Abstract
Background and purpose — The circadian clock governs endogenous day-night variations. In bone, the metabolism and growth show diurnal rhythms. The circadian clock is based on a transcription-translation feedback loop composed of clock genes including Period2 (Per2), which encodes the protein period circadian protein homolog 2. Because plasma parathyroid hormone (PTH) levels show diurnal variation, we hypothesized that PTH could carry the time information to bone and cartilage. In this study, we analyzed the effect of PTH on the circadian clock of the femur.
Patients and methods — Per2::Luciferase (Per2::Luc) knock-in mice were used and their femurs were organ-cultured. The bioluminescence was measured using photomultiplier tube-based real-time bioluminescence monitoring equipment or real-time bioluminescence microscopic imaging devices. PTH or its vehicle was administered and the phase shifts were calculated. Immunohistochemistry was performed to detect PTH type 1 receptor (PTH1R) expression.
Results — Real-time bioluminescence monitoring revealed that PTH reset the circadian rhythm of the Per2::Luc activity in the femurs in an administration time-dependent and dose-dependent manner. Microscopic bioluminescence imaging revealed that Per2::Luc activity in the growth plate and the articular cartilage showed that the circadian rhythms and their phase shifts were induced by PTH. PTH1R was expressed in the growth plate cartilage.
Interpretation — In clinical practice, teriparatide (PTH (1-34)) treatment is widely used for osteoporosis. We found that PTH administration regulated the femoral circadian clock oscillation, particularly in the cartilage. Regulation of the local circadian clock by PTH may lead to a more effective treatment for not only osteoporosis but also endochondral ossification in bone growth and fracture repair.
Parathyroid hormone (PTH) is an 84-amino acid peptide hormone produced by the parathyroid gland. There are 2 known PTH receptors, PTH1R (also known as PTH/PTH related-peptide (PTHrP) receptor) and PTH2R. PTH1R is highly expressed in the bones and kidneys whereas PTH2R is mostly expressed in the central nervous system (CitationKopic and Geibel 2013). The bioactivity of PTH comes from the 34 N-terminal amino acids (PTH (1-34)) (Potts et al. 1971). Teriparatide (recombinant human PTH (1-34)) is a bone anabolic agent for treatment of patients with osteoporosis (CitationAugustine and Horwitz 2013). In osteoporosis patients, morning teriparatide treatment appears to function better than night-time administration, but the detailed biological mechanism is not fully understood (CitationMichalska et al. 2012).
The circadian clock governs the endogenous day-night variations. At the molecular level, the circadian clock is based on a transcription-translation feedback loop composed of clock genes such as Per1-2, Cry1-2, Clock, Bmal1, RevErba, RevErbb, Rora, and Dec1-2 (CitationShearman et al. 2000, CitationLowrey and Takahashi 2011). The circadian clock in the suprachiasmatic nucleus of the clock center, in peripheral tissues, and even in cultured cell lines share essentially the same mechanism (CitationYagita et al. 2001). Bone and cartilage also have the circadian clocks (CitationIimura et al. 2012, CitationDudek and Meng 2014, CitationGossan et al. 2014). CitationGossan et al. (2013) showed that there are circadian clocks in cultured chondrocytes and in articular cartilage tissue. We showed that the growth plate and articular cartilage have a circadian rhythm, using the EM-charge-coupled device (CCD) cameras (CitationOkubo et al. 2013).
The suprachiasmatic nucleus is believed to orchestrate the circadian clock in peripheral tissues through hormonal and/or neuronal regulation (CitationBalsalobre et al. 2000, CitationTerazono et al. 2003, CitationYoo et al. 2004). CitationFu et al. (2005) found that osteoblasts have a functional molecular clock, and suggested that time information may be carried to bones via a leptin-parasympathetic neuron-bone pathway. The circadian rhythm of bioluminescence in cartilage can be reset with forskolin or synthesized glucocorticoid (dexamethasone) (CitationGossan et al. 2013, CitationOkubo et al. 2013). Interestingly, plasma PTH levels have been reported to show day-night variations under normal light-dark conditions (CitationJubiz et al. 1972) and also under “constant routine” conditions, where no external time information is available (el-Hajj Fuleihan et al. 1997). CitationHinoi et al. (2006) reported that PTH induces Per1 and Per2 expression in organ-cultured fetal metatarsal. We therefore hypothesized that PTH could carry time information to bone and cartilage. In this study, we investigated the effect of PTH on the femoral circadian clock.
Material and methods
Animals and femur sampling
Period2::Luciferase (Per2::Luc) knock-in mice were originally developed by Dr Joseph Takahashi’s group (CitationYoo et al. 2004). Mice were maintained by intercrossing with their littermates and backcrossing to C57BL/6 mice at our facility. They were kept under 12-h light-dark conditions, and food and water were available ad libitum. For tissue sampling, the mice were deeply anesthetized with isoflurane and killed. We used both male and female mice (4–15 weeks old), as no sex and age differences have been observed in the circadian clock period or phase (CitationGossan et al. 2013, CitationOkubo et al. 2013). After sterilization with 70% ethanol, femurs were collected and stored in ice-cold PBS. Muscle tissue and tendons were removed.
Femoral organ culture
Femoral organ culture was performed as previously reported (CitationOkubo et al. 2013). In brief, collected femurs were placed in 35-mm cell culture dishes. The culture medium contained phenol red-free D-MEM (Nacalai Tesque, Kyoto, Japan), 1× Glutamax (Life Technologies, Carlsbad, CA), 1 × B-27 supplement (Life Technologies), 10 mM HEPES, 200 μM beetle luciferin (Promega KK, Tokyo, Japan), 100 units/mL penicillin and 100 μg/mL streptomycin (Nacalai Tesque).
Real-time bioluminescence monitoring and PTH stimulation
Femurs were synchronized with forskolin (10 μM) for 1 h and then released into fresh culture medium as previously described (CitationGibbs et al. 2009, CitationOkubo et al. 2013) The tightly sealed culture dishes were placed under the photomultiplier tube-based real-time bioluminescence monitoring equipment, kept at 35˚C, and monitored every 20 min. Human PTH (1-34) (Peptide Institute, Inc., Ibaraki, Japan) was dissolved in distilled water. PTH (1-34) or its vehicle was added and the femurs were set under the bioluminescence counting device again. To study the effect of PTH on the femoral circadian clock, we administered PTH after the second peak (41 h after synchronization) or the trough of Per2::Luc activity (53 h after synchronization).
Microscopic observation
Microscopic bioluminescence observation was performed using a high-sensitivity CCD camera-based microscopic image analyzer and bioluminescence macroscopy (Olympus, Tokyo, Japan). After synchronization, femurs were placed under the CCD camera-based device. After 40 h of observation, 10-7 M PTH (1-34) or its vehicle was added and the femurs were placed under the device again.
Immunohistochemistry
Immunohistochemistry was performed by New Histo. Science Laboratory Co., Ltd. (Tokyo, Japan). Briefly, the femurs were fixed with 4% paraformaldehyde for 8 h, which was followed by treatment with 70% ethanol for 3–4 days. The femurs were demineralized with 10% EDTA for 2–4 weeks, and then embedded in paraffin and sliced into 3- or 4-µm sections. After deparaffinization, the samples were incubated in 3% H2O2 for 5 min, washed with 0.01 M PBS, and incubated for 50 min at room temperature in rabbit polyclonal anti-parathyroid hormone receptor 1 antibody (Acris Antibodies GmbH, Herford, Germany) diluted 1:150 in 0.01 M PBS. In the negative controls, anti-rabbit IgG was used instead of the primary antibody. For the secondary antibody, the samples were incubated in HistostarTM mouse (Rb) (Immunostaining Reagent, Anti-Rabbit) (Medical & Biological Laboratories Co., Ltd., Nagoya, Japan) for 5 min and then washed with 0.01 M PBS, followed by visualization of any antibody binding with DAB-peroxidase. Finally, the samples were counterstained with hematoxylin.
Data analysis
For detection of phase shift, the data were smoothened by a 24-h moving average and the damping effect was corrected by using a 24-h standard deviation (CitationIzumo et al. 2006). The difference in the periods between pre- and post-stimulation was calculated. The circadian period was defined as the peak-to-peak interval. Statistical analysis was performed with 1-way ANOVA followed by Dunnett’s test.
Ethics
Animal experiments were performed with approval from the Experimental Animals Committee, Kyoto Prefectural University of Medicine (date of issue: April 1, 2012; registration number: M24-240).
Results
When a low concentration of PTH (10-11 M) was administered 41 h after synchronization, the circadian bioluminescence rhythm did not show any significant changes compared to vehicle administration. From administration of medium (10-9 M) and high concentrations of PTH (10-7 M), however, we observed significant phase advances of the bioluminescence rhythm (the next peak occurred earlier than expected); 10-9 M PTH induced a phase advance of 3.22 (SD 0.63) h and 10-7 M PTH induced a phase advance of 7.69 (SD 1.15) h (). The effect on the circadian bioluminescence rhythm was different when PTH was administered 53 h after synchronization. A low concentration (10-11 M) of PTH did not induce significant phase shift compared to the vehicle, but at medium (10-9 M) and high concentrations (10-7 M), PTH induced phase delays (−4.03 (SD 0.71) h and −3.05 (SD 0.40) h, respectively) ().
Figure 1. The effects of PTH on the femoral circadian clock. A–B. Phase shift by vehicle, 10-11 M PTH, 10-9 M PTH, and 10-7 M PTH at 41 h (A) and 53 h after synchronization (B). Representative data are shown. Arrowheads indicate PTH administrations. C–D. Quantitative analysis of the phase shift at 41 h (C) and 53 h after synchronization (D). Data are mean ± SEM. The numbers of samples are shown in parentheses.
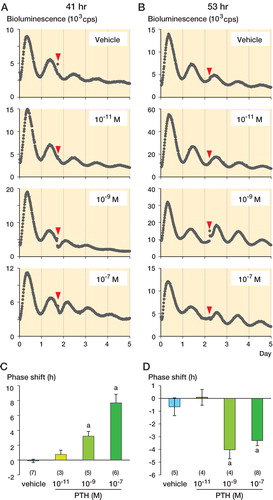
To elucidate the spatial distribution of bioluminescence, we studied the effect of PTH on the circadian clock in femurs using the CCD camera-based device (). We administered 10-7 M PTH 41 h after synchronization. This is because the largest phase shift was evoked under this condition (). Before administration of PTH, strong Per2::Luc signals were observed in cartilaginous tissues such as the epiphyseal cartilage of the growth plate and articular cartilage, whereas Per2::Luc signals in the ossified bone were extremely weak, as we have reported previously (CitationOkubo et al. 2013) (). No obvious change was observed from treatment with vehicle, and the next peak came 16 h after administration (). With PTH treatment, signal in the growth plate gradually increased and peaked 8 h after PTH administration, and then continued circadian oscillations (). Similar PTH-induced phase shifts were observed in the femoral head, the greater and lesser trochanter, and the distal end (data not shown). We also confirmed that PTH1R was expressed in the growth plate cartilage, by immunohistochemistry ().
Figure 2. The PTH-induced phase shift observed with the CCD camera-based device. A. Microscopic images of the femur. A bright-field image (left), bioluminescence image (middle), and overlaid image (right) are shown. The bioluminescence signal is shown in yellow. B. Bioluminescence images taken every 8 h indicate phase shift of the circadian rhythm in the distal femur. Femurs treated with PTH (upper row) and vehicle (lower row) are shown. C–D. Quantitative analysis of the distal growth plate. ROI was set at the distal growth plate (C). Results of a time series analysis of the relative signal intensity are also shown (D). The data were detrended by a 24-h moving average. The arrowhead indicates chemical stimulation. E. Immunohistochemistry of PTH1R. From left to right: negative control at low magnification, negative control at high magnification, anti-PTH1R at low magnification, and anti-PTH1R at high magnification. The squares at low magnification indicate the areas shown at high magnification. Black bars at low and high magnification indicate 500 µm and 10 µm, respectively.
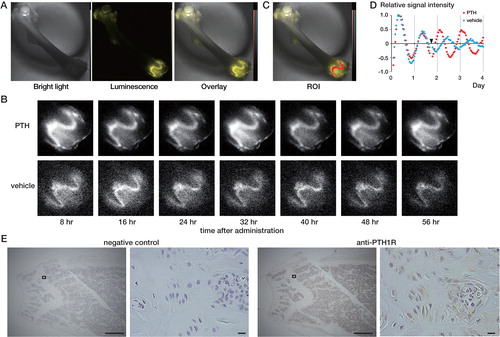
Discussion
We found that PTH reset the circadian rhythm of the Per2::Luc activity in the femurs in both a time-dependent and a dose-dependent manner. By CCD camera-based imaging analysis, the phase shift of Per2::Luc activity was observed in the growth plate and articular cartilage. These results suggest that PTH induces the phase shift of the circadian clock of cartilage. In the suprachiasmatic nucleus, a light pulse induces Per1 and Per2 mRNA expression and resets the circadian clock in both a time-dependent and an intensity-dependent manner (CitationShigeyoshi et al. 1997). CitationField et al. (2000) reported that light induced both Per mRNA expression and Per protein expression in the suprachiasmatic nucleus, although protein peaks came a few hours later. Similarly, PTH administration has been reported to induce Per1 and Per2 mRNA expression in chondrogenic cells or tissues. CitationHinoi et al. (2006) observed that PTH administered at 10-7 M induced both Per1 and Per2 promoter activity in chondrogenic ATDC5 cells via the cAMP-PKA-CRE pathway. They also showed that both Per1 mRNA expression and Per2 mRNA expression were induced 1–2 hours after administration of 10-7 M PTH to organ-cultured fetal metatarsals. Taken together with our data, this indicates that PTH may reset the circadian clock of cartilage through alteration in levels of expression of Per mRNA and protein.
Not only PTH, but also PTHrP can exert physiological effects through the activation of PTH1R (CitationKopic and Geibel 2013). In the growth plate, where we confirmed PTH1R expression, hormones and paracrines including PTHrP tightly control endochondral ossification (CitationLanske et al. 1996, CitationKronenberg 2003). Interestingly, the bone length of both PTHrP knock-out mice and clock gene Bmal1 knock-out mice is shorter (CitationSchipani et al. 1997, CitationBunger et al. 2005). PTHrP synthesis is mainly regulated by Indian hedgehog (IHH) in the growth plate (CitationChung et al. 2001). CitationTakarada et al. (2012) reported that the expression of Ihh clearly shows the circadian rhythm. Our findings raise the possibility that PTHrP carries time information to cartilage.
Despite the increasing number of studies on the cartilage circadian clock, the local clock function still remains unclear. Importantly, bone length of the chondrocyte-specific Bmal1-deficient mouse is shorter than that of its wild-type equivalent (CitationTakarada et al. 2012). Since the secretion of the systemic hormones that control bone growth presumably has normal diurnal rhythms in such circumstances, the local circadian clock must have a role as a regulating mechanism in bone growth. Our results suggest that the local circadian clock is regulated by PTH. Thus, the regulation of the local circadian clock by PTH may lead to a treatment for diseases of bone growth.
In clinical practice, teriparatide is widely used for treatment of osteoporosis, but not in young patients with bone growth disease. Endochondral ossification occurs not only in the growth plate but also in the fracture site. Teriparatide treatment has been found to accelerate fracture healing, due to an increase in chondrocyte recruitment and in the rate of differentiation, in a mouse fracture model (CitationKakar et al. 2007). Our results suggest that fracture repair may be a target of chronotherapy using teriparatide, and further studies on the circadian clock of cartilage are therefore needed to determine the most effective timing of teriparatide administration.
In summary, we have demonstrated that PTH administration induced phase shifts of oscillation of the circadian clock in the growth plate and articular cartilage of a bone organ culture system. These results suggest that PTH regulates the circadian clock of cartilage.
KY, NO, YM, and T Kubo conceived and designed the experiments. NO, YM, TKun, TH, and HI performed the experiments. NO, HF, YM, TKun, TH, and YU analyzed the data. NO, HF, YM, MA, TKub, and KY wrote the paper. All the authors approved the final manuscript.
We thank Ms Yoko Hatta-Ohashi (Olympus) for setting up the bioluminescence macroscopy. This work was supported in part by the PRESTO Program of the Japan Science and Technology Agency (KY), and by JSPS KAKENHI (grant numbers 23390051 (KY) and 25670655 (TKub). The funders had no role in the study design, data collection and analysis, the decision to publish, or preparation of the manuscript.
No competing interests declared.
Notes
- Augustine M, Horwitz MJ. Parathyroid hormone and parathyroid hormone-related protein analogs as therapies for osteoporosis. Curr Osteoporos Rep 2013; 11 (4): 400–6.
- Balsalobre A, Brown SA, Marcacci L, Tronche F, Kellendonk C, Reichardt HM, et al. Resetting of circadian time in peripheral tissues by glucocorticoid signaling. Science 2000; 289 (5488): 2344–7.
- Bunger MK, Walisser JA, Sullivan R, Manley PA, Moran SM, Kalscheur VL, et al. Progressive arthropathy in mice with a targeted disruption of the Mop3/Bmal-1 locus. Genesis 2005; 41 (3): 122–32.
- Chung UI, Schipani E, McMahon AP, Kronenberg HM. Indian hedgehog couples chondrogenesis to osteogenesis in endochondral bone development. J Clin Invest 2001; 107 (3): 295–304.
- Dudek M, Meng QJ. Running on time: the role of circadian clocks in the musculoskeletal system. Biochem J 2014; 463 (1): 1–8.
- el-Hajj Fuleihan G, Klerman EB, Brown EN, Choe Y, Brown EM, Czeisler CA. The parathyroid hormone circadian rhythm is truly endogenous--a general clinical research center study. J Clin Endocrinol Metab 1997; 82 (1): >281–6.
- Field MD, Maywood ES, O’Brien JA, Weaver DR, Reppert SM, Hastings MH. Analysis of clock proteins in mouse SCN demonstrates phylogenetic divergence of the circadian clockwork and resetting mechanisms. Neuron 2000; 25 (2): 437–47.
- Fu L, Patel MS, Bradley A, Wagner EF, Karsenty G. The molecular clock mediates leptin-regulated bone formation. Cell 2005; 122 (5): 803–15.
- Gibbs JE, Beesley S, Plumb J, Singh D, Farrow S, Ray DW, et al. Circadian timing in the lung; a specific role for bronchiolar epithelial cells. Endocrinology 2009; 150 (1): 268–76.
- Gossan N, Zeef L, Hensman J, Hughes A, Bateman JF, Rowley L, et al. The circadian clock in murine chondrocytes regulates genes controlling key aspects of cartilage homeostasis. Arthritis Rheum 2013; 65 (9): 2334–45.
- Gossan N, Boot-Handford R, Meng QJ. Ageing and osteoarthritis: a circadian rhythm connection. Biogerontology 2014. [Epub ahead of print]
- Hinoi E, Ueshima T, Hojo H, Iemata M, Takarada T, Yoneda Y. Up-regulation of per mRNA expression by parathyroid hormone through a protein kinase A-CREB-dependent mechanism in chondrocytes. J Biol Chem 2006; 281 (33): 23632–42.
- Iimura T, Nakane A, Sugiyama M, Sato H, Makino Y, Watanabe T, et al. A fluorescence spotlight on the clockwork development and metabolism of bone. J Bone Miner Metab 2012; 30 (3): 254–69.
- Izumo M, Sato TR, Straume M, Johnson CH. Quantitative analyses of circadian gene expression in mammalian cell cultures. PLoS Comput Biol 2006; 2 (10): e136.
- Jubiz W, Canterbury JM, Reiss E, Tyler FH. Circadian rhythm in serum parathyroid hormone concentration in human subjects: correlation with serum calcium, phosphate, albumin, and growth hormone levels. J Clin Invest 1972; 51 (8): 2040–6.
- Kakar S, Einhorn TA, Vora S, Miara LJ, Hon G, Wigner NA, et al. Enhanced chondrogenesis and Wnt signaling in PTH-treated fractures. J Bone Miner Res 2007; 22 (12): 1903–12.
- Kopic S, Geibel JP. Gastric acid, calcium absorption, and their impact on bone health. Physiol Rev 2013; 93 (1): 189–268.
- Kronenberg HM. Developmental regulation of the growth plate. Nature 2003; 423 (6937): 332–6.
- Lanske B, Karaplis AC, Lee K, Luz A, Vortkamp A, Pirro A, et al. PTH/PTHrP receptor in early development and Indian hedgehog-regulated bone growth. Science 1996; 273 (5275): 663–6.
- Lowrey PL, Takahashi JS. Genetics of circadian rhythms in Mammalian model organisms. Adv Genet 2011; 74: 175-230.
- Michalska D, Luchavova M, Zikan V, Raska I, Jr., Kubena AA, Stepan JJ. Effects of morning vs. evening teriparatide injection on bone mineral density and bone turnover markers in postmenopausal osteoporosis. Osteoporos Int 2012; 23 (12): 2885–91.
- Okubo N, Minami Y, Fujiwara H, Umemura Y, Tsuchiya Y, Shirai T, et al. Prolonged bioluminescence monitoring in mouse ex vivo bone culture revealed persistent circadian rhythms in articular cartilages and growth plates. PLoS One 2013; 8 (11): e78306.
- Potts JTJr, Tregear GW, Keutmann HT, Niall HD, Sauer R, Deftos LJet al. Synthesis of a biologically active N-terminal tetratriacontapeptide of parathyroid hormone. Proc Natl Acad Sci U S A 1971; 68 (1): 63–7.
- Schipani E, Lanske B, Hunzelman J, Luz A, Kovacs CS, Lee K, et al. Targeted expression of constitutively active receptors for parathyroid hormone and parathyroid hormone-related peptide delays endochondral bone formation and rescues mice that lack parathyroid hormone-related peptide. Proc Natl Acad Sci U S A 1997; 94 (25): 13689–94.
- Shearman LP, Sriram S, Weaver DR, Maywood ES, Chaves I, Zheng B, et al. Interacting molecular loops in the mammalian circadian clock. Science 2000; 288 (5468): 1013–9.
- Shigeyoshi Y, Taguchi K, Yamamoto S, Takekida S, Yan L, Tei H, et al. Light-induced resetting of a mammalian circadian clock is associated with rapid induction of the mPer1 transcript. Cell 1997; 91 (7): 1043–53.
- Takarada T, Kodama A, Hotta S, Mieda M, Shimba S, Hinoi E, et al. Clock genes influence gene expression in growth plate and endochondral ossification in mice. J Biol Chem 2012; 287 (43): 36081–95.
- Terazono H, Mutoh T, Yamaguchi S, Kobayashi M, Akiyama M, Udo R, et al. Adrenergic regulation of clock gene expression in mouse liver. Proc Natl Acad Sci U S A 2003; 100 (11): 6795–800.
- Yagita K, Tamanini F, van Der Horst GT, Okamura H. Molecular mechanisms of the biological clock in cultured fibroblasts. Science 2001; 292 (5515): 278–81.
- Yoo SH, Yamazaki S, Lowrey PL, Shimomura K, Ko CH, Buhr ED, et al. PERIOD2::LUCIFERASE real-time reporting of circadian dynamics reveals persistent circadian oscillations in mouse peripheral tissues. Proc Natl Acad Sci U S A 2004; 101 (15): 5339–46.