Abstract
In humans, other mammals, and also in Drosophila, the paternal genome in the sperm is highly condensed and organized mainly in a protamine-based chromatin structure. However, the timing and mechanism of the switch from a histone- to the protamine-based chromatin configuration is still poorly understood. We therefore established Drosophila in vitro cultures of cysts with 64 synchronously developing spermatids genetically marked with histone H2AvD-RFP and ProtamineB-eGFP. Live cell imaging showed that the switch from H2AvD-RFP to Protamine-eGFP chromatin takes approximately five hours, with a short but clear overlap of the presence of both histones and protamines. Moreover, cultured pupal testes showed H4 hyperacetylation at the canoe stage shortly before histone removal; a feature previously observed in the intact animal. We then used TSA to inhibit histone deacetylation and found that premature hyperacetylation was already induced at the round nuclei stage of spermatids. However, this premature hyperacetylation did not lead to a premature switch to the protamine-based chromatin structure, showing that histone hyperacetylation is not the sole inducer of the histone to protamine switch. Importantly, we observed that inactivation of histone acetyltransferases by anacardic acid blocks further differentiation and thus prevents the degradation of histones and the switch to a protamine-based chromatin. Thus, we conclude that H4 hyperacetylation is an essential feature but not the sole inducer of the histone to protamine switch during spermiogenesis.
Abbreviations | ||
APF: | = | after puparium formation |
h: | = | hours |
UTR: | = | untranslated region |
AA: | = | anacardic acid |
TSA: | = | trichostatin A |
INTRODUCTION
It is only once in the life cycle of many vertebrate and invertebrate species - molluscs and Drosophila- that the male genome is not mainly associated with histones in a nucleosomal structure, but in a new, very compact chromatin structure based on protamines. This unique event of the histone to protamine switch is part of the process of chromatin condensation observed during the last steps of spermiogenesis (reviewed in [Ausió Citation1999; Braun Citation2001; Oliva Citation2006]). In mammals, from meiosis onwards, somatic histones are replaced by testis-specific histones. These variant histones are then displaced by the small and basic transition proteins, which, in turn, are replaced by even smaller and more basic protamines [Drabent et al. Citation1996; Lewis et al. Citation2003; Meistrich et al. Citation2003; Yan et al. Citation2003; Govin et al. Citation2004; Zhao et al. Citation2004; Kimmins and Sassone-Corsi Citation2005]. Binding of protamines to the DNA results in a doughnut-like structure many times more compact than the nucleosomal chromatin [Ward Citation1993; Brewer et al. Citation1999]. Hence, not surprisingly, protamines are known to be essential for the formation of fertile, male gametes in mammals [Cho et al. Citation2001; Citation2003; Steger et al. Citation2003; Aoki et al. Citation2005; Torregrosa et al. Citation2006]. It has been proposed that the compact structure of protamine-based chromatin is protecting the genome from mutations [Braun Citation2001; Oliva Citation2006]. This hypothesis is supported by the recent finding that lack of protamines in Drosophila leads to a 20 fold increase of sensitivity for X-radiation [Rathke et al. Citation2010].
The molecular mechanism of the histone to protamine switch is not fully understood, although many characteristic features have been described in a number of model organisms and man (reviewed in [Braun Citation2001; Sassone-Corsi Citation2002; Rousseaux et al. Citation2005; Carrell et al. Citation2007]). For example, the histone to protamine switch in mammalian spermatids is accompanied by histone H4 hyperacetylation just prior to histone detachment and the occurrence of DNA breaks during the actual transition stages [Hazzouri et al. Citation2000; Laberge and Boissonneault Citation2005; Leduc et al. Citation2008; Hecht et al. Citation2009]. The acetylation of H4 was proposed to be a prerequisite for histone removal and a possible starting signal for the onset of the histone to protamine replacement process [Braun Citation2001; Sassone-Corsi Citation2002]. In accord with this hypothesis, impaired spermatogenesis in man and mouse has been correlated with a lack of histone H4 hyperacetylation [Sonnack et al. Citation2002; Fenic et al. Citation2004]. Additionally, the acetylation of histone H3K9 was described to increase simultaneously with H4 hyperacetylation in mouse and, to be involved in histone detachment [Hazzouri et al. Citation2000; Nair et al. Citation2008].
In the experimentally well accessible model organism Drosophila, sperm nuclei are characterized by their slim shape and highly condensed chromatin (for review see [Fuller Citation1993; Renkawitz-Pohl et al. Citation2005]). At the molecular level, Drosophila testis-specific linker histone-like proteins are known to be present in postmeiotic stages [Santel et al. Citation1997; Hempel et al. Citation2006] in resemblance to the mammals where somatic histones are exchanged for testis-specific variants. The linker histone variants together with the core histones are then removed from the spermatid chromatin to be replaced by small and basic proteins. These proteins form the major chromatin component in mature sperm and clearly represent the protamines of Drosophila [Jayaramaiah‐Raja and Renkawitz-Pohl Citation2005]. Interestingly, as in mammals, the histone to protamine switch in Drosophila is also first marked by an increase in H4 acetylation and then by the introduction of DNA breaks. This is followed by the transient presence of a possible functional homologue of transition proteins (Tpl94D) and ends with the deposition of protamines on the DNA. Although, these typical features of the transition in chromatin structure are known to take place mainly during the canoe stage [Rathke et al. Citation2007] it is not known how long this transition takes. Therefore, one of our aims was to determine the timing of the histone to protamine switch in Drosophila spermatids.
The aforementioned striking parallels between mammalian and Drosophila spermiogenesis prompted us to further explore the role played by histone acetylation in the histone to protamine switch mechanism of Drosophila. To this end, we established a double-transgenic fly line expressing H2AvD-RFP (a kind gift from C. Wenzl – unpublished; construct based on the H2AvD-GFP reported by [Clarkson and Saint Citation1999]) and ProtamineB-eGFP [Jayaramaiah‐Raja and Renkawitz-Pohl Citation2005] under the control of their natural promoters and 5′UTR for appropriate translational control of the protamineB-eGFP mRNA. Since in Drosophila, 64 postmeiotic spermatids develop in independent units, called cysts, we were able to culture isolated developing spermatid cysts and to determine the timing of the histone to protamine switch by in vivo imaging. Furthermore, we addressed the role of H4 hyperacetylation in the histone to protamine switch using a set of inhibitors in pupal testes in culture. These studies show that induction of H4 hyperacetylation at an early stage of spermiogenesis is not sufficient to induce a premature onset of the histone to protamine switch. However, upon inhibition of H4 acetylation, no histone to protamine exchange was observed. Clearly, H4 hyperacetylation is essential to allow further development of the haploid male germ cell.
RESULTS
Isolated Cysts of Germ Cells Develop in Culture Through Meiosis and the Histone to Protamine Switch
The analysis of the morphological changes occurring in sperm development, including chromatin condensation and nuclear reshaping, has lead to the description of distinct developmental stages in vertebrates and Drosophila (for review see [Holstein et al. Citation2003; Fuller Citation1993]). Drosophila provides an excellent model system in that spermatogonia derived from one asymmetric stem cell division develop as one unit encased by two somatic cells. These independent units are called cysts (reviewed by [Fuller Citation1993]). The germ cells in one cyst synchronously pass through mitotic amplification, meiosis and finally, spermiogenesis before they are released as mature, individualized sperm. In , the relevant stages of spermatogenesis are depicted schematically from the spermatocyte stage onwards to elongated spermatids. After meiosis, the nebenkern stage is characterized by round nuclei flanked by the nebenkern, an aggregation of mitochondria (). During the early elongation phases of the flagella, the nuclei are still round but then start to elongate () and enter the canoe stage ( and for the early and late canoe stage). We aimed to follow chromatin reorganization in an individual cyst with 64 synchronously developing spermatids during this reshaping. Therefore, we manually isolated spermatid cysts from flies expressing ProtamineB-eGFP and H2AvD-RFP, the latter of which is a histone variant representing core histone distribution [Rathke et al. Citation2007]. Isolated cysts of the premeiotic primary spermatocyte stage () passed through meiosis and develop further until the elongating nuclei stage, which we frequently observed at approximately 30 h after the meiotic divisions (data not shown). Unfortunately, culture conditions deteriorate and lethality increases after three days and therefore, primary spermatocytes were not observed developing beyond the elongating nuclei stage. However, cysts isolated and cultured at the round nuclei stage () developed into the elongating nuclei stage and then continue until after the histone to protamine switch between the early and late canoe stages (). , therefore, shows part of a time-lapse series obtained from a primary spermatocyte stage cyst () and the complete series of a cyst cultured at the round nuclei stage (). The temporal resolution of this virtual time-lapse recording is one image per 10 h beginning with meiotic divisions. Remarkably, the early canoe stage of spermatid nuclei appeared to be rather extended (20 h, ) when compared to earlier stages.
FIGURE 1 Germ cells in isolated cysts pass through meiosis and histone to protamine transition in culture. Time-lapse series of fluorescence micrographs of two independent isolated cysts developing in culture. Cysts of primary spermatocyte (A) and round nuclei stage (C) were isolated from testes of flies containing H2AvD-RFP and ProtamineB-eGFP fusion genes and their development was followed in culture (25°C). Part of the resulting time-lapse series of a primary spermatocyte cyst (A, B) is continued by the series of a cyst cultured at the round nuclei stage (C–G). The temporal resolution of this virtual time-lapse is one image per 10 h starting at meiotic division. The drawings next to the fluorescence micrographs represent the stage of the cysts as seen in phase contrast. At the nebenkern stage (B, ca. 10 h after division), the spermatid nuclei (n) are flanked by the nebenkern (nk) and the spermatids are surrounded by two somatic cyst cells (cy). At the round nuclei stage (C, ca. 20 h after division), germ cell nuclei are visible as a cluster and the nebenkern has elongated along the growing axonem of the flagella (fl). At the elongated nuclei stage (D, ca. 30 h after division), the nuclei are ellipsoid and the flagella have grown. At the canoe stage (E–G), nuclear shaping continues in parallel to chromatin reorganization. The early canoe stage, characterized by histone-based chromatin with H2AvD-RFP, appears to be extended in duration and is recognized at 40 and 50 h after division. Cysts cultured at the round nuclei stage complete the histone to protamine transition (F–G) and the late canoe stage chromatin is characterized by ProtamineB-eGFP (G). Scale bar: 50 μm.
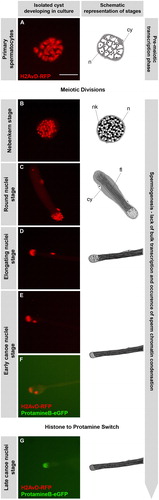
We note that in the isolated cysts, histone H2AvD-RFP persisted until the early canoe stage, while the late canoe stage nuclei contain protamine-based chromatin (). This development reflects the in situ situation in the intact fly [Jayaramaiah-Raja and Renkawitz-Pohl Citation2005] and is representative for all histones in Drosophila spermatids [Rathke et al. Citation2007]. These experiments clearly show that germ cell nuclei in cultured cysts complete the transition from histones to protamines in the normal pattern. Consequently, we applied this cyst culture system to analyze the timing of the histone to protamine switch by imaging cysts throughout the canoe stage at a higher temporal resolution.
Within Five Hours the Spermatid Chromatin Switches from a Histone- to a Protamine-based Structure
We dissected testes from histone H2AvD-RFP and ProtamineB-eGFP expressing transgenic flies and manually isolated and cultured those cysts that did not yet express protamines, but were already in the early canoe stage. To determine the time frame necessary for the completion of the histone to protamine switch in Drosophila spermatids, we followed numerous individual cysts in culture by time-lapse fluorescence microscopy, and recorded early canoe stage cysts developing through the histone to protamine switch with a temporal resolution of one image per hour ().
FIGURE 2 Time frame of the histone to protamine switch in nuclei of cultured spermatid cysts. Time-lapse series of phase contrast and fluorescence microscopic images of the tip of a spermatid cyst cultured in vitro. Spermatid cysts were isolated from testes of transgenic flies containing H2AvD-RFP and ProtamineB-eGFP fusion genes. At the start of the series, spermatid nuclei (arrows) show a clear H2AvD-RFP signal, whereas no ProtamineB-eGFP expression is detected (A). After 5 to 6 h of incubation (25°C), the histone signal has disappeared and the ProtamineB-eGFP signal is at a higher intensity (F–G). During the switch process, at 2 to 3 h, a short overlap of histone and protamine expression occurs, albeit with low fluorescence intensity of both marker proteins (C–D). Scale bar: 15 μm.
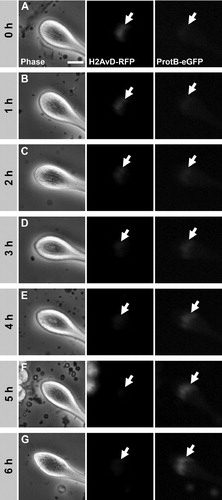
At the start of the series, the nuclei gave a clear H2AvD-RFP signal whereas no ProtamineB-eGFP signal was observed (, arrows). Consistent with our impression of an extended early canoe stage, many cysts were imaged without significant changes for several hours before entry into the histone to protamine transition. With the onset of the exchange process the histone signal disappeared gradually (, arrows) and had vanished after around 5 to 6 h (, arrows). In the same manner, the protamine signal became stronger and was clearly visible at the end of the transition process (). Interestingly, we observed a slight overlap of both signals exhibiting low fluorescence intensity (). Thus, we concluded that it takes about 5 h at 25°C to switch the chromatin structure of Drosophila spermatids from a histone- to a protamine-based configuration.
Intact, Cultured Pupal Testes are a Valuable Test System to Study the Histone to Protamine Switch by Means of Inhibitor Treatment
The histone to protamine switch is accompanied by histone hyperacetylation and DNA breaks along with their repair. For a mechanistic analysis of these processes, loss-of-function mutations of, for example, histone acetyltransferases seem not to be suitable, as they often confer embryonic or late larval lethality. Thus, we aimed to establish a testis culture system to investigate the role of histone acetyltransferases during spermiogenesis by means of suitable inhibitors.
Within testes of adult flies, numerous cysts are present that are at different stages of nuclear reorganization, and thus, it is hardly possible to follow the development of individual germ cells within a testis. However, the first meiotic division is known to occur during puparium formation (reviewed in [Renkawitz-Pohl et al. Citation2005]). Therefore, we tested at what time point in pupal testes the first cysts show a protamine-based configuration and found cysts that contain ProtamineB-eGFP at around 48 h after puparium formation (APF) (data not shown). In comparison, we observed that pupal testes at 24 h APF contain all premeiotic and postmeiotic stages up to the early canoe stage before the histone to protamine switch. Thus, first cohorts of spermatids switch to a protamine-based chromatin between 24 and 48 h APF.
It is of advantage that the gonads at 24 h APF (still pear shaped) are not yet connected to the somatic parts of the male reproductive system, such as the paragonia and vas deferens, which originate from the genital imaginal disc [Bodenstein Citation1950]. Consequently, these testes, which still lack ProtamineB-eGFP positive cysts, can be removed from the abdomen of the pupae and cultured. As expected, we found that the histone to protamine switch takes place in the cultured pupal testes after 24 h of incubation as within the intact fly (Fig. 4A and A′).
Conveniently, the testes of pupae at 24 h APF still lack pigmentation and the sheath layers are still quite thin [Bodenstein Citation1950] and, therefore, germ cells should be better accessible to inhibitors than in testes of adult flies. Furthermore, it is possible to analyze the development of germ cells after treatment for many hours spanning the in vivo time frame of the histone to protamine switch and longer. Thus, we concluded that these organ cultures of early pupal testes are suitable to study the histone to protamine switch mechanism using inhibitors. In these studies, the inhibitors of interest were added to the culture of early pupal testes andthe occurrence of ProtamineB-eGFP expressing post-switch cysts monitored.
The Histone Acetyltransferase Inhibitor Anacardic Acid Does Not Induce Apoptosis Nor is it Cytotoxic Since Post-Switch Cysts Develop Normally
The establishment of early pupal testes cultures enables us to investigate the significance of histone acetylation for the removal of histones from the spermatid nuclei using inhibitors of histone acetyltransferases (HATs) and histone deacetylases (HDACs) at retested appropriate concentrations (data not shown).
Anacardic acid (AA) and garcinol are known inhibitors of HATs from several families [Balasubramanyam et al. Citation2003; Citation2004; Varier et al. Citation2004; Sun et al. Citation2006]. However, numerous inhibitor chemicals and compounds are known to often result in cytotoxic effects and/or the induction of apoptosis in cell and organ cultures. Thus, we addressed the cytotoxicity of anacardic acid and garcinol () and tested whether cells without histones are resistant to the effects of these drugs by monitoring their ability to differentiate.
FIGURE 3 Cytotoxicity of anacardic acid (AA) in spermatogenic cells is low compared to garcinol and AA does not induce apoptosis. (A) Pupal testes showing a single ProtamineB-eGFP positive cyst were incubated for 11 h without inhibitor (A, a and a′) or with 150 μM AA (A, b and b′) and 50 μM garcinol (A, c and c′). The number of ProtB-eGFP expressing cysts (arrows) increases in control testes after 11 h of culture (A, a′). Such an increase is also observed in AA-treated testes (A, b′), whereas treatment with garcinol blocks further development of post-switch cysts (A, c′). (B) Out of 23 pupal testes in the control culture, 95.7% developed further ProtB-eGFP positive cysts after 11 h of culture as did 73.9% of 23 AA-treated testes. However, further development was observed in only 13.6% of 22 garcinol-treated testes. (C) Squash preparations of nuclei from pupal testes cultured either without inhibitor (C, a and b) or with 150 μM AA (C, c and d). DNA was stained with Hoechst (C, a, b, c, and d) and DNA breaks were visualized by the TUNEL assay (a′, b′, c′, and d′). Primary spermatocytes from AA-treated testes show chromatin distortion (C, c compare to a), but no increase in TUNEL signal (c′), indicating that apoptotic DNA degradation is not involved in this effect. The TUNEL signal in AA-treated early canoe stage nuclei (d′) is not above control level (b′).
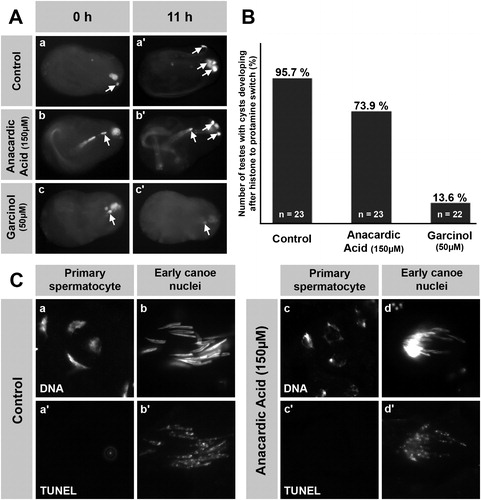
We isolated pupal testes already having one protamine positive cyst as this is an indication that several other cysts are in the process of histone degradation and protamine incorporation. Indeed, in both control pupal testes (no inhibitor) and pupal testes treated with AA (150 μM), we observed an increased number of post-switch cysts after 11 h of treatment (, a, a′, b, b′, arrows). In contrast, treatment with the HAT inhibitor garcinol (50 μM) did not allow further development, indicating a cytotoxic side effect (, c, c′, arrows). More specifically, 73.9% of 23 anacardic acid-treated testes showed increased numbers of protamine positive cysts, compared to 95.7% of the 23 control testes and only 13.6% of the 22 garcinol-treated testes (). Clearly, garcinol strongly reduces the viability of cells even after the onset of histone degradation, whereas anacardic acid reduces cell viability only slightly.
To rule out the possibility that anacardic acid might induce apoptosis and apoptotic DNA degradation, we examined DNA breaks using the TUNEL assay in spermatid nuclei of AA-treated testes (). In primary spermatocytes, DNA breaks were not detected in control testes (, a, a′) and also not detected in AA- (150 μM) treated testes (, c, c′), despite the chromatin distorting effect of AA (, c). However, it should be borne in mind that DNA breaks are a typical feature accompanying the transition from a histone- to protamine-based chromatin structure. Therefore, we checked whether the TUNEL signal increased at the canoe stage after treatment with AA. We found that in the early canoe stage nuclei, the signal was not increased above in vivo levels despite AA treatment (, b′, d′) and concluded that the AA-treated nuclei show no obvious apoptotic DNA degradation. Thus, cultured pupal testes and anacardic acid are suitable to study the functional significance of histone hyperacetylation for the histone to protamine switch in Drosophila spermiogenesis.
Treatment with Anacardic Acid Blocks the Histone to Protamine Switch in Intact Pupal Testes, Whereas Treatment with TSA has No Such Blocking Effect
Histone hyperacetylation occurring prior to the histone to protamine switch has been proposed to be a prerequisite as well as a possible starting signal for histone detachment [Braun Citation2001]. To test this hypothesis in the Drosophila background, we isolated intact pupal testes at 24 h APF before entry into the histone to protamine transition. We then subjected these testes in culture to 150 μM anacardic acid for about 24 h, in order to block histone acetylation (for details and number of testes analyzed see Materials and Methods section).
In sharp contrast to the untreated control (, A′), in AA-treated testes, no ProtamineB-eGFP expressing, post-switch cysts were detected after 24 h of incubation (, B′). ProtB-eGFP was not detected in several independent experiments with a total of 44 testes. Only 5 testes showed cysts with ProtB-eGFP expression and, most likely, these cysts already had entered the switch when AA was added. This observation strongly implies that hyperacetylation of histones is a prerequisite for Drosophila spermatids to develop from a histone-based to a protamine-based chromatin structure.
FIGURE 4 Anacardic acid (AA), but not trichostatin A (TSA), inhibits the histone to protamine switch in cultured, intact pupal testes. (A-D′) Pupal testes (24 h after puparium formation) of ProtamineB-eGFP expressing flies were incubated for 24 h in medium without inhibitors (A, A′) and medium supplemented with either 150 μM AA (B, B′), 5 μM TSA (C, C′), or 50 μM TSA (D, D′). No ProtamineB-eGFP expressing cysts can be observed at the beginning of the culture (A-D). After 24 h of incubation, some cysts have undergone the histone to protamine switch and ProtamineB-eGFP positive cysts (arrows) can be observed in control and TSA-treated testes (A′, C′, D′). However, testes treated with AA do not develop ProtamineB-eGFP positive cysts (B′). (E-F′) Low magnification overviews of squash preparations of untreated (E, E′) and AA-treated (F, F′) pupal testes. Expression of Tpl94D-eGFP is no longer detectable after treatment with AA (F, F′, arrows indicate nuclei of the latest observable stage). Arrowheads in A-D mark auto-fluorescence of putative fat body cells. Scale bar: A-D′ 100 μM; E-F′ 100 μM.
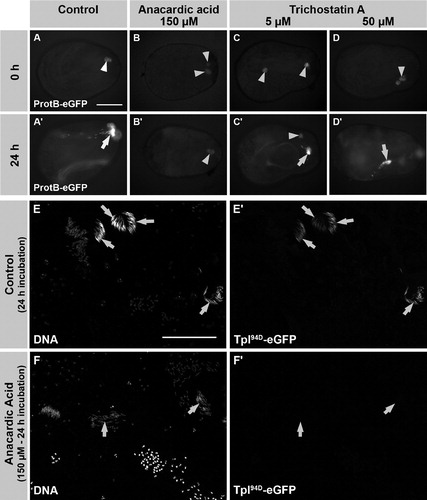
We next addressed whether blocking of HDACs by trichostatin A (TSA) influences the development of spermatids. Interestingly, we observed that in testes treated with 5 μM and 50 μM TSA, protamine-containing post-switch spermatids develop (, C′, and D, D′). Thus, inhibition of histone deacetylation did not block the switch from a histone to protamine based chromatin. However, we cannot exclude that the timing of this transition process was affected. The inhibition of HATs by anacardic acid should result in a lack of acetylated histones. Consequently, these results provide the first evidence that acetylation but not deacetylation of histones is essential for spermiogenesis in Drosophila testes.
Anacardic Acid Blocks H4 Acetylation and Induces Chromatin Compaction in the Absence of Tpl94D-eGFP and ProtamineB-eGFP Expression
To better inspect the spermatid nuclei after inhibitor treatment, we used squash preparations of cultured testes and assessed the level of histone acetylation by immunofluorescence ( and ). In control testes, acetylated histone H4 could be detected in primary spermatocytes, but this was reduced to a very low level in round nuclei stage after meiosis and then reappeared in elongating and early canoe nuclei (, row C). Moreover, we observed histone H2AvD-RFP as well as H4 hyperacetylation until the early canoe stage with protamines being expressed from the late canoe stage onwards (, rows B, C, and D). Acetylation of histone H3 (H3K9) could be clearly detected in primary spermatocytes, while in postmeiotic stages, H3K9 acetylation did not increase as it was hardly detectable and present in a constitutive level (, row F). Histone acetylation disappeared in correlation with histone degradation.
FIGURE 5 Histone H4 hyperacetylation occurs in the late elongating nuclei stage after meiosis, while histone H3K9 acetylation is found only at basic levels postmeiotically. Squash preparations of spermatid nuclei from adult testes. DNA is stained with Hoechst (rows A and E). Histone expression is represented by H2AvD-RFP fusion protein (row B) and shows loss of histones after the early canoe stage (columns 4–5). Protamine incorporation is visualized by the ProtamineB-eGFP fusion protein at the late canoe stage (row D, column 5). Histone H4 acetylation is marked with an antibody recognizing acetylated lysine 5, 8, 12, and 16 (row C). H4 acetylation is reduced to basic levels at the round nuclei stage (row C, column 2) after meiosis and then reappears in the late elongating nuclei stage and persists until histone degradation (row C, columns 3 and 4). H3K9 acetylation is found only at very low basic levels after meiosis (row F; tested with two independent antibodies). Scale bar: 10 μM.
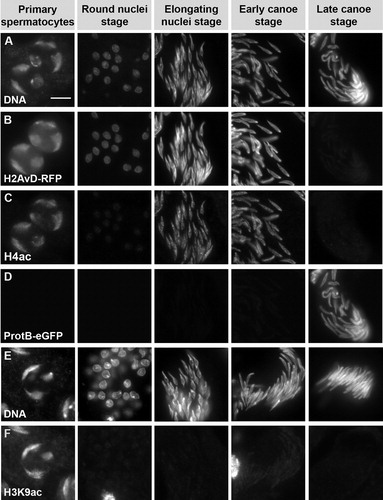
FIGURE 6 Anacardic acid (AA) and trichostatin A (TSA) affect acetylation of histone H4 and spermatid chromatin structure. Squash preparations of spermatid nuclei from pupal testes incubated with 150 μM AA (rows D-F) and 50 μM TSA (rows G and H) in comparison to untreated testes (rows A-C). DNA is stained with Hoechst (rows A, D, and G). H4 acetylation (rows B, E, and H) and H4R3-dimethylation (rows C and F) are detected by immunofluorescence. Treatment with AA leads to a complete reduction of H4 acetylation signal in all spermatid stages (row E), while other modifications like H4R3-dimethylation are not affected (compare rows C and F). In AA-treated samples the chromatin is strongly compacted in comparison to control samples (rows A and D). Although a spermatocyte nucleus is spread over a larger area after AA treatment (row D, column 1), the three large chromosome domains still appear to be strongly compacted in comparison to the untreated nuclei (row A, column 1). The AA-induced compaction is easily observed in the postmeiotic stages. Treatment with TSA leads to a decondensed chromatin structure, as is visible in premeiotic spermatocytes (row G, column 1). TSA also induces increased H4 acetylation in primary spermatocytes (row H, column 1) as well as the round nuclei stage (row H, column 2) after meiosis. In later stages, this effect seems to fade and is not detectable any longer (row H, columns 3 and 4). Scale bar: 10 μM.
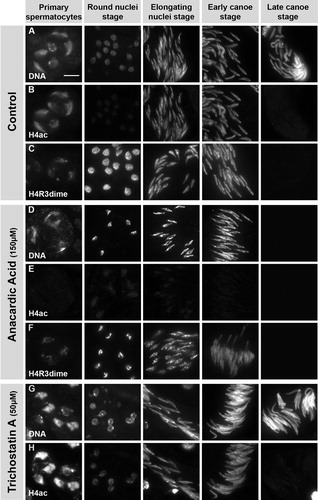
In contrast to the control testes, no H4 acetylation signal was detected in spermatid nuclei of anacardic acid- (150 μM) treated testes (, row E). The characteristic pattern of histone H4R3-dimethylation [Rathke et al. Citation2007], however, was clearly detectable and appeared unchanged (, rows C and F). This shows the persistence of histone H4 and the inhibition of its acetylation by anacardic acid. Furthermore, the DNA of AA-treated nuclei appeared to be strongly condensed in comparison to the control. This condensation was easily discernible at the round and elongated nuclei stage (, rows A and D). In agreement with our data from the intact cultured pupal testes (in shown for ProtamineB-eGFP), neither the putative transition protein Tpl94D-eGFP (, F′) nor ProtamineB-eGFP was observed in spermatid nuclei after AA treatment (data not shown). From these data, it was clearly evident that histone hyperacetylation is an essential feature to allow the development of Drosophila spermatids from a histone to protamine based chromatin structure.
TSA Induces Premature H4 Acetylation at the Round Nuclei Stage in Postmeiotic Spermatids
The inhibitory effect of trichostatin A (TSA) on both class I and II HDACs is well known [Yoshida et al. Citation1990; Mai et al. Citation2005]. However, since treatment of intact pupal testes with 5 μM and 50 μM TSA had no obvious effect on the histone to protamine switch (, C′ and D′), we analyzed the acetylation status of spermatid histones by immunofluorescence in squash preparations of TSA-treated testes (, rows G and H).
Inhibition of HDACs by TSA should increase acetylation in all developmental stages requiring HDAC activity. Indeed, the H4 acetylation signal was greatly increased and the chromatin appeared to be more decondensed in primary spermatocytes from testes treated with 50 μM TSA (, row G, column 1). Interestingly, in round nuclei of these testes, a clear H4 acetylation signal was detected that was not present in control testes (, rows B and H, column 2). Hence, this signal provided evidence that the level of histone H4 acetylation is prematurely increased in an early postmeiotic spermatid stage. Surprisingly, the effect of TSA was not as pronounced in elongating and early canoe stage nuclei (, row H, columns 3 and 4). This observation may indicate a possible reduction in the amount of HDAC present in these late postmeiotic stages.
TSA-Induced Premature H4 Acetylation Does Not Lead to Premature Signs of Histone to Protamine Switch
We observed that TSA induces premature H4 hyperacetylation at the round nuclei stage in postmeiotic spermatid nuclei (, row H, column 2). According to the assumption that increasing histone H4 acetylation plays a significant role as the starting signal for the histone to protamine switch, we should expect premature induction of histone degradation, DNA breaks and Protamine-eGFP translation. To test this hypothesis, we subjected squash preparations of TSA-treated testes to the TUNEL assay that specifically labels DNA single- and double-strand breaks in spermatid nuclei (). However, we did not detect an increase in the TUNEL signal at the round nuclei stage of TSA (5 μM and 50 μM) treated testes (, row c). Moreover, the pattern and intensity of the TUNEL signal was not altered in TSA-treated testes compared to the control (, row c). In contrast, the effects of TSA (increased H4 acetylation and decondensed chromatin) could be observed already at low concentrations (5 μM, , row b). At a higher concentration chromatin decondensation in primary spermatocytes became more obvious (50 μM, , row b).
FIGURE 7 Trichostatin A- (TSA) induced, premature H4 acetylation does not induce premature DNA breaks. Squash preparations of nuclei from pupal testes cultured without inhibitor (A) or with 5 μM TSA (B) or 50 μM TSA (C). DNA was stained with Hoechst (row a). H4 acetylation was detected by antibody (row b) and DNA breaks were detected with a TUNEL assay (row c). Treatment of pupal testes with the HDAC inhibitor TSA leads to an increase in H4 acetylation signal at the round nuclei stages (B-C, row b, column 2) that is not seen in control testes (A, row b, column 2). However, this is not accompanied by a significant increase of TUNEL signal (B-C, row c), indicating no premature induction of DNA breaks after TSA treatment. Scale bar: 10 μM.
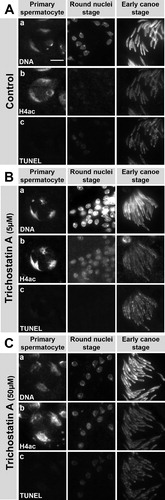
We next isolated cysts of round nuclei stage from flies expressing H2AvD-RFP and ProtamineB-eGFP. They were then incubated with either 5 μM or 50 μM TSA or without inhibitor for ca. 20 h ( and ). Only the shape and growth of the two cyst cells encasing the developing spermatid bundle appeared to be affected by TSA (, arrow). However, TSA-treated spermatids developed further and formed elongated nuclei and extended flagella (, arrowheads). Most of the cysts treated with 50 μM TSA (7/13) developed further like nearly all of the cysts treated with 5 μM TSA (13/14) or cysts that remained untreated (17/18). This indicates that spermatid development that leads to the next stage is not impaired by TSA treatment and also that no premature histone degradation is induced since the H2AvD-RFP signal was still clearly visible. Finally, we did not detect premature translation of repressed and stored protamineB-eGFP transcript (data not shown).
FIGURE 8 Anacardic acid (AA) blocks development of cultured, isolated cysts, whereas trichostatin A (TSA) treatment still allows progression of round nuclei stage spermatids to elongated stages. Fluorescence micrographs of isolated spermatid cysts in culture. The cyst shape is outlined with dashed lines. Round nuclei stage cysts were isolated from H2AvD-RFP expressing pupal testes and incubated without inhibitor (A) or with 150 μM AA (B), 5 μM TSA (C), or 50 μM TSA (D) for about 20 h. While control cysts develop from round nuclei (arrowhead) into the late elongated nuclei stage, this is not the case after AA treatment (B). AA blocks further development and nuclei become compacted and tiny (arrowhead). After TSA treatment (C, D), spermatid nuclei continue to develop into elongated nuclei stages (arrowheads), albeit TSA appears to influence cyst cell shape and growth (large arrow in D).
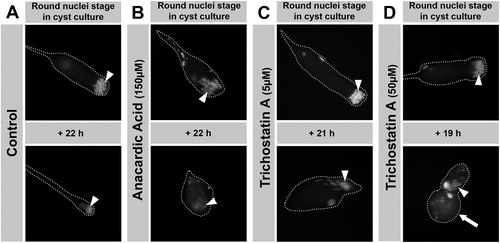
In contrast to TSA, anacardic acid treatment of isolated round nuclei stage cysts prevented further differentiation (0/13 developing cysts) (). Again, the chromatin condensing effect of AA was seen as already observed in squash preparations of treated testes (compare , row D). Not surprisingly, premeiotic, transcriptionally highly active cysts at the primary spermatocyte stage were not able to complete meiosis after incubation with either AA or TSA (data not shown). In summary, the increased level of histone H4 acetylation, prematurely induced by TSA, did not result in a premature onset of the histone to protamine switch as indicated by the absence of its characteristic hallmarks, namely histone degradation and occurrence of DNA breaks.
DISCUSSION
During spermiogenesis in many organisms, the spermatids switch from a histone to a protamine based chromatin structure. However, the timing and mechanism of this reorganization is only poorly understood. One common feature across-species is the accumulation of histone H4 acetylation prior to histone degragation as has been shown, for example, in humans [Hecht et al. Citation2009], mouse [Hazzouri et al. Citation2000], rats [Meistrich et al. Citation1992], fish [Christensen et al. Citation1984], the mollusc Sepia [Kurtz et al. Citation2007], and Drosophila melanogaster [Rathke et al. Citation2007]. Here, we used spermatid cyst cultures and organ cultures to study the timing of the histone to protamine switch and to elucidate the functional significance of H4 hyperacetylation for developing spermatids in Drosophila.
Isolated Spermatid Cysts Pass Through Meiosis and Complete the Histone to Protamine Switch within Five Hours in Culture
For many years, Drosophila spermatid cysts have been known to be able to develop outside of the testis in the appropriate culture media (e.g. [Kuroda Citation1974]). This culture technique was then used to follow the timely progression of Drosophila spermatid stages in various culture conditions and to analyze a range of mechanisms in detail, e.g. sperm individualization [Cross and Shellenbarger Citation1979; Noguchi and Miller Citation2003; Kawamoto et al. Citation2008].
In this study, we applied time-lapse imaging to follow the development of cultured, individual spermatid cysts isolated from a transgenic fly line expressing H2AvD-RFP and ProtamineB-eGFP. We were able to document that postmeiotic spermatids, cultured at the round nuclei stage, successfully pass through the canoe stage including the switch from histones to protamines between the early and late canoe stages. From these cultures, we gained the impression that the duration of the early canoe stage is extended (more than 20 h) in comparison to earlier stages. Hence, we propose that this phase prepares the release of histones from the DNA and their subsequent degradation. In parallel, perinuclear microtubules are rearranged; a feature required for nuclear shaping (for review see [Fuller Citation1993]). In vivo, it takes 96 h to develop from the 16 cell spermatocyte stage to mature spermatids [Lindsley and Lifschytz Citation1972; Tokuyasu Citation1975]. The same development was recently reported to take only 48 h in cultures complemented with fly extract [Kawamoto et al. Citation2008]. However, in our cyst cultures, the timing of germ cell development corresponds well to that of the situation in vivo. Here, the expression of Protamine-eGFP serves as a useful marker for the late stages of spermiogenesis, since other signs, like coiling and the appearance of cystic bulges (individualization), frequently occur prematurely in culture.
Making use of a double transgenic fly strain expressing histone H2AvD-RFP and ProtamineB-eGFP, we observed the complete switch of histones to protamines within a short time of approximately 5 h at 25°C in isolated spermatid cysts in culture. Given the extended early canoe stage duration, this is a short time frame for such a chromatin reorganization. To allow the rapid degradation of histones, it is very likely that the histones are degraded directly in the nucleus by testis-specific proteasomes that were shown to be located in the nucleus as well as to be involved in spermiogenesis [Zhong and Belote Citation2007].
In the time-lapse recordings, we observed a short overlap of 1 to 2 h between histones and protamines with both markers being visible at low fluorescence intensity. We did not recognize this overlap phase in earlier studies with squash preparations of adult testes, probably due to the short duration of the overlap and the faint fluorescence of both markers.
During this histone and protamine overlap phase detected in Drosophila spermatid proteins like Tpl94D are present [Rathke et al. Citation2007]. These proteins might be characteristic for the chromatin at the transition stage similar to the situation in mammals where transition proteins (TP1 and TP2) bridge the switch from histones to protamines (reviewed by [Braun Citation2001]).
To date, neither fluorescent fusion proteins nor antibodies against histones produced significant signal in squash preparations of Drosophila spermatids after the histone to protamine switch. This may indicate a complete degradation of histones in Drosophila spermatogenesis. However, we cannot exclude the existence of residual pockets of histone bound chromatin possibly fulfilling similar functions to those recently proposed for residual histones in mammalian sperm [Arpanahi et. al. Citation2009; Hammoud et. al. Citation2009].
In Drosophila, Histone Deacetylation is Not Essential for the Histone to Protamine Switch
We performed inhibitor studies on cultured pupal testes and isolated spermatid cysts of Drosophila. The protamine-eGFP transgene allowed us to follow the consequences of HDAC inhibition on chromatin reorganization over many hours in vivo. The inhibition of HDACs by TSA in cultures of pupal testes did not block the occurrence of the histone to protamine switch. In addition, late pre-switch stages of developing spermatids were unaffected by the inhibitor and did not show increased H4 acetylation. This indicates that histone deacetylase activity is dispensable in these stages and for the progression of the switch mechanism. However, at the round nuclei stage, H4 acetylation was clearly increased by TSA treatment. We therefore propose that in Drosophila spermatids after meiosis, the HDAC concentration could be reduced gradually towards the stages of nuclear elongation. This scenario was previously shown to be the case after meiosis in round spermatids of mice [Hazzouri et al. Citation2000].
Histone Hyperacetylation is Not Sufficient to Induce the Histone to Protamine Switch
Here we show that the histone to protamine switch is not impaired in intact pupal testes of Drosophila cultured for 24 h with TSA. In addition, we clearly observed increased levels of H4 hyperacetylation already in early postmeiotic spermatids, constituting premature histone hyperacetylation. In cultures of isolated cysts at the round nuclei stage incubated for 20 h in the presence of TSA, we could follow the development of an individual bundle of spermatids into the next stage characterized by elongated nuclei. We did not however detect premature histone degradation or the translation of protamine transcripts after TSA treatment of cultured cysts. Hence, it appears that spermatids develop normally despite premature histone hyperacetylation. Also in mouse, incubation of spermatogenic cells with TSA for 3 h was observed to lead to increased H4 acetylation levels in early postmeiotic spermatids. However, longer-term development of the germ cells was not investigated in these cultures [Hazzouri et al. Citation2000]. TSA-induced premature hyperacetylation in mouse spermatids seems to be accompanied by premature DNA breaks after 4 h of incubation [Laberge and Boissonneault Citation2005]. Consequently, we wanted to know whether this association between histone acetylation and DNA breaks was also true for Drosophila spermiogenesis. However, we did not detect significantly increased levels of DNA breaks in spermatid nuclei of TSA-treated testes using the TUNEL assay. We consider it very possible, that a connection between increased H4 acetylation levels and the occurrence of DNA breaks does not exist in Drosophila spermatids. While in mammals, the best supported candidate for the DNA nick introducing agent is Topoisomerase II [Leduc et al. Citation2008], this is still not known in Drosophila and involvement of nucleases is equally possible. Hence, independent pathways for histone acetylation on one hand and induction of DNA breaks on the other converging in the histone to protamine switch are possible as was already proposed for other features of Drosophila spermiogenesis [Fuller Citation1993]. We conclude that TSA-induced histone acetylation is not sufficient to act as the sole starting signal triggering the histone to protamine switch since we did not detect induction of histone degradation or increased DNA breaks.
Histone Acetylation Plays an Essential Role in Drosophila Spermiogenesis
During mouse spermiogenesis, it was shown that next to histone H4 hyperacetylation, histone H3 acetylation also increases [Hazzouri et al. Citation2000]. This increase may argue for a general role of histone acetylation in facilitating the release of histones since reduced acetylation of H3 at lysine 9 leads to increased retention of H3 in spermatid nuclei [Nair et al. Citation2008]. We tested hyperacetylation of histone H3 in Drosophila with two independent antibodies against histone H3 lysine 9 acetylation and found no postmeiotic increase. Nevertheless, after the histone to protamine switch, all histones, including H3, are degraded and hence, could not be detected by immunofluorescence in Drosophila spermatids any longer [Rathke et al. Citation2007]. This finding possibly indicates that other core histone acetylation sites could be important in fly or that hyperacetylation of histones might have an additional function other than facilitating histone release.
The importance of H4 hyperacetylation for mechanisms other than histone release is further supported by our inhibitor studies with anacardic acid. Anacardic acid is a small molecule compound isolated from cashew nut shell liquid and a known inhibitor of p300 and PCAF families of HAT enzymes [Balasubramanyam et al. Citation2003]. Recently, it was also shown to inhibit Tip60, a HAT from the MYST family, and is therefore thought to be a rather general inhibitor of HAT activity in vitro and in vivo [Sun et al. Citation2006]. As shown in this study, AA treatment of intact pupal testes as well as isolated spermatid cysts leads to a severe lack in histone H4 acetylation in all pre-switch stages of Drosophila spermatids. This lack of acetylation is accompanied by a compacted chromatin structure, as visualized by Hoechst staining in squash preparations and also in nuclei of isolated cysts in culture. In contrast to TSA, after AA treatment, we observed no further development of spermatid cysts in culture and no histone to protamine switch occurred in pupal testes. In our view, this supports the hypothesis that H4 hyperacetylation mediates a more open chromatin structure not only to facilitate histone release but also to fulfill roles crucial to the development of mature spermatids in Drosophila.
Lack of histone acetylation may lead to arrested development by several possible paths. For example, histone acetylation may be involved in regulating transcription of specific genes. Despite the absence of general transcription in Drosophila spermiogenesis, the postmeiotic transcription of a small set of genes, grouped under the name of “cups and comets”, was discovered after the round nuclei stage [Barreau et al. Citation2008]. In studies with inhibitors of transcription, it was shown that spermatid development still takes place; however, only cysts up to the round nuclei stage were described [Gould-Somero and Holland Citation1974]. While a single role for hyperacetylated H4 in regulating transcription is doubtful, it is possible that a lack of this acetylation blocks transcription of the cup and comet genes, and hence, any further development. Another possible major role for histone acetylation is directly connected to the induced chromatin compaction phenotype. As already proposed for rooster spermatids by Oliva and Mezquita [Citation1982], increased histone acetylation opens up the chromatin structure, thereby facilitating access of chromosomal proteins fulfilling crucial roles for spermatid development. Finally, these mechanisms may be monitored by a checkpoint system sensing errors in the orchestrated progression of the independent pathways involved in spermiogenesis. This system would arrest the development of faulty spermatid cysts and therefore ensure that only fertile spermatids reach maturity.
Further investigations will need to address these possible roles of histone hyperacetylation in spermatids of such diverse species as mammals, fish, squid, and insect. Here, Drosophila could, yet again, serve as a resourceful model organism to study male fertility in the context of chromatin reorganization.
MATERIALS AND METHODS
Fly Strains
Fly strains were maintained at 18°C or 25°C on standard medium. A fly strain expressing ProtamineB-eGFP was generated by Jayaramaiah-Raja and Renkawitz-Pohl [Citation2005] and a strain expressing Tpl94D-eGFP by Rathke et al. [Citation2007]. For in vivo time-lapse imaging, each of these strains were crossed with flies expressing H2AvD-RFP (a kind gift from C. Wenzl [Clarkson and Saint Citation1999]) to obtain stable, balanced, double-transgenic strains.
Culture of Pupal Testes and Isolated Spermatid Cysts
The protocol for culturing Drosophila pupal testes and spermatid cysts was adapted from Cross and Shellenbarger [Citation1979] and Noguchi and Miller [Citation2003]. In brief, pupal testes at approximately one day after puparium formation were dissected in Shields and Sang M3 insect culture medium (Sigma) supplemented with 10% fetal bovine serum (heat inactivated, insect culture tested, Sigma) as well as 100 U/ml penicillin and 100 μg/ml streptomycin (Gibco-Invitrogen). To obtain intact spermatid cysts, dissected pupal testes were disrupted with thin needles and cysts gently squeezed out of the testis in glass-bottom culture dishes (MatTek). Isolated cysts were staged on the one hand according to morphology as assessed using phase contrast microscopy and on the other hand according to expression of H2AvD-RFP or ProtamineB-eGFP as assessed in fluorescence microscopy. Cysts were transferred to separate culture dishes with drawn out glass Pasteur pipettes. All cultures were incubated at 25°C. Images for time-lapse recordings of cultures were acquired with a Nikon Eclipse TE 2000-S inverted microscope equipped with a Nikon DXM 1200F digital camera and a heating table (MiniTüb HT 50).
Inhibitor Treatment
Cultures of intact pupal testes and isolated cysts were treated with inhibitors for ca. 24 h before fixation and further processing if not stated otherwise in results. For treatment of the pupal testes cultures, generally, eight pupal testes for each inhibitor or control per experiment were used. The experiments were repeated between three and six times. Anacardic acid (Merck Biosciences) was dissolved in DMSO to obtain a 28.69 mM stock solution. Trichostatin A (Cell Signalling Tech.) was dissolved in ethanol to obtain a 4 mM stock solution. Garcinol (Biomol Int.) was dissolved in DMSO to obtain a 16.6 mM stock solution. For treatment, inhibitors were diluted appropriately in culture medium and added to the culture chambers. Control cultures with solvent alone were analyzed in parallel.
Immunofluorescence
For immunofluorescence analysis, cultured pupal testes were squashed and treated essentially as described in Hime et al. [Citation1996]. DNA was stained with Hoechst 33258. Histone H3K9 acetylation was detected either with antibody “Anti-Acetyl-Histone H3 [Ac-Lys9]” (Sigma, Cat.No. H9286) in 1:1500 dilution or with antibody “Rabbit polyclonal to Histone H3 (acetyl K9)” (abcam, Cat.No. ab4441) in 1:200 dilution. Histone H4R3-dimethylation was detected with antibody “Anti-dimethyl-Histone H4 (Arg3)” (Millipore, Cat.No. 07-213) in 1:200 dilution. These primary antibodies were marked with anti-rabbit, Cy3-conjugated antibody (Dianova) in 1:100 dilution.
Acetylation of histone H4 was detected with a 1:200 dilution of antibody “Anti-acetyl-Histone H4” (Upstate, Cat.No. 06-598) recognizing acetylation of H4K5, H4K8, H4K12, and H4K16. This antibody was used in conjunction with anti-rabbit, Cy5-conjugated antibody (Dianova) in 1:100 dilution.
To detect DNA single- and double-strand breaks a terminal dUTP-transferase nick end labelling (TUNEL) assay was used according to the manufacturer's instructions (In Situ Cell Death Detection Kit – TMR red, Roche).
Samples were analysed with a Zeiss Axioplan 2 imaging microscope. Images were acquired with a Zeiss AxioCam MRm digital camera and with equal exposure times for control and treated samples.
ACKNOWLEDGMENTS
We thank Sunil Jayaramaiah Raja for initial discussion of this project, Bridlin Barckmann and Christina Rathke for stimulating discussions and critical reading, and Katja Gessner for excellent secretarial assistance. We also thank Prof. Dr. Uta M. Bauer for kindly providing the H4R3-dimethylation antibody and Prof. Dr. Monika Hassel for use of the inverse microscope with imaging facility. This work was supported by the Deutsche Forschunsgemeinschaft (Forschergruppe 531 “Chromatin mediated biological decisions”, Re628/12-3 to R. R.-P.).
Declaration of Interest: The authors report no conflicts of interest. The authors alone are responsible for the content and writing of the paper.
REFERENCES
- Aoki, V. W., Moskovtsev, S. I., Willis, J., Liu, L., Mullen, J. B. M. and Carrell, D. T. (2005) DNA integrity is compromised in protamine-deficient human sperm. J Androl 26:741–748.
- Arpanahi, A., Brinkworth, M., Iles, D., Krawetz, S. A., Paradowska, A., Platts, A. E., Saida, M., Steger, K., Tedder, P. and Miller, D. (2009) Endonuclease-sensitive regions of human spermatozoal chromatin are highly enriched in promoter and CTCF binding sequences. Genome Res 19:1338–1349.
- Ausiò, J. (1999) Histone H1 and evolution of sperm nuclear basic proteins. J Biol Chem 274:31115–31118.
- Balasubramanyam, K., Swaminathan, V., Ranganathan, A. and Kundu, T. K. (2003) Small molecule modulators of histone acetyltransferase p300. J Biol Chem 278:19134–19140.
- Balasubramanyam, K., Altaf, M., Varier, R. A., Swaminathan, V., Ravindran, A., Sadhale, P. P. and Kundu, T. P. (2004) Polyisoprenylated benzophenone, garcinol, a natural histone acetyltransferase inhibitor, represses chromatin transcription and alters global gene expression. J Biol Chem 279:33716–33726.
- Barreau, C., Benson, E., Gudmannsdottir, E., Newton, F. and White-Cooper, H. (2008) Post-meiotic transcription in Drosophila testes. Development 135:1897–1902.
- Bodenstein, D. (1950) The postembryonic development of Drosophila. In: Biology of Drosophila, Demerec, M. (Ed.), NY: John Wiley and Sons; pp. 275–364.
- Braun, R. E. (2001) Packaging paternal chromosomes with protamine. Nat Genet 28:10–12.
- Brewer, L. R., Corzett, M. and Balhorn, R. (1999) Protamine-induced condensation and decondensation of the same DNA molecule. Science 286:120–123.
- Carrell, D. T., Emery, B. R. and Hammoud, S. (2007) Altered protmaine expression and diminished spermatogenesis: what is the link? Hum Reprod Update 13:313–327.
- Cho, C., Willis, W. D., Goulding, E. H., Jung-Ha, H., Choi, Y. C., Hecht, N. B. and Eddy, E. M. (2001) Haploinsufficiency of protamine−1 or −2 causes infertility in mice. Nat Genet 28:82–86.
- Cho, C., Jung-Ha, H., Willis, W. D., Goulding, E. H., Stein, P., Xu, Z., Schultz, R. M., Hecht, N. B. and Eddy, E. M. (2003) Protamine 2 deficiency leads to sperm DNA damage and embryo death in mice. Biol Reprod 69:211–217.
- Christensen, M. E., Rattner, J. B. and Dixon, G. H. (1984) Hyperacetylation of histone H4 promotes chromatin decondensation prior to histone replacement by protamines during spermatogenesis in rainbow trout. Nucleic Acids Res 12:4575–4592.
- Clarkson, M. and Saint, R. (1999) A His2AvDGFP fusion gene complements a lethal His2AvD mutant allele and provides an in vivo marker for Drosophila chromosome behavior. DNA Cell Biol 18:457–462.
- Cross, D. P. and Shellenbarger, D. L. (1979) The dynamics of Drosophila melanogaster spermatogenesis in in vitro cultures. J Embryol exp Morph 53:345–351.
- Drabent, B., Bode, C., Bramlage, B. and Doenecke, D. (1996) Expression of the mouse testicular histone gene H1t during spermatogenesis. Histochem Cell Biol 106:247–251.
- Fenic, I., Sonnack, V., Failing, K., Bergmann, M. and Steger, K. (2004) In vivo effects of histone-deacetylase inhibitor trichostatin-A on murine spermatogenesis. J Androl 25:811–818.
- Fuller, M. T. (1993) Spermatogenesis. In: The Development of Drosophila melanogaster, vol. I. Bate, M. and Martinez-Arias, A. (Eds.), NY: CSHL Press; pp. 71–147.
- Gould-Somero, M. and Holland, L. (1974) The timing of RNA synthesis for spermiogenesis in organ cultures of Drosophila melanogaster testes. Wilhelm Roux' Archive 174:133–148.
- Govin, J., Caron, C., Lestrat, C., Rousseaux, S. and Khochbin, S. (2004) The role of histones in chromatin remodelling during mammalian spermiogenesis. Eur J Biochem 271:3459–3469.
- Hammoud, S. S., Nix, D. A., Zhang, H., Purwar, J., Carrell, D. T. and Cairns, B. R. (2009) Distinctive chromatin in human sperm packages genes for embryo development. Nature 460:473–478.
- Hazzouri, M., Pivot-Pajot, C., Faure, A.-K., Usson, Y., Pelletier, R., Sèle, B., Khochbin, S. and Rousseaux, S. (2000) Regulated hyperacetylation of core histones during mouse spermatogenesis: involvement of histone-deacetylases. Eur J Cell Biol 79:950–960.
- Hecht, N., Behr, R., Hild, A., Bergmann, M., Weidner, W. and Steger, K. (2009) The common marmoset (Callithrix jacchus) as a model for histone and protamine expression during human spermatogenesis. Hum Reprod 24:536–545.
- Hempel, L. U., Rathke, C., Raja, S. J. and Renkawitz-Pohl, R. (2006) In Drosophila, don juan and don juan like encode proteins of the spermatid nucleus and the flagellum and both are regulated at the transcriptional level by the TAF II80 cannonball while translational repression is achieved by distinct elements. Dev Dyn 235:1053–1064.
- Hennig, W. (2003) Chromosomal proteins in the spermatogenesis of Drosophila. Chromosoma 111:489–494
- Hime, G. R., Brill, J. A. and Fuller, M. T. (1996) Assembly of ring canals in the male germ line from structural components of the contractile ring. J Cell Sci 109:2779–2788.
- Holstein, A. F., Schulze, W. and Davidoff, M. (2003) Understanding spermatogenesis is a prerequisite for treatment. Reprod Biol Endocrinol 1:107.
- Jayaramaiah-Raja, S. and Renkawitz-Pohl, R. (2005) Replacement by Drosophila melanogaster protamines and Mst77F of histones during chromatin condensation in late spermatids and role of sesame in the removal of these proteins from the male pronucleus. Mol Cell Biol 25:6165–6177.
- Kawamoto, T., Kawai, K., Kodama, T., Yokokura, T. and Niki, Y. (2008) Autonomous differentiation of Drosophila spermatogonia in vitro. Develop Growth Differ 50:623–632.
- Kimmins, S. and Sassone-Corsi, P. (2005) Chromatin remodelling and epigenetic features of germ cells. Nature 434:583–589.
- Kuroda, Y. (1974) Spermatogenesis in pharate adult testes of Drosophila in tissue cultures without ecdysones. J Insect Physiol 20:637–640.
- Kurtz, K., Martínez-Soler, F., Ausió, J. and Chriva, M. (2007) Acetylation of histone H4 in complex structural transitions of spermiogenic chromatin. J Cell Biochem 102:1432–1441.
- Laberge, R. M. and Boissonneault, G. (2005) On the nature and origin of DNA strand breaks in elongating spermatids. Biol Reprod 73:289–296.
- Leduc, F., Maquennehan, V., Bikond Nkoma, G. and Boissonneault, G. (2008) DNA damage response during chromatin remodeling in elongating spermatids of mice. Biol Reprod 78:324–332.
- Lewis, J. D., Song, Y., de Jong, M. E., Bagha, S. M. and Ausió, J. (2003) A walk through vertebrate and invertebrate protamines. Chromosoma 111:473–482.
- Lindsley, D. L. and Lifschytz, E. (1972) The genetic control of spermatogenesis in Drosophila. In: Symposium on the Genetics of the Spermatozoon, Beatty, R. A. and Gluecksohn-Waelsch, S. (Eds.), UK: Academic Press Edinburgh; pp. 203–222.
- Mai, A., Massa, S., Rotili, D., Cerbara, I., Valente, S., Pezzi, R., Simeoni, S. and Ragno, R. (2005) Histone deacetylation in epigenetics: An attractive target for anticancer therapy. Med Res Rev 25:261–309.
- Meistrich, M. L., Trostle-Weige, P. K., Lin, R., Bhatnagar, Y. M. and Allis, C. D. (1992) Highly acetylated H4 is associated with histone displacement in rat spermatids. Mol Reprod Dev 31:170–181.
- Meistrich, M. L., Mohapatra, B., Shirley, C. R. and Zhao, M. (2003) Roles of transition nuclear proteins in spermiogenesis. Chromosoma 111:483–488.
- Nair, M., Nagamori, I., Sun, P., Mishra, D. P., Rhéaume, C., Li, B., Sassone-Corsi, P. and Dai, X. (2008) Nuclear regulator Pygo2 controls spermiogenesis and histone H3 acetylation. Dev Biol 320:446–455.
- Noguchi, T. and Miller, K. G. (2003) A role for actin dynamics in individualization during spermatogenesis in Drosophila melanogaster. Development 130:1805–1816.
- Oliva, R. and Mezquita, C. (1982) Histone H4 hyperacetylation and rapid turnover of its acetyl groups in transcriptionally inactive rooster testis spermatids. Nucleic Acids Res 10:8049–8059.
- Oliva, R. (2006) Protamines and male infertility. Hum Reprod Update 12:417–435.
- Rathke, C., Baarends, W. M., Jayaramaiah-Raja, S., Bartkuhn, M., Renkawitz, R. and Renkawitz-Pohl, R. (2007) Transition from a nucleosome-based to a protamine-based chromatin configuration during spermiogenesis in Drosophila. J Cell Sci 120:1689–1700.
- Rathke, C., Barckmann, B., Burkhard, S., Jayaramaiah-Raja, S., Roote, J. and Renkawitz-Pohl, R. (2009) Distinct functions of Mst77F and protamines in nuclear shaping and chromatin condensation during Drosophila spermiogenesis. EJCB (in press).
- Renkawitz-Pohl, R., Hempel, L., Hollmann, M. and Schäfer, M. (2005) Spermatogenesis. In: Comprehensive Molecular Insect Science, Gilbert, L. I., Iatrou, K. and Gill, S. S. (Eds.), Elsevier Ltd.; pp. 157–177.
- Rousseaux, S., Caron, C., Govin, J., Lestrat, C., Faure, A. K. and Khochbin, S. (2005) Establishment of male-specific epigenetic information. Gene 345:139–153.
- Santel, A., Winhauer, T., Blümer, N. and Renkawitz-Pohl R. (1997) The Drosophila don juan (dj) gene encodes a novel sperm specific protein component characterized by an unusual domain of a repetitive amino acid motif. Mech Dev 64:19–30.
- Sassone-Corsi, P. (2002) Unique chromatin remodeling and transcriptional regulation in spermatogenesis. Science 296:2176–2178.
- Sonnack, V., Failing, K., Bergmann, M. and Steger, K. (2002) Expression of hyperacetylated histone H4 during normal and impaired human spermatogenesis. Andrologia 34:384–390.
- Steger, K., Fink, L., Failing, K., Bohle, R. M., Kliesch, S., Weidner, W. and Bergmann, M. (2003) Decreased protamine-1 transcript levels in testes from infertile men. Mol Hum Reprod 9:331–336.
- Sun, Y., Jiang, X., Chen, S. and Price, B. D. (2006) Inhibition of histone acetyltransferase activity by anacardic acid sensitizes tumor cells to ionizing radiation. FEBS Lett. 580:4353–4356.
- Tokuyasu, K. T. (1975) Dynamics of spermiogenesis in Drosophila melanogaster. VI. Significance of “onion” Nebenkern formation. J Ultrastruct Res 53:93–112.
- Torregrosa, N., Domíngez-Fandos, D., Camejo, M. I., Shirley, C. R., Meistrich, M. L., Ballescà, J. L. and Oliva, R. (2006) Protamine 2 precursors, protamine1/protamine2 ration, DNA integrity and other sperm parameters in infertile patients. Hum Reprod 21:2084–2089.
- Varier, R. A., Swaminathan, V., Balasubramanyam, K. and Kundu, T. P. (2004) Implications of small molecule activators and inhibitors of histone acetyltransferases in chromatin therapy. Biochem Pharmacol 68:1215–1220.
- Ward, W. S. (1993) Deoxyribonucleic acid loop domain tertiary structure in mammalian spermatozoa. Biol Reprod 48:1193–1201.
- Yan, W., Lang, M., Burns, K. H. and Matzuk, M. M. (2003) HILS1 is a spermatid-specific linker histone H1-like protein implicated in chromatin remodeling during mammalian spermiogenesis. PNAS 100:10546–10551.
- Yoshida, M., Kijima, M., Akita, M. and Beppu, T. (1990) Potent and specific inhibition of mammalian histone deacetylase both in vivo and in vitro by trichostatin A. J Biol Chem 265:17174–17179.
- Zhao, M., Shirley, C. R., Mounsey, S. and Meistrich, M. L. (2004) Nucleoprotein transitions during spermiogenesis in mice with transition nuclear protein Tnp1 and Tnp2 mutations. Biol Reprod 71:1016–1025.
- Zhong, L. and Belote, J. M. (2007) The testis-specific proteasome subunit Prosα6T of D. melanogaster is required for individualization and nuclear maturation during spermatogenesis. Development 134:3517–3525.