Abstract
The morphology and size of spermatozoa hinder the study of the functional properties of the spermatozoa plasma membrane. However, some studies have revealed the presence of a number of ion channels in this cell. We set out to measure the endogenous currents and to study the effect of the venom of the Chilean black widow spider (Latrodectus mactans). By patch-clamping bovine spermatozoa our results indicate the presence of an outwardly rectifying current, sensitive to changes in K+ concentration (30–140 mM) and to tetraethylammonium (TEA, 10–100 mM). The application of the venom (7.5 µg/ml) blocks these K+ currents and then alters the passive properties of the plasma membrane. This leads to the entry of Ca++, reflected by a change in basal fluorescent units (5±2 at 35±10 FAU). The Ca++ influx follows a reduction in the membrane conductance (control 22±2; venom 10±1 pS), as calcium channels open in accord with voltage dependence.
Abbreviations | ||
TEA: | = | tetraethylammonium |
FAU: | = | fluorescent arbitrary units |
pS: | = | membrane conductance |
CCVD: | = | calcium channel voltage dependent |
PBS: | = | phosphate saline buffer solution |
INTRODUCTION
There is strong experimental evidence that mature mammalian spermatozoa have several ionic-conductors, including those driven by voltage dependent K+ channels [Darszon et al. Citation1996; Labarca et al. Citation1995; Nuccitelli and Ferguson Citation1994]. Recent reports using whole cell patch-clamping to study ion-conductance, have described the functional properties of Ca++ channels (CATsper), which are key components of capacitation [Darszon et al. Citation2005; Wennemuth et al. Citation2000].
The mammalian spermatozoa acquire the functional capacity to fertilize an egg during their trajectory along the female genital tract [Boni et al. Citation2007]. In this process the plasma membrane potential is hyperpolarized by the activation of pH-sensitive K+-channels. This leads to an increase in Ca++ permeability [Kumar et al. Citation2000; Linares-Hernandez et al. Citation1998; Shi and Ma Citation1998]. However, this increase of intracellular Ca++ is not the sole cause of capacitation, it has also been suggested that the intracellular pH and transient plasma membrane depolarization play a role [Fraire-Zamora and Gonzalez-Martinez Citation2004; Neri-Vidaurri Pdel et al. Citation2006] similar to the series of events that occur in somatic cells [Baker et al. Citation1973]. The detailed mechanism that leads to spermatozoa capacitation remains to be detailed.
In a previous report we described that the venom of the Chilean black widow spider Latrodectus mactans increased the concentration of intracellular Ca++ in spermatozoa [Romero et al. Citation2007]. Furthermore, this venom is known to block the TEA-sensitive K+ currents in neurons [Parodi and Romero Citation2008] as well as endogenous K+ currents of Xenopus laevis oocytes [Parodi et al. Citation2008]. To assess the effect of this venom on the plasma membrane potential of bovine spermatozoa we used the nystatine-perforated patch-clamp technique. The results that begin to dissect the molecular components of capacitation are reported below.
RESULTS
Exposure to Venom Increases Free Intracellular Calcium
It has been shown by spectrophotometry that exposing spermatozoa to the venom of the Chilean L. mactans leads to an increase in pH and free intracellular Ca++ [Romero et al. Citation2007]. To extend these results we assessed the changes in the intracellular Ca++ by fluorometry and patch-clamp. The spermatozoa loaded with Fluo-3 is shown in . As expected, the basal level of free intracellular Ca++ is low, with most of the cells emitting some faint fluorescence. In contrast, 30 min after the addition of the venom, the number of cells exhibiting fluorescence increased considerably (). This was consistently observed even after 3 repetitions suggesting that sudden depolarization of the plasma membrane may induce similar effects.
FIGURE 1 Venom-induced rise of intracellular Ca++. Sample images of Fluo-3 AM loaded spermatozoa in the absence (A), or presence (B) of total venom (7.5 μg/ml). (C), fluorometric records. (D), fluorescent arbitrary units (FAU) of spermatozoa in high K+ (30 mM) or venom (7.5 μg/ml); the bars represent means±SD of three different experiments. Calibration bar=5 μm.
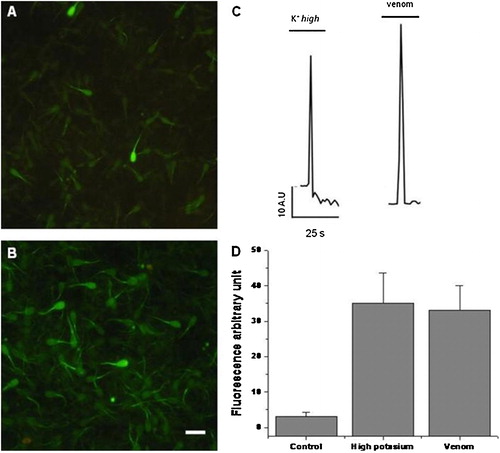
shows typical fluorescence traces of the spermatozoa midpiece loaded with Fluo-3 acquired with sensicam, for time lapse assessment with fast perfusion of either venom or a high concentration of K+ (30 mM). Notice the rise in fluorescence and the immediate decrease of the emission after a few s as measured by its change in the midpiece. The fluorometric data is summarized in showing that both venom and K+ increased the free Ca++ up to 35 fluorescent arbitrary units (FAU) as compared to the uninduced control. Together this data suggests that spermatozoa calcium increases after depolarization or perfusion with venom.
Patch-Clamping Reveals a TEA-Sensitive K+ Current
Spermatozoa exhibitsvaried permeability to ions reflecting several channels, including the voltage- sensitive K+ and −Ca++ [Darszon et al. Citation2006]. As illustrated in , whole-cell patch-clamping of spermatozoa showed inward and outward currents. P pulses that ranged between −100 mV to +100 mV were more sensitive to 50 mM TEA. These currents are plotted as a current/ voltage curve (I/V) (). The TEA-sensitivity of the outward current is consistent with the presence of a voltage dependent potassium channel (Kv channel; IUPHAR classification). These channels are crucial towards maintaining the plasma membrane potential [Gutman et al. Citation2003]. Increasing the concentration of extracellular K+ shifted the equilibrium potential to more negative values (). This is in accord with that predicted by the Nerst's equation ( and ) indicating that the current was due to K+ TEA-sensitive channels.
FIGURE 2 A TEA-sensitive outwardly rectifying K+. (A), sample records of membrane currents obtained in the absence or the presence of 5 mM TEA. (B), I/V relation from the sample currents (n=6). (C), I/V plot in increasing concentrations of K+.
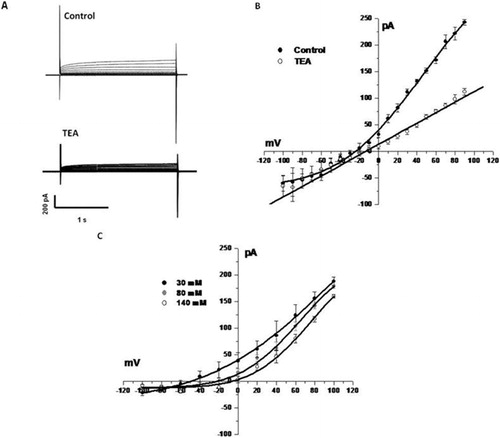
TABLE 1 Calculated [I/pA = f (Vm/mV)] and Experimental Reversal Membrane Potentials.
Venom Alters the TEA-Sensitive K+ Current
Outwardly-rectifying currents were efficiently and reversibly blocked when the spermatozoa were exposed to the venom (). The changes in membrane conductance are summarized in . Venom reduces the membrane conductance from 22±1 pS to approximately 10±2 pS (). In contrast upon exposure to venom, the membrane resistance changed from 200 mΩ±12 to 800 mΩ±23, suggesting an important change in the passive membrane properties.
FIGURE 3 Venom blocks the TEA-sensitive current. (A), I/V relation in the absence or presence of 7.5 μg/ml venom. (B), the membrane conductance is reduced in spermatozoa exposed to the venom. (C), comparative effect of venom, high K+, and increasing concentrations of TEA (10 to 50 mM) on the prepulse current. The insert shows sample recordings of the prepulse in control cells and cells exposed to venom. The bars represent means±SD of six different experiments.*
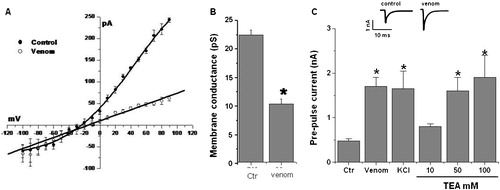
We explored whether venom also modulates spermatozoa K+ currents by increasing the free intracellular Ca++ as known to occur in somatic cells [Romero et al. Citation2007]. Briefly hyperpolarizing the spermatozoa plasma membrane from −60 mV to −80 mV activates a calcium-dependent K+ current (, insert). The tail-current derived from this response in the presence of either venom, high K+, or TEA is plotted in . In most cases at 7.5 μg/ml venom with 30 mM potassium or 50 to 100 mM TEA the K+ currents were blocked. As expected the pre-pulse currents were approximately 300% higher than the control. Interestingly, 10 mM TEA did not affect the current.
DISCUSSION
A previous study has indicated that the venom induced cellular effect is mediated by low molecular weight heat sensitive peptides present in the venom, [Parodi and Romero Citation2008]. As described above, the venom of the Chilean L. mactans is effective even in the absence of α-latrotoxin, which is the primary component described for this venom in another species of spider [Ushkaryov et al. Citation2008]. The results reported in this study suggest that the venom of L. mactans increases the free intracellular Ca++ negatively impacting the TEA-sensitive K+ spermatozoa current. All conductances are crucial to spermatozoa capacitation.
After several min of exposure of the spermatozoa to the venom, free intracellular Ca++ rises. As shown in and , the change in the membrane conductance after exposure to venom suggests depolarization and calcium channel voltage dependent (CCVD) activation in the spermatozoa cells. This may reflect the blockage of voltage-dependent K+ channels leading to the entrance of Ca++ from the extracellular medium in accord with previous studies that suggested that the venom inhibits several K+ conductances [Parodi and Romero Citation2008; Parodi et al. Citation2008]. Like the oocyte Kv1.1 s [Parodi et al. Citation2008], this channel is also present in the spermatozoa midpiece [Darszon et al. Citation1999]. Since Kv1.1 is integral to membrane potential regulation [Gutman et al. Citation2003] we compared the effect with high K+ added in the medium. In both cases the concentration of free Ca++ exhibited a transient rise and sharp fall as detected by spermatozoal midpiece fluorescence. However, a rise in Ca++ was observed throughout the cell after long periods of time. This is consistent with the view that a CCVD was present [Darszon et al. Citation2005]. A direct pharmacological study is required to specify the Ca++ and K+ currents involved.
The molecular identity of the K+ channel modulated by the venom was indirectly assessed by the activation kinetics and TEA-sensitivity of the currents. At 5 μM TEA-blocks the outward-rectifying K+ current and likely represents a Kv1-like current [Marconi et al. Citation2008], although mouse brain large-conductance (mSlo) has also been identified in spermatozoa [Navarro et al. Citation2008]. The currents blocked by the spider venom appear similar to those found in frog oocytes and neurons in culture [Parodi and Romero Citation2008; Parodi et al. Citation2008] although differences in the expression profiles of Kv channels [Darszon et al. Citation2006] among species have been observed. Nevertheless the functional data reported here and elsewhere [Darszon et al. Citation2006; Marconi et al. Citation2008; Navarro et al. Citation2008] is consistent with the view that Kv channels are integral to the spermatozoa.
Other natural sources of spermicidal action have been reported, including scorpion toxins and plant extracts [Harat et al. Citation2008; Lopez-Gonzalez et al. Citation2003]. Together with the previous studies of the spermicidal properties of the venom of the Chilean L. mactans we were enabled, with a new set of pharmacological tools, to identify the active compound(s) that mediate the modulation of ionic-conductances. Perhaps they could provide a resource for a new generation of contraceptives.
MATERIALS AND METHODS
Spider Retrieval
Female adult L. mactans were captured in Chile during the summer months (December 2006 and January 2007) from the area of “Alto Bio Bio” in the Bio-Bio state (72°16′51″ W, 7°45′24″ S) as previously described [Romero et al. Citation2003]. Care was taken not to damage the breeding zones. The specimens were separately maintained in individual jars. To stimulate the production and concentration of venom in the glands, the spiders were raised without food and only given water for 30 days.
Venom Retrieval
The spiders were immersed in liquid nitrogen and after 1 min transferred to a phosphate saline buffer solution (PBS: 0.1 M NaH2PO4, 0.01 M Na2HPO4, 1.35 M NaCl, pH 7.4) at 4°C. The glands were removed and the membrane that binds them to the base was sectioned. Each gland, was placed into a tube containing PBS (25 pairs of glands for 100 μl of PBS) and homogenized. The homogenate was immediately centrifuged at 1,000×g for 15 min. The supernatant was subsequently aliquoted, and frozen at −20°C. The protein content of the venom was determined by a modification of the Bradford method (BioRad Protein Assay).
Spermatozoa
Bovine spermatozoa were obtained from samples cryopreserved in liquid nitrogen, which were thawed at 35°C for 1 min then suspended in human tubal fluid (HTF) medium supplemented with 3% BSA. Spermatozoa were selected by the swim-up technique (recommendation of [World Health Organization Citation1999]) and motile spermatozoa were placed in a recording chamber coated with poly-L-lysine (0.2 mg/mL). The recording chamber was then washed with a standard external solution that contained 150 mM NaCl, 5.4 mM KCl, 2.0 mM CaCl2, 1.0 mM MgCl2, 10 mM glucose buffered with 10 mM HEPES, pH 7.4 buffer.
Micropipette Preparation
Borosilicate glass capillary tubes 1.5 mm of internal diameter (Sutter Instrument Co., CA, USA) were placed in a vertical puller (Narishige PP 830 m Barishige, Tokyo, Japon) and then treated with a micropolisher (Narishige MF 900) to obtain a micropipette tip with electrical resistances ranging from 10 to 12 MΩ.
Electrophysiology
Electrical currents were recorded by using the perforated whole-cell patch-clamp technique [Horn and Marty Citation1988] at room temperature, using a patch-clamp amplifier (Warner PC-501A). For whole-cell recordings, the external solution contained 150 mM NaCl, 5.4 mM KCl, 2.0 mM CaCl2, 1.0 mM MgCl2, 10 mM glucose buffered with 10 mM HEPES, pH 7.4 buffer. The internal solution contained 120 mM KCl, 2.0 mM MgCl2, 2 mM ATP-Na2-ATP, 10 mM BAPTA, 0.5 mM GTP buffered with 10 mM HEPES, pH 7.4 buffer. For perforated patch-clamp 10 μg/ml nystatine was added [Ermishkin et al. Citation1976]. For some experiments, the external solution included either 7.5 µg/ml of venom or 5–100 mM TEA. Spermatozoa were clamped at a holding potential of −60 mV. The ionic currents were captured online and digitized at a sampling rate of 1.2 kHz using pClamp 9 (Molecular Devices, Axon) after being filtered (internal 4 Bessel; frequency cutoff=3 kHz). Pulse protocols, data capture, and recording analysis were performed using pClampfit 9.0 software (Molecular Devices, Axon).
Fluo-3 AM Loading
Bovine spermatozoa were loaded with 5 μM Fluo-3 AM in pluronic acid/DMSO, 10% w/v (Molecular Probes, Eugene, OR, USA) and mounted in a fast-switching flow perfusion chamber adapted on the stage of an inverted fluorescent microscope (Eclipse TE, Nikon) equipped with a xenon lamp and a 40× objective (22–24°C). The cells were briefly illuminated (200 ms) for fast record real time analysis, using a computer-controlled Lambda 10-2 filter wheel (Sutter Instruments). Regions of interest (ROI) were simultaneously selected on midpiece containing Fluo-3 fluorescence (excitation 480 nm, emission 510 nm). Images were collected at 2–5 s intervals during a continuous 5-min period or at 0 or 30 min. The imaging was carried out with a 12 bit cooled SensiCam camera (PCO, Germany), using the imaging software Axon Workbench 2.2 (Axon Instruments). The spermatozoa were exposed to 7.5 µg/ml of total venom () or 30 mM potassium () by bath perfusion, and changes in the emission of midpiece fluorescence, measured in FAU and scored.
ACKNOWLEDGMENTS
Fernando Romero, Raul Sanchez, and Jorge Parodi were supported by the Fondef-Conicyt Chile No DO5I10416. Ataúlfo Martinez-Torres was supported by CONACYT 55025 and UNAM-PAPIIT 204806. Jorge Parodi has postdoctoral fellow from CTIC-UNAM and from MIDEPLAN-Chile. Patricia Navarrete has CONICYT grant. We are in debt with Dr. Luis Aguayo for help with measurement equipment for calcium experiments.
REFERENCES
- Baker, P. F., Meves, H. and Ridgway, E. B. (1973) Calcium entry in response to maintained depolarization of squid axons. J Physiol 231:527–548.
- Boni, R., Gualtieri, R., Talevi, R. and Tosti, E. (2007) Calcium and other ion dynamics during gamete maturation and fertilization. Theriogenology 68 Suppl 1:S156–164.
- Darszon, A., Acevedo, J. J., Galindo, B. E., Hernandez-Gonzalez, E. O., Nishigaki, T., Trevino, C. L., Wood, C. and Beltran, C. (2006) Sperm channel diversity and functional multiplicity. Reproduction 131:977–988.
- Darszon, A., Labarca, P., Nishigaki, T. and Espinosa, F. (1999) Ion channels in sperm physiology. Physiol Rev 79:481–510.
- Darszon, A., Lievano, A. and Beltran, C. (1996) Ion channels: key elements in gamete signaling. Curr Top Dev Biol 34:117–167.
- Darszon, A., Nishigaki, T., Wood, C., Trevino, C. L., Felix, R. and Beltran, C. (2005) Calcium channels and Ca2+ fluctuations in sperm physiology. Int Rev Cytol 243:79–172.
- Ermishkin, L. N., Kasumov, K. M. and Potzeluyev, V. M. (1976) Single ionic channels induced in lipid bilayers by polyene antibiotics amphotericin B and nystatine. Nature 262:698–699.
- Fraire-Zamora, J. J. and Gonzalez-Martinez, M. T. (2004) Effect of intracellular pH on depolarization-evoked calcium influx in human sperm. Am J Physiol Cell Physiol 287:C1688–1696.
- Gutman, G. A., Chandy, K. G., Adelman, J. P., Aiyar, J., Bayliss, D. A., Clapham, D. E., et al. (2003) International Union of Pharmacology. XLI. Compendium of voltage-gated ion channels: potassium channels. Pharmacol Rev 55:583–586.
- Harat, Z. N., Sadeghi, M. R., Sadeghipour, H. R., Kamalinejad, M. and Eshraghian, M. R. (2008) Immobilization effect of Ruta graveolens L. on human sperm: a new hope for male contraception. J Ethnopharmacol 115:36–41.
- Horn, R. and Marty, A. (1988) Muscarinic activation of ionic currents measured by a new whole-cell recording method. J Gen Physiol 92:145–159.
- Kumar, S., Ying, Y. K., Hong, P. and Maddaiah, V. T. (2000) Potassium increases intracellular calcium simulating progesterone action in human sperm. Arch Androl 44:93–101.
- Labarca, P., Zapata, O., Beltran, C. and Darszon, A. (1995) Ion channels from the mouse sperm plasma membrane in planar lipid bilayers. Zygote 3:199–206.
- Linares-Hernandez, L., Guzman-Grenfell, A. M., Hicks-Gomez, J. J. and Gonzalez-Martinez, M. T. (1998) Voltage-dependent calcium influx in human sperm assessed by simultaneous optical detection of intracellular calcium and membrane potential. Biochim Biophys Acta 1372:1–12.
- Lopez-Gonzalez, I., Olamendi-Portugal, T., De la Vega-Beltran, J. L., Van der Walt, J., Dyason, K., Possani, L. D., Felix, R. and Darszon, A. (2003) Scorpion toxins that block T-type Ca2+ channels in spermatogenic cells inhibit the sperm acrosome reaction. Biochem Biophys Res Commun 300:408–414.
- Marconi, M., Sanchez, R., Ulrich, H. and Romero, F. (2008) Potassium current in mature bovine spermatozoa. Syst Biol Reprod Med 54:231–239.
- Navarro, B., Kirichok, Y., Chung, J. J. and Clapham, D. E. (2008) Ion channels that control fertility in mammalian spermatozoa. Int J Dev Biol 52:607–613.
- Neri-Vidaurri Pdel, C., Torres-Flores, V. and Gonzalez-Martinez, M. T. (2006) A remarkable increase in the pHi sensitivity of voltage-dependent calcium channels occurs in human sperm incubated in capacitating conditions. Biochem Biophys Res Commun 343:105–109.
- Nuccitelli, R. and Ferguson, J. E. (1994) Fertilization and ion channels. Science 263:988.
- Parodi, J. and Romero, F. (2008) Synaptic effects of low molecular weight components from Chilean Black Widow spider venom. Neurotoxicology 29:1121–1126.
- Parodi, J., Romero, F., Miledi, R. and Martinez-Torres, A. (2008) Some effects of the venom of the Chilean spider Latrodectus mactans on endogenous ion-currents of Xenopus laevis oocytes. Biochem Biophys Res Commun 375:571–575.
- Romero, F., Altieri, E., Urrutia, M. and Jara, J. (2003) Venom of Latrodectus mactans from Chile (Araneae, Theridiidae): effect on smooth muscle. Rev Biol Trop 51:305–312.
- Romero, F., Cunha, M. A., Sanchez, R., Ferreira, A. T., Schor, N. and Oshiro, M. E. (2007) Effects of arachnotoxin on intracellular pH and calcium in human spermatozoa. Fertil Steril 87:1345–1349.
- Shi, Y. L. and Ma, X. H. (1998) Ion-channels reconstituted into lipid bilayer from human sperm plasma membrane. Mol Reprod Dev 50:354–360.
- Ushkaryov, Y. A., Rohou, A. and Sugita, S. (2008) alpha-Latrotoxin and its receptors. Handb Exp Pharmacol 184:171–206.
- Wennemuth, G., Westenbroek, R. E., Xu, T., Hille, B. and Babcock, D. F. (2000) CaV2.2 and CaV2.3 (N- and R-type) Ca2+ channels in depolarization-evoked entry of Ca2+ into mouse sperm. J Biol Chem 275:21210–21217.
- World Health Organization (1999) Commentry: legal and ethical aspects of sperm retrieval. J Law Med Ethics 27:359–361, 295.