribute to gastric ulcer onset. Indomethacin, a widely-used non-steroidal anti-inflammatory drug, inhibits both COX isoforms and thereby exacerbates damage to gastric tissues (Delaney et al. Citation2007; Maity et al. Citation2009). Lastly, alcohol induces gastric lesions, in part by inhibition of anti-oxidant enzymes – leading to induction of oxidative stress, modulation of the NO system and reduction of gastric mucosal blood flow (Kim et al. Citation2005; Arafa & Sayed-Ahmed Citation2003).
Fluoxetine is a selective serotonin re-uptake inhibitor (SSRI) that is increasingly being used in the treatment of mental depression, panic disorders and alcoholism. It is generally believed to inhibit serotonin (5-hydroxytryptamine; 5HT) transporters (Cusack et al. Citation1994; Wong et al. Citation1995). Because ulcerations are associated with a depletion of serotonin in gastric tissues (Debnath & Guha Citation2007; Al Asmari et al. Citation2014), it became of interest to ascertain whether an agent like fluoxetine could also impart potential effects on ulcer induction/progression. Accordingly, the current study was designed to evaluate any gastroprotective effect of fluoxetine against indomethacin- and alcohol-induced ulcers specifically; underlying mechanisms of any effect, i.e. whether fluoxetine affected COX-1, COX-2, serotonin, and histamine levels as part of any protective effect, were also examined. For comparative purposes, ranitidine – an H2-receptor antagonist used in gastric acid disorder treatments – was also employed to not only compare efficacy of the drugs (Yeomans et al. Citation2006), but to also help clarify potential mechanisms of effect of fluoxetine.
Materials and methods
Materials
Fluoxetine hydrochloride was a generous gift from Amoun Pharmaceutical Industries, (Obour, Egypt). Ranitidine hydrochloride (Zantac; 25 mg/ml) was purchased from Glaxo Smith Kline, (Cairo, Egypt) and indomethacin (INDO) from Cairo Pharmaceutical Ind., (Cairo, Egypt). Absolute alcohol (99%) and sodium nitrite were purchased from El-Nasr company (Abuzabal, Egypt); sulfosalicylic acid was bought from Fluka (Buchs, Switzerland); N-(1-naphthyl)-ethylene-diamine was obtained from GIBCO (Grand Island, NY); sulfanilamide was purchased from Winlab (Leicestershire, UK); vanadium trichloride (VCl3) and o-phthalaldehyde were bought from Acros Organics (Trenton, NJ); reduced glutathione (GSH) was purchased from BDH (London, UK); and, bis-(3-carboxy-4-nitrophenyl) disulfide (DTNB), 3,3,5,5-tetra-methyl benzidine, serotonin and hexadecyltrimethyl ammonium bromide (HETAB) were all purchased from Sigma (St. Louis, MO). All chemicals were analytical grade and solutions used in the present study were all freshly prepared.
Experimental animals
Wistar rats (male, 180–200 g, 8-weeks-of-age) were obtained from the animal house at the College of Veterinary Medicine, Cairo University (Cairo). All rats were housed under specific pathogen-free conditions with a 12-h light:dark cycle at constant temperature (25 ± 2 °C) and provided ad libitum access to standard rodent chow (El-Nasr, Abuzabal, Egypt). All rats were acclimatized for 1 week prior to use in experiments. After this period, the rats were weighed and randomly allocated into eight experimental groups (n = 7/group). Rats in Group I were designated Control 1; Group II were INDO-induced ulcer; Group III were Ranitidine + INDO; Group IV were Fluoxetine + INDO; Group V were Control 2; Group VI were alcohol-induced ulcer; Group VII were Ranitidine + alcohol; and Group VIII were Fluoxetine + alcohol.
All animals were injected intraperitoneally (IP) once a day for 14 days prior to induction of gastric ulcer (see below). Rats in the control and ulcer groups were injected with 0.5 ml 0.9% saline each day. Rats in the ranitidine-pre-treated groups were injected with 30 mg ranitidine/kg IP (Saranya & Geetha Citation2011) for the 14 days. Rats in the fluoxetine pre-treated groups were injected with 20 mg fluoxetine/kg IP for the 14 days (Laakso et al. Citation1996). All procedures and experimental protocols complied with the Guide on the Care and Use of Laboratory Animals published by the National Institute of Health and Nutrition and were approved by the Scientific Research Ethics Committee of Tanta University.
Note: Readers may wonder why these studies used treatments (a) for 14-days, (b) only at a single dose level of fluoxetine, and (c) by the IP route. With regard to (a), because traditionally, the time needed for improvement of a clinical state in a patient taking an SSRI like fluoxetine is generally several weeks, it was assumed potential effects on the ulcer-related end-points would also require a period of adaptive changes in the host. Future studies will better refine any timeline needed for ‘positive’ effects to be achieved OR if, once the patient has adapted (assuming a 2 week initial treatment regimen) and gained beneficial anti-ulcer effects, the dosing can be relegated to an on-demand/shorter (albeit non-daily) regimen. With regard to (b), our own preliminary studies (as well as those of Laakso et al. (Citation1996), who performed dose-range studies of effects of fluoxetine on 5-HT receptor binding sites) found that doses of 10–20 mg/kg/day led to significant optimal effects. Future studies will refine (i.e. potentially lessen) dose levels needed for positive effects to again be achieved. Lastly, with regard to (c), although fluoxetine was developed as an oral use drug, the hydrochloride salt of the pure drug can affect gastric juice pH (i.e. increase acidity), resulting in false pH values. The changes in gastric pH would, in turn, affect hormones that control histamine release (Schubert & Peura Citation2008) and so affect histamine content in samples. In addition, fluoxetine causes dyspepsia/nausea in a percentage of subjects and so leads to decreased food intake/body weight loss. Any positive results from the IP studies here (in regard to impact on ulcers) could then lead pharmacologists/drug developers to develop alternate salts of the drug so that many of these untoward effects could be avoided while still imparting a beneficial anti-ulcer effect in humans. At that point, the studies here would be repeated using an oral dosing route to see if results using the IP regimen were then reproduced.
Induction of ulceration
All rats were fasted for 24 h in metabolic cages before ulcer induction to ensure their stomachs were empty. During the fasting period, rats were allowed to have free access to water only. On the day of ulcer induction, access to water was stopped for 2 h (Ismail et al. Citation2012). On Day 14, 30 min after the final IP injection of the various treatments/saline (Ji et al. Citation2012), Group 1 rats were administered 1 ml saline per os, while rats in Groups II–IV were given 100 mg INDO/kg; 5 h later, the rats were euthanized by ether asphyxiation (Araujo et al. Citation2011). In parallel, rats in Group V (Control 2) were administered 1 ml saline per os and those in Groups VI–VIII 5 ml/kg absolute alcohol; 1 h later, these rats were euthanized by ether asphyxiation (Vidya et al. Citation2001). In all cases, the stomach of each rat was excised, opened along the greater curvature and all the gastric juice was collected; thereafter, the tissue was rinsed with 0.9% saline. The excised stomach was then cut into two halves; one half was immediately fixed in 10% buffered formalin, while the remainder was cut into pieces, weighed and stored at −20 °C for later use in the various assays outlined below.
Determination of gastric mucosal injury
Gastric injury was determined by calculating ulcer score according to the method of Suleyman et al. (Citation2009a). Stomachs were tacked to a planer surface with small pins and then the total area of stomach and ulcerous areas were each traced using a cellophane sheet for analysis. The sum of the ulcerous areas was expressed as mm2 (ulcer score). Any drug anti-ulcer activity was evaluated by comparing the results obtained from the control vs treated groups using the formula: anti-ulcer effect (% protection) = 1 − [{ulcer score of treatment group/ulcer score of ulcer group} × 100].
Gastric pH determination
Gastric pH was determined via the method of Muniappan and Sundararaj (Citation2003). In brief, gastric juice that had been collected in a test tube was centrifuged at 3000 rpm for 10 min at 4 °C. From the supernatant, aliquots (0.5 ml each) were taken and diluted with 1 ml of distilled water and the pH of the solution was measured using a Model HI 110 pH meter (Hanna Instruments, Providence, RI).
Nitric oxide (NO) content
Gastric NO levels were determined via the method of Miranda et al. (Citation2001). Samples of isolated tissues (≈100 mg) were each homogenized in 10 vol ice-cold saline solution using a PT 3100 Polytron homogenizer (Kinematica instruments, Lucerne, Switzerland). Upon disruption, absolute ethanol was added (2:1 vol ratio) to precipitate all proteins. After allowing materials to separate over a 15 min period (at 25 °C), the supernatant was recovered. To 0.5 ml supernatant, 0.5 ml vanadium chloride (8 mg VCl3/ml) was added, rapidly followed by addition of 0.25 ml of 2% sulfanilamide and 0.25 ml of 0.1% N-(1-naphthyl)-ethylene diamine. The mixture was then vortexed and incubated at 37 °C for 30 min before its absorbance was measured at 540 nm in a Model UV-1601 PC spectrophotometer (Shimadzu, Kyoto, Japan). Sodium nitrite was dissolved in distilled water and serial dilutions were prepared; 0.5 ml of each dilution was used in place of tissue extract and processed as above. Values of NO in each sample were then determined by extrapolation from the prepared standard curve.
Serotonin content
The serotonin content was determined by the method of Schlumpf et al. (Citation1974). Samples of gastric tissue (≈200 mg) were homogenized in 5 ml HCl–butanol (0.85:1000 v/v) solution for 1 min and the sample was then centrifuged (10 min, 3000 rpm, 4 °C). From the resultant supernatant, 1 ml was removed and added to a tube containing 2.5 ml heptane and 0.31 ml of 0.1 M HCl. After 10 min of vigorous shaking, the tube was centrifuged (10 min, 3000 rpm, 4 °C) to separate the phases. The overlaying organic phase was removed and 1 ml of the aqueous phase was combined with 1.25 ml of a 20 mg % o-phthalaldehyde solution. The mixture was then heated to 100 °C for 10 min in a waterbath. After the samples were then cooled to room temperature, the fluorescence of each sample was measured at 360 nm excitation and 470 nm emission using a Model RF-5301PC fluorometer (Shimadzu). Serotonin was dissolved in distilled water and serial dilutions were prepared; 1 ml of each dilution was used in place of the tissue extract and processed as above. Values of serotonin in the samples were then determined by extrapolation from the prepared standard curve.
Histamine content
To determine tissue histamine levels, 100 mg gastric tissue was washed with 0.01 M PBS then homogenized in 10 vol ice water containing protease inhibitor cocktail (2 mM AEBSF, 1 mM EDTA, 130 μM bestatin, 14 μM E-64, 1 μM leupeptin and 0.3 μM aprotinin; Sigma) and 0.05% (v/v) Tween-20. The samples were then centrifuged at 5000 rpm for 10 min at 4 °C and the resultant supernatant was analyzed for histamine using a MyBio Source rat histamine ELISA kit (San Diego, CA) and measurements in a LMR-9602 plate reader (Labnics Equipment, Fremont, CA). The sensitivity of the kit was 80 pg histamine/ml.
Tumor necrosis factor (TNFα) content
To determine tissue TNFα levels, 250 mg gastric tissue was homogenized in 2.5 ml ice-cold phosphate-buffered saline (PBS, pH 7.4) containing protease inhibitor cocktail and 0.05% (v/v) Tween-20. The samples were then centrifuged at 3000 rpm for 10 min at 4 °C and the resultant supernatant was analyzed using an ALPCO Diagnostics rat TNFα ELISA kit (Salem, MA) and measurements in the plate reader. The sensitivity of the kit was 4 pg TNFα/ml.
Neutrophil infiltration
Myeloperoxidase (MPO) is a specific enzyme released by activated PMN (Frank et al. Citation2005). As such, it is often used as an indicator of PMN infiltration into gastric mucosal tissues (Grisham et al. Citation1990). For these analyses, 100 mg gastric tissue was homogenized in 10 vol ice-cold potassium phosphate buffer (pH 7.4). The homogenate was then centrifuged at 20 000 rpm for 20 min at 4 °C; the resulting pellets were then re-suspended in 10 vol ice-cold 50 mM potassium phosphate buffer (pH 6.0) containing 0.5% HETAB and 10 mM EDTA and then re-homogenized. An aliquot (50 μl) of this latter homogenate was diluted to 500 μl with the pH 6.0 buffer and then 200 μl of this solution was added to a 2 ml reaction volume containing 80 mM potassium phosphate buffer (pH 5.4), 0.5% HETAB and 1.6 mM tetramethyl benzidine. The mixture was then warmed to 37 °C before 200 μl of 0.3 mM H2O2 was added. The rate of change in absorbance was then continuously measured at 655 nm in the spectrophotometer.
Homogenate protein contents
Protein contents in each of the various gastric tissue homogenates were determined using a standard biuret assay system. In brief, 50 μl gastric tissue homogenate was added to 2 ml Biuret reagent, mixed well and incubated for 10 min at 37 °C. Thereafter, the samples were assessed at 550 nm in the Shimadzu spectrophotometer. As a standard, bovine serum albumin (BSA; Sigma) was dissolved in distilled water and serial dilutions prepared; 50 μl of each dilution was used in place of the tissue extract and processed as above. Protein levels in the samples were then determined by extrapolation from the prepared standard curve.
Reduced glutathione
Reduced glutathione (GSH) in the gastric tissues was determined using Ellman’s reagent. In brief, 250 mg gastric tissue was homogenized in 2.5 ml ice-cold 0.1 M phosphate buffer (pH 7.4) and then 4% sulfo-salicylate solution was added to precipitate proteins. The materials were then centrifuged at 3000 rpm for 10 min at 4 °C. To 0.5 ml of the resultant supernatant, 4.5 ml bis-(3-carboxy-4-nitrophenyl) disulfide (DTNB) reagent was added and the absorbance was measured at 412 nm in the spectrophotometer. Based on the extinction coefficient for the fluorophore generated, the amount of reduced GSH in the sample was calculated.
Histological and immunohistochemical analysis
The excised stomachs that had been fixed in 10% buffered formalin solution for 24 h were subsequently processed in ascending grades of alcohol and, finally, in xylene. The tissues were then embedded in paraffin, serially-sectioned to ≈4-μm thickness, mounted on slides and then stained with hematoxylin and eosin (H&E; Sigma). Samples then underwent histological examination in a blinded manner by a certified pathologist using a Model BM-2101 light miscroscope (Olympus, Yuyao, China).
For immunohistochemical detection of COX-1 and COX-2, an Ultra-Vision ONE HRP polymer detection system (ThermoFisher Scientific, Fremont, CA) was used, according to manufacturer protocols. Slides were de-paraffinized in pure xylene and then re-hydrated in alcohol. Endogenous peroxidase activity was blocked by incubating the sections in a 3% H2O2 solution for 30 min, then washing with PBS and incubation in 2% bovine serum albumin/PBS for 30 min at room temperature. The sections were then incubated overnight at 4 °C in a solution of PBS containing primary antibodies (1:100 dilution each) against COX-1 or COX-2. After this, each slide was washed three times with PBS and then each was incubated for 30 min with kit-provided One HRP polymer. Immunoreactive structures were developed through reactions with kit diaminobenzidine (DAB) substrate for 4–6 min at room temperature. The slides were then counterstained with hematoxylin, dehydrated in alcohol, cleared in xylene, and then underwent microscopic examination. Scoring was based upon the number of positive nuclei, i.e. 0%, 10%, 20% or 100% in each given section analyzed (a total of 150 mm/slide), with 10 sections/slide being examined per animal. Scores were then summed and averaged; group expression levels were reported as negative (±) (<10%), +1 (10–25%), +2 (25–50%), +3 (50–75%) or +4 (75–100%).
Statistical analysis
Results were expressed as the mean ± SD. Comparisons between different groups were carried out by one-way analysis of variance (ANOVA). For comparison between group body weight values, a Student’s paired t-test was used; all other parameters were analyzed using an independent t test. A p value < 0.05 was accepted as significant. Minitab computer software (v.17; Minitab Inc., State College, PA) was used to carry out these analyses.
Results
General effects of 14-day treatments with fluoxetine and ranitidine on rat hosts
Rats in Control groups 1 and 2 and in the INDO- and alcohol-only induced ulcer groups underwent significant increases in body weight by the end of the experiment (Day 14) (). On the other hand, rats that received treatment with ranitidine showed no significant change in body weight over this period. Conversely, rats that received fluoxetine had a significant reduction in body weight over the period. By the end of the experiment, all rats that had received either drug had body weights lower than the control or ulcer only groups. It was noted that fluoxetine-treated rats displayed reduced food intake and somnolence; rats that received ranitidine treatment did not show these effects.
Table 1. Animal body weights at Days 1, 7 and 14 of experiment.
Effect of fluoxetine on ulcer score
Indomethacin (INDO) and alcohol caused severe ulcerations of the gastric mucosa in rats. Pre-treatment with fluoxetine and ranitidine significantly reduced ulcer scores by 91.2% and 90.0%, respectively, compared with levels of damage induced by INDO alone, and significantly by 98.5% and 52.5%, respectively, compared to that occurring due to alcohol-induced damage. The effect of fluoxetine was not significantly different from that of ranitidine against the INDO-induced ulcer, but different in the treatment of the alcohol-induced ulcers (
Table 2. Effects on gastric ulcer score, pH, NO and serotonin contents.
Effect of fluoxetine on gastric juice pH
INDO and alcohol each caused significant decreases in gastric juice pH; the changes were by 29.2% and 32.8%, respectively, compared to the pH of juice from rats in Control Groups 1 and 2. Fluoxetine and ranitidine pre-treatment significantly increased pH values by, respectively, 51.4% and 49.7% that in the rats that received INDO alone and significantly so again by 43.3% and 32.8%, respectively, to that in the alcohol-only rats. With regard to this endpoint, fluoxetine imparted no significant difference from ranitidine in INDO-induced hosts, but did have a significantly different impact from ranitidine in alcohol-induced hosts ().
Effect of fluoxetine on gastric nitric oxide (NO) content
INDO and alcohol significantly decreased gastric tissue NO content by 53.1% and 77.1%, respectively, compared to the level of NO in tissues of rats in control Groups 1 and 2 (). Pre-treatment with fluoxetine and ranitidine significantly increased gastric tissue NO by 127.4% and 74.3%, respectively, relative to that in the INDO-only rats, and by 300.4% and 121.1% respectively, vs that from alcohol-only rats. Fluoxetine effects were significantly different from those of ranitidine in both ulcer models.
Effect of fluoxetine on gastric serotonin content
INDO and alcohol significantly decreased gastric tissue serotonin levels by, respectively, 53.6% and 71.0% compared to the content in tissues from control Group 1 and 2 rats (). Pre-treatment with fluoxetine and ranitidine significantly increased gastric serotonin by 121.5% and 96.2%, respectively, relative to that from rats that received INDO alone, and by 261.0% and 117.0%, respectively, vs that from alcohol-only rats. The effect of fluoxetine was significantly different from that of ranitidine in both ulcer models.
Effect of fluoxetine on gastric histamine content
INDO and alcohol significantly depleted gastric histamine content by 54.5% and 55.4%, respectively, compared to the levels seen in tissues of rats in control Groups 1 and 2 (
Table 3. Effects on gastric histamine, TNFα, MPO and GSH content.
Effect of fluoxetine on gastric TNFα content
INDO and alcohol both significantly increased gastric TNFα content. Specifically, levels were increased by, respectively, 263.0% and 322.7% compared to the TNFα levels in tissues in control Group 1 and 2 rats (). Pre-treatment with fluoxetine and ranitidine significantly decreased TNFα levels by 66.5% and 76.8%, respectively, relative to that in rats that received INDO alone, and by 81.3% and 53.7%, respectively, vs that in the alcohol-only rats. The effect of fluoxetine was significantly different from that of ranitidine in both ulcer models.
Effect of fluoxetine on gastric MPO content
INDO and alcohol both significantly increased gastric tissue MPO content by 109.1% and 161.7%, respectively, compared to the content seen with rats in control Groups 1 and 2 (). Pre-treatment with fluoxetine and ranitidine significantly decreased gastric tissue MPO by 58.2% and 43.5%, respectively, relative to that seen with rats that received INDO alone, and by 71.0% and 41.9%, respectively, vs that from alcohol only-treated rats. The effect of fluoxetine was significantly different from that of ranitidine in both ulcer models.
Effect of fluoxetine on gastric reduced GSH content
INDO and alcohol each altered the levels of reduced GSH relative to levels seen in tissues of rats in the control groups. INDO and alcohol significantly decreased GSH levels by, respectively, 78.1% and 85.4% compared to control values (). Pre-treatment with fluoxetine and ranitidine significantly increased GSH by 280.7% and 258.5%, respectively, relative to that in rats that received INDO alone, and by 524.5% and 217.7%, respectively, vs that in alcohol only-treated rats. Fluoxetine had no significantly different effect than ranitidine in the INDO-induced ulcer model, but did impart a significantly different one in the alcohol-induced hosts.
Effect of fluoxetine on gastric tissue histology
Rats in control Groups 1 and 2 had normal gastric mucosa architectures (). INDO-ulcerated rats presented tissues with deep ulcerations (), while those treated with alcohol presented with superficial ulcerations (). Ranitidine and fluoxetine pre-treatments decreased INDO-induced mucosal ulcerations and led to hosts having near-normal gastric architectures (). On the other hand, while fluoxetine pre-treatment led to a nearly normal architecture (), it could not prevent ulceration to the same extent as fluoxetine ().
Figure 1. Rat gastric mucosa. (A, E) Normal gastric mucosa in Control group 1 and 2 rats, respectively. (B) Deep ulceration with sloughing of tissue till the basal gland in INDO rat. (C) Healing of mucosa starting from the basal area of ulcer in ranitidine + INDO rat. (D) Healing of mucosa starting from the basal area of the ulcer in fluoxetine + INDO rat. (F) Superficial ulceration that does not involve whole thickness of mucosa, with mononuclear cell infiltration in alcohol-only rat. (G) Mild superficial ulceration with mononuclear cells infiltration in ranitidine + alcohol rat. (H) Healing from basal ulcer in fluoxetine + alcohol rat. Representative photo-micrographs from each group are shown. (H&E; magnification = 40×).
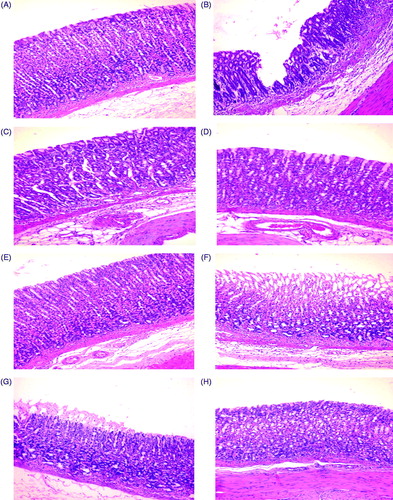
Effect of fluoxetine on COX-1 and COX-2 expression in gastric tissue
Immunohistochemical staining of gastric sections showed a constitutive expression of COX-1 in rats in Control groups 1 and 2 (). INDO completely inhibited COX-1 expression presented as lack of staining (); alcohol alone only reduced the expression to a mild-positive status (). Ranitidine pre-treatment did not improve COX-1 expression in either the INDO- or alcohol-induced ulcer models (). Fluoxetine pre-treatment did give rise to a moderate improvement in both models ().
Figure 2. COX-1 expression in rat gastric mucosa. (A, E) Highly positive (+++) constitutive expression of COX-1 in gastric mucosa of Control groups 1 and 2 rats, respectively. (B) Negative (±) expression in INDO rat. (C): negative (±) expression in ranitidine + INDO rat. (D) Moderate (++) expression in fluoxetine + INDO rat. (F) Weak positive (+) expression in alcohol-only rat. (G) Negative expression (±) in ranitidine + alcohol rat. (H) Moderate (++) expression in fluoxetine + alcohol rat. Representative photomicrographs from each group are shown. (PAP; magnification = 40×).
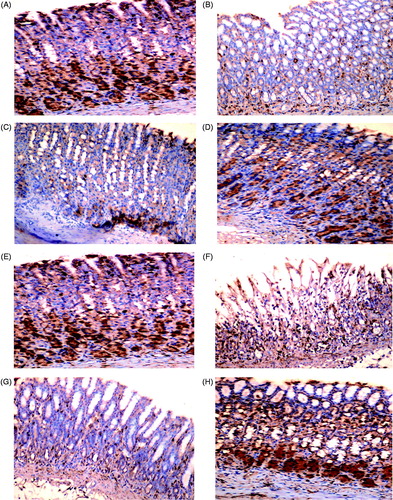
Immunohistochemical staining of gastric sections from rats in Control Groups 1 and 2 illustrated there was a high background expression of COX-2 (). INDO completely inhibited this expression (), while alcohol enhanced it around the rim of the ulcers (). Ranitidine pre-treatment led to only moderate COX-2 expression and healing of the gastric mucosa in INDO-induced ulcers (); conversely, this regimen led to a high COX-2 expression around the rim of the remaining ulcer in the alcohol-induced host tissues (). Fluoxetine pre-treatment led to markedly enhanced expression of COX-2 in both ulcer models () relative to in the INDO- or alcohol-only host tissues.
Figure 3. COX-2 expression in rat gastric mucosa. (A, E) Highly positive (+++) expression of COX-2 in gastric mucosa of Control groups 1 and 2 rats, respectively. (B) Negative (±) expression in INDO rat. (C) Moderate positive (++) expression in ranitidine + INDO rat. (D) Highly positive (+++) expression in fluoxetine + INDO rat. (F) Highly positive (+++) expression around the rim of the ulcer in alcohol-only rat. (G) Highly positive (+++) expression around remaining ulceration in ranitidine + alcohol rat. (H) Highly positive (+++) expression in fluoxetine + alcohol rat. Representative photomicrographs from each group are shown (PAP; magnification = 40×).
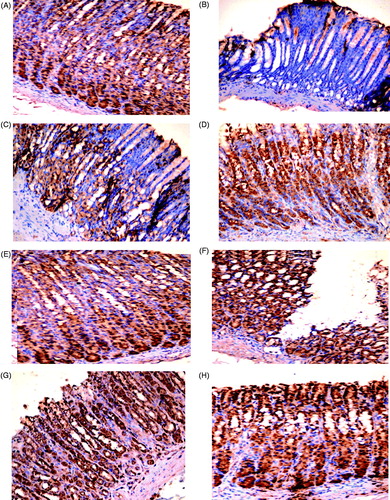
Discussion
Although reports have demonstrated that SSRI are associated with increased gastrointestinal bleeding (Dalton et al. Citation2003), many anti-depressant drugs, including SSRI, have been shown to impart anti-ulcer activity in various models of experimental ulcer (Dursun et al. Citation2009; Ji et al. Citation2012). In the study here, pre-treatment with fluoxetine significantly prevented mucosal ulcerations induced by INDO and alcohol. Macroscopic examinations – supported by histopathologic evaluations – here showed that the integrity of the gastric mucosa in fluoxetine-pre-treated rats was near normal and that the effect was more protective than that from ranitidine.
Endogenous 5-HT is involved in regulation of gastric function, i.e. it inhibits gastric acid (Stephens et al. Citation1989) while increasing mucous secretion (Guha & Ghosh Citation1995). The present results showed that INDO and alcohol depleted gastric serotonin content, an outcome in agreement with previous studies that found that ulcerations increased concomitant with a depletion of serotonin in gastric tissues (Debnath & Guha Citation2007; Al Asmari et al. Citation2014). The enteric nervous system, similar to the central nervous system, contains a serotonin re-uptake system and is also inhibited by serotonin re-uptake inhibitors (Gershon & Jonakait Citation1979). This could help explain why fluoxetine was able to let the rats maintain gastric serotonin content near control levels in both models, moreso than ranitidine was able to maintain. In addition, 5-HT is also known to induce satiety and reduce appetite (Halford et al. Citation2007); this could help explain the weight loss that was noted here in the fluoxetine-treated rats – as opposed to some untoward toxicity of the drug alone.
Besides helping to maintain gastric blood flow, NO protects against ulceration by activating the cyclooxygenase pathway and promoting prostaglandin synthesis. (Salvemini et al. Citation1993). The current results showed that fluoxetine significantly prevented reductions in NO levels induced by INDO and alcohol. The higher NO level in fluoxetine pre-treated rats compared to that caused by ranitidine in both models might be attributed to the ability of fluoxetine to maintain gastric serotonin content and a 5-HT2B receptor agonism by fluoxetine itself (Peng et al. Citation2014). The 5-HT2B receptor, distributed in the fundus and antrum (Komada & Yano Citation2007), activates constitutive and inducible NOS and stimulates NO production (Manivet et al. Citation2000). Also, fluoxetine stimulated NO production might be via NF-κB, an important upstream modulator of messenger RNA levels of nitric oxide synthase expression (Ha et al. Citation2006).
Many studies have considered COX-1 as playing a key role in the housekeeping of the gastric mucosa because of effects on PGE2 production (Xie et al. Citation1992; Ikari et al. Citation1999). Studies using SC-560, a selective COX-1 inhibitor, were used to show that only concurrent inhibition of both COX-1 and COX-2 allowed for gastric ulceration to evolve (Wallace et al. Citation2000). Moreover, COX-2 expression is markedly up-regulated in gastric ulcers and its inhibition results in a delay in ulcer healing processes (Perini et al. Citation2003) as COX-2 enhances angiogenesis and induces growth factors responsible for ulcer repair (Mizuno et al. Citation1997) through induction of the proliferation of epithelial cells during the repair process (Tarnawski Citation2005). Interestingly, COX-2 provides a “back-up source” for PG production when COX-1-induced PG synthesis is reduced (Shigeta et al. Citation1998). This suggests that, along with COX-1, the activity of COX-2 serves as another defense mechanism key to maintenance of mucosal integrity and ulcer healing.
In the present study, the gastric mucosa of control rats had ample expression of both COX-1 and COX-2, an outcome in agreement with that of Sun et al. (Citation2000). Fluoxetine markedly enhanced COX-1 expression, while ranitidine did not improve COX-1 in both models of ulcer induction. This could be explained by an ability of fluoxetine to increase plasma adrenaline (Blardi et al. Citation2005) that, in turn, is able to increase COX-1 levels in gastric tissue by stimulating α2 receptors (Suleyman et al. Citation2009b). The current results also showed that fluoxetine increased expression of COX-2 in both models, a finding in agreement with a previous study wherein fluoxetine up-regulated brain COX-2 expression (Wang et al. Citation2011). Fluoxetine, through the 5-HT2B receptor, transactivates epidermal growth factors receptors (EGF) (Li et al. Citation2008) that can, in turn, increase gastric blood flow at the ulcer margins and significantly up-regulate expression of COX-2 (but not COX-1) in ulcerated mucosa (Brzozowski et al. Citation2001). It is important to note that serotonin also activates COX pathways and stimulates PG synthesis through coupling of 5-HT2B and 5-HT2A receptors with an increase in the presence of free arachidonic acid (Tournois et al. Citation1998). As such, the increases in serotonin induced by the drug (relative to levels seen in hosts that only received ulcer-inducing agent) could also ultimately be contributing to the reparative/protective effects seen here.
Although ethanol induced elevated expression of COX-2 in gastric mucosa, the ulceration was still severe. Other reports have suggested that, in the presence of oxidative damage, PG could be converted into products of oxidation, such as 8-iso-PGF2α (Natale et al. Citation2004), that induce vasoconstriction and platelet aggregation that might enhance ulcerogenic actions of necrotizing agent and promote tissue oxidation (Sametz et al. Citation2000). The effect of fluoxetine on gastric acid secretion and subsequently higher gastric pH compared to values in the untreated ulcer counterparts may be secondary to its effect on NO and COX enzymes. Previous studies showed that NO and PGE2 inhibited gastric acid secretion (Berg et al. Citation2004; Kato et al. Citation2005).
Histamine binds to H2 receptors on parietal cells and stimulates acid secretion (El-Omar et al. Citation1997). Human and animal gastric ulcer studies found significantly less gastric mucosal histamine content in ulcerated hosts than in controls (Man et al. Citation1981; Cho et al. Citation1981). The experiment here showed that INDO and alcohol each depleted gastric mucosal histamine and that fluoxetine significantly increased it (as compared to in untreated ulcer counterparts). This could be related to the increase in gastric pH and inhibition of gastric secretion, i.e. this caused less histamine to be lost in gastric juice and prevented depletion of endogenous histamine (Man et al. Citation1981). Further, fluoxetine up-regulated COX-1 and COX-2 enzymes that synthesize PGE2 and – here – increased NO in both models compared to ranitidine. PGE2, its analogs and NO have previously been shown to impart an inhibitory effect on histamine release from entero-chromaffin like (ECL) cells (Kato et al. Citation1998; Lindstrom & Hakanson Citation1998; Freitas et al. Citation2004); this could explain the higher histamine content in gastric tissue and suggest that fluoxetine may have an anti-secretory effect.
INDO and alcohol up-regulated TNFα levels in ulcerated tissue and also led to enhanced PMN migration to the site. It is likely the latter occurred through increased expression of adhesion molecules on both PMN and endothelial cells, leading to tissue ischemia (Santucci et al. Citation1995). Results here showed that fluoxetine pre-treatment significantly prevented increases in TNFα levels in both models; this would support an anti-inflammatory effect of fluoxetine noted in previous studies (Abdel-Salam et al. Citation2003; Mozaffari et al. Citation2011). This ability of fluoxetine to inhibit TNFα formation/release may be attributed to its impact on serotonin, as serotonin can inhibit monocyte TNFα synthesis (Arzt et al. Citation1991) and up-regulate expression of COX iso-forms; as noted earlier, PGE2 can also inhibit production of TNFα (Menard et al. Citation2007). Fluoxetine also significantly inhibited INDO- and ethanol-induced increases in MPO levels here; this outcome was in agreement with a previous study that demonstrated an inhibitory effect of fluoxetine on MPO levels in irritable bowel syndrome (Kato et al. Citation1998). Fluoxetine also is known to inhibit the depletion in GSH to counter oxidative stresses produced during ulcer formation (Kirkova et al. Citation2010). This anti-oxidant effect of fluoxetine might be related to the fact the drug causes increases in levels of serotonin (which itself imparts an anti-oxidant effect; Ham et al. Citation1999).
Based on the data here, it appears ranitidine could not prevent alcohol-induced damage to the same extent it did against INDO-induced damage to the gastric mucosa. This may be attributed to the inhibitory effect of ranitidine on gastric alcohol dehydrogenase enzyme (Gugler Citation1994). Ranitidine inhibits first-pass metabolism and increases blood alcohol concentration after oral administration (Brown et al. Citation1995) that may exaggerate the damage induced by alcohol on gastric tissue. As a consequence, any anti-secretory activity ranitidine is not likely to be as effective/efficacious in preventing tissue damage completely.
Conclusions
The anti-ulcer effects of fluoxetine may be attributed to up-regulation of both cyclo-oxygenase enzymes in gastric tissue and nitric oxide (NO) levels. In addition, imparting of anti-inflammatory/anti-oxidant effects and causing a stabilization of histamine and serotonin levels that were depleted could also help explain the inhibitory effects on gastric acid secretion. In light of this investigation, fluoxetine might be considered to be a drug of choice in patients with gastric ulcers in the future. Nevertheless, while fluoxetine was beneficial in regard to reducing ulcer-related effects, this effect on host appetite should not be ignored going forward and steps to assuring proper caloric intake would need to be addressed.
Acknowledgments
The authors wish to express special thanks to the Faculty of Pharmacy at Tanta University for supporting this research. We also wish to thank Dr Karima El-Desouky (Professor of Pathology, Tanta University, Egypt) for her great assistance in the histopathological and immunohistochemical assays.
Disclosure statement
The authors report no conflicts of interest. The authors alone are responsible for the content and writing of the paper.
References
- Abdel-Salam O, Nofal S, El-Shenawy S. 2003. Evaluation of anti-inflammatory and anti-nociceptive effects of different anti-depressants in rat. Pharmacol Res. 48:157–165.
- Al Asmari A, Arshaduddin M, Elfaki I, Kadasah S, Al Robayan A, Al Asmary S. 2014. Aripiprazole an atypical anti-psychotic protects against ethanol induced gastric ulcers in rats. Intl J Clin Exp Med. 7:2031–2044.
- Arafa H, Sayed-Ahmed M. 2003. Protective role of carnitine esters against alcohol-induced gastric lesions in rats. Pharmacol Res. 48:285–290.
- Araujo D, Takayama C, de Faria F, Socca E, Dunder R, Manzo L, Luiz-Ferreira A. 2011. Gastro-protective effects of essential oil from Protium heptaphyllum on experimental gastric ulcer models in rats. Rev Braz Farmacogn. 4:721–729.
- Arzt E, Costas M, Finkielman S, Nahmod V. 1991. Serotonin inhibition of TNFα synthesis by human monocytes. Life Sci. 48:2557–2562.
- Bauer H, Jung T, Tsikas D, Stichtenoth D, Frolich J, Neumann C. 1997. Nitric oxide inhibits the secretion of TH1- and TH2-associated cytokines in activated human T-cells. Immunology. 90:205–211.
- Berg A, Redeen S, Ericson A, Sjöstrand S. 2004. Nitric oxide - an endogenous inhibitor of gastric acid secretion in isolated human gastric glands. BMC Gastroenterol. 4:1–9.
- Blardi P, de Lalla A, Auteri A, Iapichino S, Dell'Erba A, Castrogiovanni P. 2005. Plasma catecholamine levels after fluoxetine treatment in depressive patients. Neuropsychobiology. 51:72–76.
- Brown A, Fiaterone J, Day C, Bennett M, Kelly P, James O. 1995. Ranitidine increases bio-availability of post-prandial ethanol by reduction of first-pass metabolism. Gut. 37:413–417.
- Brzozowski T, Konturek P, Konturek S. 2001. Effect of local application of growth factors on gastric ulcer healing and mucosal expression of COX-1 and -2. Digestion. 64:15–29.
- Cho C, Ogle C, Wong S, Koo M. 1981. Effects of zinc sulfate on ethanol- and indomethacin-induced ulceration and changes in PGE2 and histamine levels in rat gastric glandular mucosa. Digestion. 32:288–295.
- Coates M, Mahoney C, Linden D, Sampson J, Chen J, Blaszyk H, Crowell M, Sharkey K, Gershon M, Mawe G, et al. 2004. Molecular defects in mucosal serotonin content and decreased serotonin re-uptake transporter in ulcerative colitis and irritable bowel syndrome. Gastroenterology. 126:1657–1664.
- Cusack B, Nelson A, Richelson E. 1994. Binding of anti-depressants to human brain receptors: Focus on newer generation compounds. Psychopharmacology. 114:559–565.
- Dalton S, Johansen C, Mellemkjaer L, Norgard B, Sorensen H, Olsen J. 2003. Use of selective serotonin reuptake inhibitors and risk of upper gastrointestinal tract bleeding: A population-based cohort study. Arch Intern Med. 163:59–64.
- Debnath S, Guha D. 2007. Role of Moringa oleifera on enterochromaffin cell count and serotonin content of experimental ulcer model. Indian J Exp Biol. 45:726–731.
- Delaney J, Opatrny L, Brophy J, Suissa S. 2007. Drug-drug interactions between anti-thrombotic medications and the risk of gastrointestinal bleeding. Can Med Assoc. 177:347–351.
- Dursun H, Bilici M, Albayrak F, Ozturk C, Saglam M, Alp H, Suleyman H. 2009. Anti-ulcer activity of fluvoxamine in rats and its effect on oxidant and anti-oxidant parameters in stomach tissue. BMC Gastroenterology. 9:1–10.
- El-Omar E, Oien K, El-Nujumi A, Gillen D, Wirz A, Dahill S, Williams C, Ardill J, McColl K. 1997. Helicobacter pylori infection and chronic gastric acid hypo-secretion. Gastroenterology. 113:15–24.
- Frank T, Grulke S, Deby-Dupont G, Deby C, Duvivier H, Peters F, Serteyn D. 2005. Development of an enzyme linked immunosorbent assay for specific equine neutrophil myelo-peroxidase measurement in blood. J Vet Diagn Invest. 17:412–419
- Freitas C, Baggio C, da Silva-Santos J, Rieck L, de Moraes Santos C, Júnior C, Ming L, Garcia Cortez D, Marques M. 2004. Involvement of nitric oxide in gastro-protective effect of aqueous extract of Pfaffia glomerata Pedersen, Amarnthanthaceae, in rats. Life Sci. 74:1167–1179.
- Gershon M, Jonakait G. 1979. Uptake and release of 5-HT by enteric 5-hydroxy-tryptaminergic neurons effect of fluoxetine and chlorimipramine. Br J Pharmacol. 66:7–9.
- Grisham M, Benoit J, Granger D. 1990. Assessment of leukocyte involvement during ischemia and re-perfusion of intestine. Meth Enzymol. 186:729–742.
- Gugler R. 1994. H2-antagonists and alcohol. Do they interact? Drug Safety. 10:271–280.
- Guha D, Ghosh A. 1995. Effect of cerebellar nodulation on gastro-duodenal PGE2 and 5-HT content of rat. Indian J Exp Biol. 33:361–364.
- Ha E, Jung K, Choe B, Bae J, Shin D, Yim S, Baik H. 2006. Fluoxetine increases the nitric oxide production via NF-κB-mediated pathway in BV2 murine microglial cells. Neurosci Lett. 397:185–189.
- Halford J, Harrold J, Boyland E, Lawton C, Blundell J. 2007. Serotonergic drugs: Effects on appetite expression and use for the treatment of obesity. Drugs. 67:27–55.
- Ham S, Kim D, Lee S, Kim Y, Lee C. 1999. Anti-oxidant effect of serotonin and L-DOPA on oxidative damage of brain synaptosomes. Korean J Physiol Pharmacol. 3:147–155.
- Ikari S, Sakai A, Tanaka A, Ikeda A, Inoue K, Takeguchi N. 1999. Prostaglandin E2-activated housekeeping Cl channels in the basolateral membrane of rat gastric parietal cells. Japan J Physiol. 49:365–372.
- Ismail I, Golbabapour S, Hassandarvish P, Hajrezaie M, Abdul Majid N, Kadir F, Al-Bayaty F, Awang K, Hazni H, Abdulla M. 2012. Gastro-protective activity of Polygnum chinense aqueous leaf extract on ethanol-induced hemorrhagic mucosal lesions in rats. Evid-Based Complement Alt Med. 2012:1–9.
- Ji C, Fan D, Li W, Guo L, Liang Z, Xu R, Zhang J. 2012. Evaluation of anti-ulcerogenic activity of the anti-depressants duloxetine, amitriptyline, fluoxetine and mirtazapine in different models of experimental gastric ulcer in rats. Eur J Pharmacol. 691:46–51.
- Jimenez M, Martín M, Alarcón de la Lastra C, Bruseghini L, Esteras A, Herrerías J, Motilva V. 2004. Role of L-arginine in ibuprofen-induced oxidative stress and neutrophil infiltration in gastric mucosa. Free Radic Res. 38:903–911.
- Kato S, Aihara E, Yoshii K, Takeuchi K. 2005. Dual action of PGE2 on gastric acid secretion through different EP receptor subtypes in the rat. Am J Physiol. G89:64–69.
- Kato S, Kitamura M, Korolkiewicz R, Takeuchi K. 1998. Role of nitric oxide in regulation of gastric acid secretion in rats: Effect of NO donor and NO synthase inhibitors. Br J Pharmacol. 123:839–846.
- Kim J, Choi S, Choi S, Kim H, Chang H. 2005. Suppressive effect of astaxanthin isolated from xanthophyllomyces dendrorhous mutant on ethanol–induced gastric mucosal injury in rats. Biosci Biotechnol Biochem. 69:1300–1305.
- Kirkova M, Tzvetanova E, Vircheva S, Zamfirova R, Grygier B, Kubera M. 2010. Anti-oxidant activity of fluoxetine: studies in mice melanoma model. Cell Biochem Funct. 28:497–502.
- Komada T, Yano S. 2007. Pharmacological characterization of 5-hydroxytryptamine-receptor subtypes in circular muscle from the rat stomach. Biol Pharm Bull. 30:508–513.
- Laakso A, Pälvimäki E, Kuoppamäki M, Syvälahti E, Hietala J. 1996. Chronic citalopram and fluoxetine treatments up-regulate 5-HT2C receptors in rat choroid plexus. Neuropsycho-pharmacology. 15:143–151.
- Li B, Zhang S, Zhang H, Nu W, Cai L, Hertz L, Peng L. 2008. Fluoxetine-mediated 5-HT2B receptor stimulation in astrocytes causes EGF receptor transactivation and ERK phosphorylation. Psychopharmacology (Berlin). 201:443–458.
- Li Y, Lu G, Zou X, Li Z, Peng G, Fang D. 2006. Dynamic functional and ultrastructural changes of gastric parietal cells induced by water immersion-restraint stress in rats. World J Gastroenterol. 12:3368–3372.
- Lindstrom E, Hakanson R. 1998. Prostaglandins inhibit secretion of histamine and pancreastatin from isolated rat stomach ECL cells. Br J Pharmacol. 124:1307–1313.
- Magro F, Vieira-Coelho M, Fraga S, Serrão M, Veloso F, Ribeiro T, Soares-da-Silva P. 2002. Impaired synthesis or cellular storage of norepinephrine, dopamine, and 5-hydroxytrypta-mine in human inflammatory bowel disease. Dig Dis Sci. 47:216–224.
- Maity P, Bindu S, Dey S. 2009. Indomethacin, a non-steroidal anti-inflammatory drug, develops gastropathy by inducing reactive oxygen species-mediated mitochondrial pathology and associated apoptosis in gastric mucosa: A novel role of mitochondrial aconitase oxide-tion. J Biol Chem. 284:3058–3068.
- Man W, Saunders J, Ingoldby C, Spencer J. 1981. Effect of cimetidine on amounts of histamine in gastric mucosa of patients with gastric or duodenal ulcers. Gut. 22:923–926.
- Manivet P, Mouillet-Richard S, Callebert J, Nebigil C, Maroteaux L, Hosoda S, Kellermann, O, Launay J. 2000. PDZ-dependent activation of nitric oxide synthase by the serotonin-2B receptor. J Biol Chem. 275:9324–9331.
- Ménard G, Turmel V, Bissonnette E. 2007. Serotonin modulates the cytokine network in the lung: Involvement of PGE2. Clin Exp Immunol. 150:340–348.
- Miranda K, Espey M, Wink D. 2001. A rapid simple spectrophotometric method for simultaneous detection of nitrate and nitrite. Nitric Oxide. 5:62–71.
- Mizuno H, Sakamoto C, Matsuda K, Wada K, Uchida T, Noguchi H, Akamatsu T, Kasuga M. 1997. Induction of COX-2 in gastric mucosal lesion and its inhibition by specific antagonist delay healing in mice. Gastroenterology. 112:387–397.
- Mozaffari S, Esmaily H, Rahimi R, Baeeri M, Sanei Y, Asadi-Shahmirzadi A, Salehi-Surmaghi MH, Abdollahi M. 2011. Effects of Hypericum perforatum extract on rat irritable bowel syndrome. Pharmacogn Mag. 7:213–223.
- Muniappan M, Sundararaj T. 2003. Anti-inflammatory and anti-ulcer activities of Bambusa arundinacea. Ethnopharmacology. 88:161–167.
- Natale G, Lazzeri G, Lubrano V, Colucci R, Vassalle C, Fornai M, Blandizzi C, Del Tacca M. 2004. Mechanisms of gastroprotection by lansoprazole pre-treatment against experimentally induced injury in rats: Role of mucosal oxidative damage and sulfhydryl compounds. Toxicol Appl Pharmacol. 195:62–72.
- Neu B, Puschmann A, Mayerhofer A, Hutzler P, Grossmann J, Lippl F, Schepp W, Prinz C. 2003. TNFα induces apoptosis of parietal cells. Biochem Pharmacol. 65:1755–1760.
- Pawlik T, Konturek P, Konturek J, Konturek S, Brzozowski T, Cześnikiewicz M, Plonka M, Bielanski W, Areny H. 2002. Impact of Helicobacter pylori and non-steroidal anti-inflammatory drugs on gastric ulcerogenesis in experimental animals and in humans. Eur J Pharmacol. 449:1–15.
- Peng L, Gu L, Li B, Hertz L. 2014. Fluoxetine and all other SSRIs are 5-HT2B agonists - importance for their therapeutic effects. Curr Neuropharmacol. 12:365–379.
- Perini R, Ma L, Wallace J. 2003. Mucosal repair and COX-2 inhibition. Curr Pharm Des. 9:2207–2211.
- Pique J. Whittle B, Esplugues J. 1989. The vasodilator role of endogenous nitric oxide in the rat gastric microcirculation. Eur J Pharmacol. 174:293–296.
- Salvemini D, Misko T, Masferrer J, Seibert K, Currie M, Needleman P. 1993. Nitric oxide activates cyclooxygenase enzymes. Proc Natl Acad Sci USA. 90:7240–7244.
- Sametz W, Hennerbichler S, Glaser S, Wintersteiger R, Juhan H. 2000. Characterization of prostanoid receptors mediating action of the 8-iso-PGF2 and 8-iso-PGF2α, in the smooth muscle preparation. Br J Pharmacol. 130:1903–1910.
- Santucci L, Fiorucci S, DiMatteo F, Morelli A. 1995. Role of TNFα release and leucocyte margination in indomethacin-induced gastric injury in rats. Gastroentrology. 108:3932–3401.
- Saranya P, Geetha A. 2011. Anti-ulcer activity of Andrographis paniculata (Burm. f.) waal. against cysteamine-induced duodenal ulcer in rats. Indian J Exp Biol. 49:525–533.
- Schlumpf M, Lichtensteiger W, Langemann H, Waser P, Hefti F. 1974. A fluorometric micro-method for the simultaneous determination of serotonin, noradrenaline, and dopamine in milligram amounts of brain tissue. Biochem Pharmacol. 23:2437–2446.
- Schubert ML, Peura DA. 2008. Review in basic and clinical gastroenterology: Control of gastric acid secretion in health and disease. Gastroenterology. 134:1842–1860.
- Shigeta J, Takahashi S, Okabe S. 1998. Role of cyclooxygenase-2 in the healing of gastric ulcers in rats. J Pharmacol Exp Therapeut. 286:1383–1390.
- Stephens R, Garrick T, Weiner H, Tache Y. 1989. Serotonin depletion potentiates gastric secretory and motor responses to vagal but not peripheral gastric stimulants. J Pharmacol Exp Ther. 251:524–530.
- Suleyman H, Cadirci E, Hacimuftuoglu A. 2009a. Comparative study of gastroprotective potential of some anti-depressants in indomethacin-induced ulcer in rats. Chem Biol Int. 180:318–324.
- Suleyman H, Dursun H, Bilici M. 2009b. Relation of adrenergic receptors, which have roles in gastroprotective and anti-inflammatory effect of adrenal gland hormones, with cyclo-oxygenase enzyme levels in rats. J Physiol Pharmacol. 60:129–134.
- Sun W, Tsuji S, Tsujii M. 2000. Induction of COX–2 in rat gastric mucosa by rebamipide, a muco-protective agent. J Pharmacol Exp Ther. 295:447–452.
- Tarnawski A. 2005. Cellular and molecular mechanisms of gastrointestinal ulcer healing. Digest Dis. 50(Suppl 1):S24–S33.
- Tournois C, Mute V, Manivet P. Launay J, Kellermann O. 1998. Cross-talk between 5-hydroxytryptamine receptors in a serotonergic cell line: involvement of arachidonic acid metabolism. J Biol Chem. 273:17498–17503.
- Vidya S, Ramesh A, Alekhya A, Lohitha N. 2001. Anti-ulcer activity of Eugenia Jambolana leaves against ethanol-induced gastric ulcer in albino rats. J Pharmaceut Res Dev. 3:106–112.
- Wallace J, Miller M. 2000. Nitric oxide in mucosal defense. A little goes a long-way. Gastroenterology. 119:512–520.
- Wallace J, McKnight W, Reuter B, Vergnolle N. 2000. NSAID-induced gastric damage in rats: Requirement for inhibition of both COX-1 and COX-2. Gastroenterology. 119:706–714.
- Wang Y, Yang F, Liu Y, Gao F, Jiang W. 2011. Acetylsalicylic acid as an augmentation agent in fluoxetine treatment resistant depressive rats. Neurosci Lett. 499:74–79.
- Whittle B. 2003. Gastrointestinal effects of non-steroidal anti-inflammatory drugs. Fundam Clin Pharmacol. 17:301–313.
- Wong D, Bymaster F, Engleman E. 1995. Prozac (fluoxetine, Lilly 110140), the first selective serotonin uptake inhibitor and an anti-depressant drug: 20 years since its first publication. Life Sci. 57:411–441.
- Xie W, Robertson D, Simmons D. 1992. Mitogen-inducible prostaglandin G/H synthase: New target for non-steroidal anti-inflammatory drugs. Drug Dev Res. 25:249–265.
- Yeomans N, Sevedberg L, Naesdal J. 2006. Is ranitidine therapy sufficient for healing peptic ulcers associated with non-steroidal anti-inflammatory drug use? Intl J Clin Pract. 60:1401–1407.