Abstract
The present study was conducted in Wistar rats to determine whether prepubertal dietary zinc deficiency causes apoptotic changes in testes. Prepubertal male Wistar rats (40–50 gm) were divided into 3 groups: zinc control (ZC), pairfed (PF), and zinc deficient (ZD). Control and pairfed groups were given a 100 ppm zinc diet while the deficient groups received 1 ppm zinc diet for 2 and 4 weeks (w), respectively. Ultrastructural studies revealed several apoptotic features such as wavy basement membrane, displaced nuclei, chromatin condensation, plasma membrane blebbing, nuclear membrane dissolution, loss of inter-Sertoli cell junctional complexes, and intercellular bridges and deformed mitochondria. A variable spectrum of sperm defects had also been visualized e.g., acrosomal deformities such as decapitation and a ring of condensed chromatin around the nuclear periphery, deformed sperm heads with a condensed nucleus, tail-elements with superfluous cytoplasm, and damage to the mitochondrial sheath and aggregation of spermatozoa within the membrane. This was further supported by TUNEL studies. Apoptotic index, epididymal sperm concentration, motility, and fertility index also revealed a significant (P < 0.05) decrease in zinc deficient groups (2 and 4 w) when compared with their respective control and pairfed groups. All the above findings are indicative that changes observed in the testes after dietary zinc deficiency are due to the onset of apoptosis. Increased apoptotic degeneration in testes may cause irreversible changes in the germ cells associated with decreased epididymal sperm concentration, motility, and fertility index which contributes to the low efficiency of spermatogenesis thereby indicating a possible role of zinc in fertility.
Introduction
Zinc, an essential trace element, is ubiquitously present throughout all biological systems and has abundant and varied functions within these systems [Kaji 2001; Auld 2009; Maret 2009, Tuerk and Fazel 2009]. Zinc deficiency, a global problem, contributes negatively to human health and development [Maret and Sandstead 2008; Walingo 2009]. Moderate and marginal zinc deficiency, due to inadequate intake, is by far more common throughout the world than severe deficiency [Al-Timimi 2009], especially in those stages of life when requirements are increased such as infancy, childhood, and pregnancy [Fesharakinia et al. 2009, Hess and King 2009; Uriu-Adams and Keen 2010]. Zinc affects different aspects of mammalian reproduction [Dissanayake et al. 2009; Björndahl and Kvist 2010]. Low zinc intake by young males of several species including humans, interferes with normal sexual development [Bedwal et al. 1991; Saxena et al. 1993; Bedwal and Bahuguna 1994; Kaji 2001; Dissanayake et al. 2009] and leads to gonadal dysfunction. Zinc also plays an essential role in the synthesis and secretion of luteinizing hormone (LH), follicle-stimulating hormone (FSH) and prolactin, gonadal differentiation, testicular steroidogenesis, and genetic expression of steroid hormone receptors [Bedwal et al. 1991; Bedwal and Bahuguna 1994; Ali et al. 2007]. Insufficient zinc initiates programmed cell death in hepatocytes, glioma, monocytes, fibroblasts, kidney, brain, prostate, and testicular cells [Fraker 2005; Yan et al. 2008; Adamo and Oteiza 2010]. Multiple studies have demonstrated the essential role of Zn2+ for cell survival. Zinc deficiency accelerates apoptosis both in vitro and in vivo [Zalewski et al. 1991; Duffy et al. 2001]. The ability of Zn2+ to prevent DNA fragmentation has been attributed to its inhibitory effect on Ca2+ and Mg2+-dependent endonucleases [Giannakis et al. 1991; Zhivotovsky et al. 1994], although there are numerous targets of zinc in the apoptotic cascade such as caspase-3, caspase-6, bcl-2, p53, etc. [Takahashi et al. 1996; Stennicke and Salvesen 1997; Wolf et al. 1997; Fukamachi et al. 1998; Chai et al. 1999; Jankowski-Hennig et al. 2000; Fanzo et al. 2001; Ho and Ames 2002; Ho et al. 2003], imbalance of either would lead to the initiation of apoptosis. Abnormally accelerated apoptosis of germ cells may lead to an imbalance of cell proliferation and death resulting in spermatogenic impairment [Hassan et al. 2009]. Studies have shown that germ cell degeneration in testes involves an apoptotic mechanism [Billig et al. 1995; Henriksen et al. 1995]. The present study was therefore conducted on Wistar rats to determine whether prepubertal dietary zinc deficiency causes apoptotic changes in testes.
Results
Ultrastructural Studies
Normal ultrastructural details of basement membrane, spermatogonia, spermatocytes, spermatids, spermatozoa, and Sertoli and Leydig cells are evident in the control groups after 2 and 4 weeks (FigsA and 2A). The PF group after 2 and 4 w also showed somewhat normal features except for the appearance of lipid droplets, phagocytic vacuoles, and abundant multivesicular bodies in the cytoplasm (B and 2B). The 2 w ZD group testes displayed a wavy basement membrane with basal lamina, endothelial cells, and disrupted collagen fibres. Cytoplasm showed extensive vacuolization, disruption of intercellular bridges, and abundant multivesicular bodies (C). The ultrastructure of primary spermatocyte nuclei of the 2 w ZD group illustrated early apoptotic changes with chromatin condensation on the periphery. Cytoplasm revealed extensive damage with a large number of multivesicular bodies (D). The nuclei of the 2 w ZD group Leydig cell exhibited peripheral heterochromatin but the juxtanuclear region of cytoplasm showed extensive vacuolization, dilated smooth endoplasmic reticulum (ER), and abundant multivesicular bodies (E). The variable degree of degeneration seems to be more pronounced after 4 w of zinc deficiency. Testes from the 4 w ZD group revealed several apoptotic features such as wavy and thickened basement membrane, dissolution of collagen fibres, dense, irregular, and displaced nuclei, chromatin condensation, plasma membrane blebbing, nuclear membrane dissolution, vacuolization, loss of inter-Sertoli cell junctional complexes and intercellular bridges, and deformed mitochondria (A, B, C, and H). A variable spectrum of sperm defects like acrosomal deformities such as decapitation and a ring of condensed chromatin around the nuclear periphery, deformed sperm heads with a condensed nucleus, tail elements with superfluous cytoplasm, damaged mitochondrial sheath, and aggregation of spermatozoa enclosed by cytoplasm and a common membrane (D, E, F, and G) were also visualized.
Figure 1. Electronmicrograph of control, pairfed, and zinc deficient groups after 2 weeks. The scale bar = 2 µm. (A) Electronmicrograph of 2ZC group testes illustrating normal features of basement membrane (BM) showing basal lamina (bl), collagen fibres (cf), and endothelial cells (ed). Leydig cell nucleus (Nu) revealed characteristic peripheral heterochromatin (Hc). Presence of Type-A Spermatogonia (Sg) with its distinctive spherical nucleus and dense nucleolar body in association with the inner aspect of the nuclear envelope (Ne) is evident along with a large number of mitochondria (mt) with tubular cristae, rough endoplasmic reticulum (rER), ribosomes (r) and lysosomes (Ly) in the cytoplasm. (B) Electronmicrograph of 2PF group testes showing disrupted basement membrane (BM) with basal lamina (bl), collagen fibres (cf), and myoid cell process (My). Sertoli cell nucleus (Sc) exhibiting indentation and cytoplasm showing a large number of multivesicular bodies (mvb), lipid droplets (Ld), dilated smooth endoplasmic reticulum (sER), rough endoplasmic reticulum (rER), inter-Sertoli cell junctional complexes (Is), and parts of spermatocyte nuclei (Sp) with synaptonemal threads (St). (C) Ultrastructure of testes of 2ZD group testes displaying wavy basement membrane (BM) with basal lamina (bl), endothelial cells (ed), and disrupted collagen fibres (cf). Cytoplasm showing extensive vacuolization (V) and disruption of intercellular bridges (Icb), polyribosomes (pr), ribosomes (r), smooth endoplasmic reticulum (sER), rough endoplasmic reticulum (rER), abundant multivesicular bodies (mvb), and displaced Type A spermatogonium nucleus (Nu) showing chromatin conglomerates (Ch) with distinct nuclear envelope (Ne). (D) Ultrastructure of primary spermatocyte nuclei (Sp) of 2ZD group testes illustrating early apoptotic change viz. chromatin condensation (Cc) on the periphery. Extensive cytoplasmic damages with a large number of multivesicular bodies (mvb) are also distinct. (E) Electronmicrograph of 2ZD group testes illustrating juxtanuclear region of cytoplasm showing extensive vacuolization (V), dilated smooth endoplasmic reticulum (sER), and aggregation of multivesicular bodies (mvb). Peripheral heterochromatin (Hc) of Leydig cell nucleus (Nu) are evident.
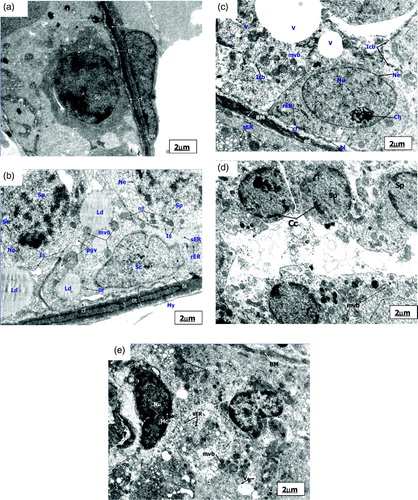
Figure 2. Electronmicrograph of control and pairfed groups after 4 weeks. The scale bar = 2 µm. (A) Electronmicrograph of testes of 4ZC group illustrating normal features of basement membrane (BM) with basal lamina (bl), collagen fibres (cf), and endothelial cells (ed). Cytoplasm shows characteristic peripheral arrangement of mitochondria (pmt) with dilated intra-cristal space, rough endoplasmic reticulum (rER), and inter-Sertoli cell junctional complexes (Is). Sertoli cell (Sc) and Leydig cell (Lc) are distinct. The nucleus of Leydig cells (Lc) reveals peripheral heterochromatin (Hc). (B) Electronmicrograph of testes of 4PF group showing basement membrane (BM) with basal lamina (bl), collagen fibres (cf), and endothelial cells (ed). Sertoli cell (Sc) with lobulated nucleus (Nu), mitochondria (mt), smooth endoplasmic reticulum (sER), lysosomes (Ly), phagocytic vacuoles (pgv), and mutivesicular bodies (mvb) seen in large number in the cytoplasm.
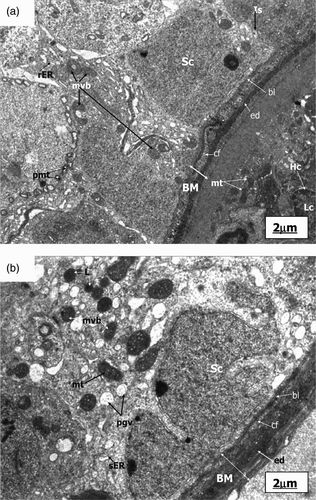
Figure 3. Electronmicrograph of zinc deficient groups after 4 weeks showing several apoptotic features. The scale bar = 2 µm (A, B, C, D, E, and G); The scale bar = 1 µm (F and H). (A) Electronmicrograph of testes of 4ZD group showing basement membrane (BM) with layer of basal lamina (bl), collagen fibers (cf) and cytoplasmic extensions of endothelial cells (ed). Degenerative changes are evident in the Sertoli cell (Sc) cytoplasm. Multivesicular bodies (mvb), vacuolization (v), and few mitochondria (mt) are distinct. (B) Electronmicrograph of 4ZD group testes showing disrupted spermatogenic epithelium (BM), dissolution of collagen fibres (cf) and displaced Type B spermatogonium nucleus (Nu) with condensed chromatin (Cc) associated with the nuclear envelope (Ne). Cytoplasm reveals vacuolization (V) with complete loss of junctional complexes. Lysosomes (Ly), deformed mitochondria (Amt), polyribosomes (pr), smooth endoplasmic reticulum (sER), and lipid droplets (Ld) are evident. (C) Ultrastructure of 4ZD group testes displaying primary spermatocyte nucleus (Nu) showing dissolution of nuclear envelope (Ne) and chromatin conglomerates (Ch). Cytoplasm reveals ovoid mitochondria (mt) and interrupted intercellular bridges (Icb). Cytoplasmic dissolution (CD) is also evident in some areas. (D) Electronmicrograph of testes of 4ZD group displaying detachment of acrosomal cap (ac) from head region of spermatid nucleus (Nu) just above the sub-acrosomal space (sas). One of the apoptotic spermatid (Asd) showing ring of condensed chromatin (Cc) around the nuclear periphery. Presence of mitochondria (mt) and lysosomes (Ly) are distinct. Degeneration of cytoplasm (CD) between the two spermatids is evident. (E) Electronmicrograph of testes of 4ZD group revealing deformed sperm heads (DS) with condensed nucleus (Nu), and disintegrated nuclear envelope (Ne). Cytoplasm shows few deformed mitochondria (Amt), multivesicular bodies (mvb), and dissolution of cytoplasm (CD) in some areas. (F) Cross sectional view of mid-piece (mp), principal piece (pp), and end piece (ep) of 4ZD spermatozoa tail revealing outer dense fibres (df) and central fibres (cf), mitochondrial sheath degeneration (mts) and retention of superfluous cytoplasm (sfc). (G) Aggregation of spermatozoa enclosed by a cytoplasm and common membrane (M). (H) Electronmicrograph of testes of 4ZD group displaying late apoptotic stage of Leydig cell nucleus (Nu) in which both chromatin (Cc) and cytoplasm have condensed. Peripheral heterochromatin (Hc) is distinct. Blebs (B) in the nuclear membrane of Leydig cell is also evident.
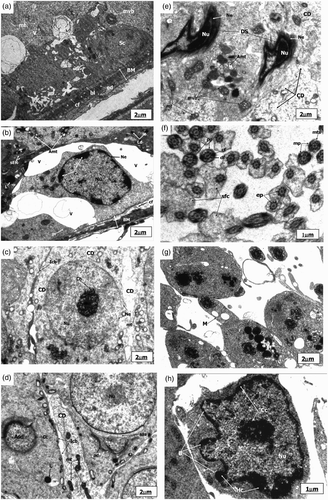
TUNEL Assay
The 2 and 4 w ZC control groups exhibited negative TUNEL staining in the majority of tubules although few tubules revealed TUNEL positive germ cells (A and 5A). The PF 2 and 4 w groups only showed positive staining in a few germ cells (B and 5B). An increase in the frequency of TUNEL positive cells was evident in the case of the 2 w ZD group as compared to the control group (13.85 ± 0.21 vs. 1.90 ± 0.08) (C; Table 1). As illustrated in C and , a higher apoptotic rate of germ cells were also observed in the case of the 4 w ZD group when compared with its respective control group (31.47 ± 0.20 vs. 2.62 ± 0.12).
Figure 4. Microphotograph of TUNEL staining after 2 weeks for control, pairfed, and zinc deficient groups. Note: 400X. (A) Microphotograph of testes of 2ZC group revealing negative TUNEL staining in all the germ cells. (B) Microphotograph of testes of 2PF group showing few TUNEL positive germ cells (arrows). (C) Microphotograph of testes of 2ZD group exhibiting few TUNEL positive apoptotic spermatogonia and primary spermatocytes (arrows).
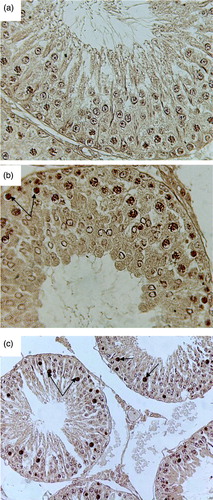
Figure 5. Microphotograph of TUNEL staining after 4 weeks for control, pairfed, and zinc deficient groups. Note: 400X. (A) Microphotograph of testes of 4ZC group exhibiting negative TUNEL staining in all the germ cells. (B) Microphotograph of testes of 4PF group showing few TUNEL positive cells (arrows). (C) Microphotograph of testes of 4ZD group revealing increase in the number of apoptotic cells. Positive staining seen in spermatogonia, primary spermatocytes, and giant cells (Gi) (cap-phase spermatids) (arrows).
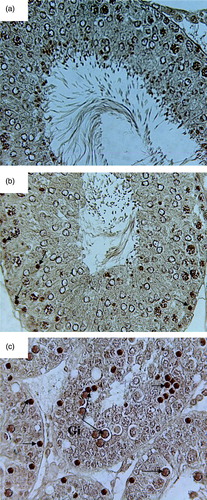
Table 1. Apoptotic Index (%) in testes of Wistar rats.
Epididymal Sperm Concentration, Sperm Motility, and Fertility Index
A significant (P < 0.05) decrease in epididymal sperm concentration, sperm motility, and fertility index were evident in zinc deficient groups after 2 and 4 w when compared with their respective control and PF groups. Parallel observations are summarized in Tables and when comparisons were made between ZC and PF and PF and ZD groups in both sets of experiments.
Table 2. Epididymal sperm concentration (x 106/ ml) and motility (%) of Wistar rats.
Table 3. Fertility Index of male Wistar rats.
Discussion
Morphological alterations of the nucleus, cell-membrane, cytosol, and mitochondria are apparent during apoptotic cell death. Ultrastructurally, apoptosis is characterized by cell volume shrinkage, chromatin condensation, blebbing of the nuclear envelope, fragmentation of the nucleus, cytoplasmic vacuolization, condensation of the cytoplasm, and disintegration of the cell into membrane bound remnants termed apoptotic bodies [Kerr et al. 1972; Widlak et al. 2002; Hassan et al. 2009]. Most organelles in the cytoplasm retain their normal appearance only during the early stages of apoptosis, but at later stages cytoplasmic organelles are no longer identifiable [Erkkilä et al. 1998]. The present study demonstrated severe atrophic changes which increased with the duration of zinc deficiency. Electron microscopy revealed several apoptotic features. The tunica propria of seminiferous tubules from control and PF groups were comprised of elongated endothelial cells surrounded by extracellular matrix (ECM) consisting of basal lamina and thin collagen fibres. The basement membrane of the 2 w ZD group was wavy with disrupted collagen fibres and peritubular myoid cells, a significant component of blood-testes barrier that ensures the structural cohesion and contraction of seminiferous tubules [Dym and Fawcett 1970]. After 4 w, the degeneration was more prominent. Disruption of lamina propria at several areas and dissolution of collagen fibres were also observed. Bahuguna and Bedwal [1998] also reported alterations in the microenvironment of seminiferous tubules owing to perturbations in the boundary layer. Dirami et al. [1995] reported that basement membrane plays a crucial role in Sertoli cell survival in vitro. Apoptosis is induced by disruption of the interactions between normal epithelial cells and ECM [Frisch and Francis 1994]. It is possible that the cooperation between Sertoli cells and peritubular myoid cells might have been disrupted in zinc deficient groups and as a result an abnormality in the synthesis of ECM could have occurred, leading to the disruption in the boundary tissues of the seminiferous tubules.
In the present study, spermatogonia of ZC and PF group showed normal structure. In comparison the deficient groups, presented dislocated spermatogonia with irregular cell boundary and dense chromatin material associated with the nuclear envelope. Cytoplasm showed vacuolization and loss of junctional complexes. Electron microscopic (EM) studies by others [Erkkilä et al. 1998; Pentikäinen et al. 1999] have revealed dense nuclear chromatin with numerous vacuoles in the cytoplasm during the early stages of apoptosis in spermatogonia.
The primary spermatocytes of control groups exhibited normal architecture. The 2 and 4 w PF groups also exhibited normal features, although degeneration was evident around the nucleus that could be due to low intake of essential nutrients as a result of a restricted food supply. In comparison, primary spermatocytes of the 2 w deficient group showed early apoptotic change i.e., chromatin condensation in crescent form on the periphery of nucleus with a few scattered clumps, a feature also reported by authors [Kerr et al. 1972; Erkkilä et al. 1997]. After 4 w of zinc deficiency, primary spermatocytes showed nuclear envelope disruption and chromatin conglomerates. The intercellular bridges were disrupted and the number of healthy mitochondria reduced. Intercellular bridges are necessary for sharing molecular signals between rat spermatids. They may function in a similar way in spermatogonia and primary spermatocytes [Ventelä et al. 2003]. The disruption of intercellular bridges in zinc deficiency is consistent with the alteration in pro-apoptotic signals in spermatogonia and primary spermatocytes. Erkkilä et al. [1998] also reported that during the later stages of primary spermatocyte apoptosis, the structure of nuclear envelope started to disperse and finally disappeared. The presence of chelatable zinc in pre-leptotene spermatocytes has been suggested to play an essential role at the onset of meiosis [Sorensen et al. 1998]. Zinc deficiency might affect spindle formation hence cell division through microtubules which are required for the formation of spindle fibres where zinc is one of the essential ions for polymerization [Hesketh 1984].
Ultrastructurally, spermatids of the 4 w ZC and PF groups presented an acrosomal complex with an intact outer- and inner-acrosomal membrane and homogenous acrosomal content. However, the 4 w ZD spermatids showed acrosomal deformities such as decapitation (i.e., detachment of the acrosomal cap from the head region) and a ring of condensed chromatin around the nuclear periphery. Their cytoplasm revealed loss of intercellular bridges, lysosomes, and deformed mitochondria. These features have been observed by others [Merker and Günther 1997; Erkkilä et al. 1998]. The final stages of spermatid differentiation, which include cytoplasmic modeling and the formation of the residual body [Russell 1979], were adversely affected by zinc-deprivation. Barney et al. [1968] also reported that the transformation of spermatids into spermatozoa is inhibited in zinc deficient rats. Dramatic biochemical and ultrastructural changes in nuclei occur as mammalian spermatids develop into spermatozoa. CREM (cyclic AMP response element modulator) knock-out mice are sterile due to arrest of spermatogenesis at the round spermatid stage [Nantel et al. 1996]. Infertility is due to lack of expression of key post-meiotic genes i.e., protamines 1 and 2, transition proteins TNP1 and TNP2, proacrosin, and calspermin [Sassone-Corsi 1998]. CREM activity in post meiotic-germ cells is enhanced by its association with LIM only protein, ACT (Activator of CREM) in the testis [Fimia et al. 1999; Palermo et al. 2001]. ACT is composed of four and a half LIM domains each containing two zinc finger motifs that facilitate protein-protein interactions [Fimia et al. 1999], thus suggesting a role of zinc in spermatogenesis. The present study displayed abnormalities in the condensation of nuclei of mature spermatids and spermatozoa which suggests that zinc deficiency might alter the transition process by causing changes in nucleoproteins of spermatozoa as it has been reported that zinc is also involved in histone displacement in the process of nuclear condensation during spermiogenesis [Hunt and Johnson 1990].
The cross sectional structural view of the mid piece of the spermatozoa tails of the 4 w ZC and PF groups appears normal although the degeneration of the mitochondrial sheath with blebs in the membrane of the principal piece and intermediate filaments were observed in 4 w PF groups. The mitochondrial sheath of mid piece and fibrous sheath of tail regions of spermatozoa of the 4 w ZD groups showed degeneration along with superfluous cytoplasm. This group also showed deformed sperm heads with condensed nuclei, a disintegrated nuclear envelope, cytoplasmic dissolution, and aggregation of 5–10 spermatozoa enclosed by cytoplasm and a common membrane. These structures presented a process involved in the separation of spermatids into individual spermatozoa that might have also been affected by zinc deficiency and hence might increase the frequency of abnormal, double, triple, or even more spermatozoa with defective structures in the ejaculate. Parallel were the findings of Dinsdale and Williams [1980], Wallace et al. [1984], Bedwal and Bahuguna [1994], Merker and Günther [1997], and Merrells et al. [2009]. Zinc is known to influence the motility, viability, and morphology of spermatozoa all of which are vital for normal sperm physiology [Ebisch et al. 2007; Merrells et al. 2009]. Omu et al. [2008] reported that zinc therapy reduces asthenozoospermia through several mechanisms preventing oxidative stress, apoptosis, and sperm DNA fragmentation. In the sperm nucleus, zinc contributes to stability of the quarternary structure of chromatin and preserves genomic integrity [Wallace et al. 1984; Kvist et al. 1988; Bedwal and Bahuguna 1994; Ho et al. 2003; Song et al. 2009; Björndahl and Kvist 2010]; a model of which has been proposed [Björndahl and Kvist 2011]. Production of reactive oxygen species (ROS) by spermatozoa is a normal physiological process [Ebisch et al. 2007; Shamsi et al. 2008] but high levels of ROS are potentially toxic to sperm quality and function [Saleh and Agarwal 2002]. Mammalian spermatozoa are rich in polyunsaturated fatty acids (PUFA) which are vulnerable to attack by ROS and membrane lipid peroxide ion mediated by lipid peroxidation [Dandekar et al. 2002; Zalata et al. 2004]. Previous reports from our laboratory showed an increased lipid peroxidation (TBARS) concentration in testes, and caput and cauda epididymis of zinc deficient Wistar rats [Nair et al. 2005]. Lipid peroxidation of PUFA molecules of plasma membrane of spermatozoa is largely responsible for defective spermatozoa and male infertility [Aitken et al. 1993]. Lipid peroxidation causes leakiness of sperm plasma membrane which in turn losses its fluidity and integrity [Aitken et al. 1993], damaging the mid-piece and axonemal complex leading to decreased concentration and loss of motility as observed in the present study, since disruption of the testicular homeostatic mechanism influences considerably the epididymal reserves and decreases capacitation [Tramer et al. 1998; Dandekar et al. 2002]. Zinc deficiency adversely affects sperm integrity and modulates testes fatty acid composition by interrupting essential fatty acid metabolism thereby affecting overall testicular function [Merrells et al. 2009]. Ultrastructural observations of the present study exhibited several sperm defects such as tail-elements with superfluous cytoplasm and degeneration in mitochondrial sheath which further supported that zinc deficiency affects sperm motility. Henkel et al. [1999] reported the importance of zinc during epididymal sperm maturation for functional competence of the outer dense fibers and, therefore, motility. A low content of nuclear zinc would impair the structural stability of chromatin and thereby increase the vulnerability of the male genome [Kvist et al. 1988]. This may be one of the reasons for affecting the fertilizing capacity of spermatozoa. This is further supported by a decrease in fertility index in the 2 and 4 w ZD groups as observed in the present study by cohabiting the zinc deficient males with proven females.
Leydig cell nuclei of the 2 and 4 w ZC and PF groups were more or less elongated with heterochromatin masses present towards the periphery. The 2 w ZD groups displayed degeneration of cytoplasm along with the remnants of cell organelles, dilated smooth endoplasmic reticulum (sER), lipid droplets, vacuolization, and abundant multivesicular bodies (MVBs). Further, the degeneration of Leydig cell was more prominent after 4 w of zinc deficiency as evident by condensation of both cytoplasm and chromatin with larger heterochromatin areas. Hesketh [1982] reported that Leydig cells from zinc-deficient animals were smaller with abnormal endoplasmic reticulum.
The highly coordinated nature of spermatogenesis requires intimate functional and junctional communication between Sertoli and germ cells [Sluka et al. 2006]. In the present study, the ovoid nucleus of Sertoli cells in the 2 and 4 w ZC and PF groups was characterized by a deep indentation with a well-defined dense nucleolus. The Sertoli cells of the 2 w ZD group revealed normal structural features, whereas the 4 w ZD group nucleus exhibited small indentations with loss of specialized intercellular junctions. The degenerative changes of Sertoli cells accounts for the increased apoptotic cell number which may be due to loss of its phagocytic activity. Detachment of epithelial cells from the matrix [Dirami et al. 1995] and the absence of basement membrane for Sertoli cells [Frisch and Francis 1994] results in apoptosis. The present study emphasized that altered Sertoli cell structures i.e., appearance of vacuoles due to cytoplasmic degeneration and morphological disruption of Sertoli cell junctional specializations might be the major cause of extensive testes deterioration. This supports the notion that Sertoli cells are sensitive to zinc deficiency that mediates the disruption of spermatogenic epithelium. These alterations may be the main cause of germ cell loss by apoptosis and tubular atrophy in zinc deficient rats.
The major hallmark of apoptosis in the nucleus is the internucleosomal fragmentation of double stranded DNA into fragments of 180 to 200 base pair length [Saraste and Pulkki 2000]. During apoptosis, caspase-3 cleaves the inhibitor allowing the nuclease to cut the chromatin [Rao et al. 1996]. Using terminal deoxynucleotidyl transferase (TdT) dUTP nick end labelling (TUNEL) method, degenerating cells could probably be identified as primary spermatocytes and round spermatids located in the adluminal compartment. Ultrastructural studies also confirmed degenerative changes in primary spermatocytes and round spermatids. TUNEL positive stained cells were equivalent to the observations of ultrastructural studies enabling the determination of cells undergoing apoptosis. Rare apoptotic spermatogonia displaced from the basal lamina were evident in ultrastructural studies. Further, both in the 2 and 4 w ZD groups primary spermatocytes and cap phase spermatids were prominently affected. Li et al. [1998] observed a significant (P < 0.01) increase in apoptotic number of spermatogenic cells after zinc deficiency. Nodera et al. [2001] also reported the appearance of TUNEL positive cells after 3 w of zinc deficiency in testes and thymus of 7 w old Sprague Dawley rats. Abnormally accelerated apoptosis of germ cells may lead to an imbalance of cell proliferation and death resulting in spermatogenic impairment [Takagi et al. 2001; Kimura et al. 2003]. The correct balance between the apoptotic pathways is important for the maintenance of genomic integrity while preventing unnecessary cell death [Clarke and Allan 2009]. In the present study, apoptotic frequency was more pronounced after 4 w of the experiment, hence it can be concluded that prolonged zinc deficiency enhances apoptosis of testicular cells.
Increased apoptotic degeneration in testes may cause irreversible changes in the germ cells associated with decreased epididymal sperm concentration, motility, and fertility index. Together, the above findings are indicative that the onset of apoptosis observed in the testes can be induced by dietary zinc deficiency, which contributes to the low efficiency of spermatogenesis thereby indicating a possible role of zinc in fertility.
Materials and Methods
The composition of basal experimental diet (per Kg) was prepared as follows: egg albumin - 180 g, corn oil – 100 g, corn starch – 443g, sucrose – 200 g, cellulose – 30 g, choline chloride – 2g, AIN-76 salt mixture – 35g; AIN-76C vitamin-antibiotic mixture – 10 g; DL-methionine – 7g; D-Biotin - 20 mg. Zinc contents of basal diets from each lot was estimated at 213.9 nm in air-acetylene flame on GBC 902 Atomic Absorption Spectrophotometer (AAS) and zinc concentrations were adjusted to 1.0 ppm and 100 ppm by addition of appropriate amounts of zinc sulphate. Sixty colony bred male pre-pubertal Wistar rats (30 – 40 d; 40–50 gm) were divided into three groups (each comprised of 10 animals in a 2 and 4 w sub group: control, pairfed, and zinc deficient): (i) ZC (100ppm zinc diet), (ii) PF (100ppm zinc diet but the amount of feed was equal to the (average) amount consumed by the zinc deficient group the previous day, this group was run so as to study starvation effects caused by reduced intake of diet and stress effects of synthetic diet), and (iii) ZD (1.00ppm zinc diet).
The animals were housed individually in polypropylene cages with stainless steel grills. The polypropylene cages, grills, and bottles were washed with detergent solution, demineralized water and finally were rinsed in 10% EDTA so as to avoid any contamination and subsequent removal of zinc. The experiments were set for 2 and 4 w and were approved by the Departmental Ethics Committee, Department of Zoology, University of Rajasthan, Jaipur, India. Animals were anaesthetized under light ether anesthesia and testes were excised, cleaned off of extraneous tissues, and processed.
Ultrastructural Studies
Testes were removed and immersed quickly in Karnovsky's fixative, cut into small pieces under a drop of fixative and again kept in fresh fixative for 4 h. After 4 h, tissues were washed frequently in cacodylate buffer (pH – 7.2) and kept overnight at 4°C. The pieces were osmicated with Caulfield's fixative for 2 h at 4°C and then washed in cold TDW (giving 4–5 changes) and brought to room temperature. They were then dehydrated in ethanol series, cleared in dry acetone, and embedded in araldite CY 212. Semithin sections were cut, stained with toludine blue, and observed under a light microscope. Ultrathin sections (30 - 40nm) were stained with lead citrate and uranyl acetate. Twenty loci of testicular tissue of different groups were sampled and 10 were taken into account. Observations and micrographs were taken on a Transmission Electron Microscope (Morgagni 268D, FEI Company, The Netherlands; All India Institute of Medical Sciences, New Delhi).
TUNEL
In situ detection (TA 4625, R and D Systems, Inc, Minneapolis, USA) was used to identify apoptotic cells by detecting DNA fragmentation through a combination of enzymology and immunohistochemistry techniques. Bouin's fixed (5 µm) sections of testes were used. In brief, after deparaffinization and rehydration, tissue sections were incubated with proteinase K, washed in distilled water, and then incubated in phosphate buffered saline solution (PBS) containing 3% hydrogen peroxide for 10 min to quench endogenous peroxidase activity. Next, biotinylated nucleotides were detected by using streptavidin-horseradish peroxidase conjugate followed by the substrate diaminobenzidine (DAB). The enzyme reaction generates an insoluble coloured precipitate where DNA fragmentation has occurred.
Quantification of apoptotic cells
Nine testes from each experimental group were used for the study. The apoptotic index was calculated by dividing the number of TUNEL positive germ cells per seminiferous tubule by the total number of germ cells per seminiferous tubule and the result was multiplied by 100. Mean AI of each case was calculated. At least 20 apoptotic tubule cross sections (TCS) within 200 TCS in each testis were taken into account.
Epididymal sperm concentration and sperm motility
Epididymal sperm concentration and motility were assessed by the method of Linder et al. [1986]. The epididymal sperm were collected by cutting the epididymis into small pieces and flushing the sperm in normal saline. The sperm collected was centrifuged at 225 × g for 10 min. The pellet was resuspended in 2.0 ml of normal saline. An aliquot of this solution was placed in a Neubauer hemocytometer and sperm counts, motile and non-motile sperms were counted. Sperm motility was expressed as a percent of motile sperm of the total sperm counted.
Fertility index
Ten males from each experimental group were reared until adulthood on standard rat feed (Aashirwad Ltd., Chandigarh, India) and tap water ad libitum and assessed for mating and fertility. Each male was placed with an adult female rat in the ratio of 1:2. Females were checked for plugs and if found were caged separately till offspring were born and counted. If a plug was not found after 7 d, the male was scored as not having mated and was removed from the cage, the female was monitored to confirm the pregnancy.
Statistical analysis
Data were expressed as mean ± SEM. Further analysis of 2 and 4 w treatment groups was carried out separately using One way Analysis of Variance (ANOVA) and if the difference was found to be significant then post-hoc test (Duncan's Multiple Comparison Test) was applied. P < 0.05 was considered to be significant.
Abbreviations
ZC: | = | zinc control |
PF: | = | pairfed |
ZD: | = | zinc deficient |
LH: | = | luteinizing hormone |
ER: | = | endoplasmic reticulum |
FSH | = | follicle-stimulating hormone |
ECM: | = | extracellular matrix |
CREM: | = | cyclic AMP response element modulator |
ACT: | = | activator of CREM |
ROS: | = | reactive oxygen species |
PUFA: | = | polyunsaturated fatty acids |
TUNEL: | = | terminal deoxynucleotidyl transferase [TdT] dUTP nick endlabelling |
MVBs: | = | multivesicular bodies. |
Acknowledgments
Author Ms. Deepa Kumari, thanks the Department of Science and Technology (DST), Government of Rajasthan, Jaipur [F [5/2] vipro/ DST/ R and D/07-1937] for providing financial assistance, the Department of Zoology, University of Rajasthan, Jaipur [F-41/08/DRS-81/91] for providing Departmental Research Fellowship and the University Grants Commission (UGC), New Delhi [F.4-3/2006 [BSR] 5- 96/2007[BSR] for providing Meritorious Research Fellowship.
Declaration of Interest: The authors report no conflicts of interest. The authors alone are responsible for the content and writing of the paper.
References
- Adamo, A.M., Oteiza and P.I. (2010) Zinc deficiency and neurodevelopment: The case of neurons. Biofactors 36:117–124.
- Aitken, R.J., Harkiss, D. and Buckingham, D.W. (1993) Analysis of lipid peroxidation mechanisms in human spermatozoa. Mol Reprod Dev 35:302–315.
- Ali, H., Ahmed, M., Baig, M. and Ali, M. (2007) Relationship of zinc concentrations in blood and seminal plasma with various semen parameters in infertile subjects. Pak J Med Sci 23:111–114.
- Al-Timimi, D.J. (2009) Marginal zinc deficiency: A significant but unrecognized public health problem in Iraq. Duhok Med J 3:1–3.
- Auld, D.S. (2009) The ins and outs of biological zinc sites. Biometals 22:141–148.
- Bahuguna, A. and Bedwal, R.S. (1998) Effects of zinc deficiency on boundary layers of seminiferous tubules of testes of Wistar rats. Curr Sci 75:611–616.
- Barney, G.H., Orgebin-Crist, M.C. and Macapinalac, M.P. (1968) Genesis of esophageal parakeratosis and histologic changes in the testes of the zinc-deficient rat and their reversal by zinc repletion. J Nutr 95:526–534.
- Bedwal, R.S., Nair, N. and Mathur, R.S. (1991) Effects of zinc deficiency and toxicity on reproductive organs, pregnancy and lactation - A review. Trace Elem Med 8:89–100.
- Bedwal, R.S. and Bahuguna, A. (1994) Zinc, Copper and Selenium in reproduction. Experientia 50:626–640.
- Billig, H., Furuta, I., Rivier, C., Tapanainen, J., Parvinen, M. and Hsueh, A.J. (1995) Apoptosis in testis germ cells: Developmental changes in gonadotropin dependence and localization to selective tubule stages. Endocrinology 136:5–12.
- Björndahl, L. and Kvist, U. (2010) Human sperm chromatin stabilization: A proposed model including zinc bridges. Mol Human Reprod 16:23–29.
- Björndahl, L. and Kvist, U. (2011) A model for the importance of zinc in the dynamics of human sperm chromatin stabilization after ejaculation in relation to sperm DNA vulnerability. Syst Biol Reprod Med 57(1–2):86–92. http://www.ncbi.nlm.nih.gov/pubmed/21204594
- Chai, F., Truong-Tran, A.Q., Ho, L.H. and Zalewski, P.D. (1999) Regulation of caspase activation and apoptosis by cellular zinc fluxes and zinc deprivation: A review. Immunol Cell Biol 77:272–278.
- Clarke, P.R. and Allan, L.A. (2009) Cell-cycle control in the face of damage - A matter of life and death. Trends Cell Biol 579:1–10.
- Dandekar, S.P, Nadkarni, G.D, Kulkarni, V.S., Pnekar, S. (2002) Lipid peroxidation and antioxidant enzymes in male infertility. J Postgrad Med 48:186–189.
- Dinsdale, D. and Williams, R.B. (1980) Ultrastructural changes in the sperm tail of zinc deficient rats. J Comp Pathol 90:559–566.
- Dirami, G., Ravindranath, N., Kleinman, H.K. and Dym, M. (1995) Evidence that basement membrane prevents apoptosis of Sertoli cells in vitro in the absence of known regulators of Sertoli function. Endocrinology 135:4439–4447.
- Dissanayake, D.M.A.B., Wijesinghe, P.S., Ratnasooriya, W.D., Wimalasena, S. (2009) Effects of zinc supplementation on sexual behavior of male rats. J Human Reprod Sci 2:57–61.
- Duffy, J.Y., Miller, C.M., Rutschilling, G.L., Ridder, G.M., Clegg, M.S., Keen, C.L. and Daston, G.P. (2001) A decrease in intracellular zinc level precedes the detection of early indicators of apoptosis in HL-60 cells. Apoptosis 6:161–172.
- Dym, M. and Fawcett, D.W. (1970) The blood-testis barrier in the rat and the physiological compartmentation of the seminiferous epithelium. Biol Reprod 3:308–326.
- Ebisch, I.M.W., Thomas, C.M.G., Peters, W.H.M., Bract, D.D.M. Steegers-Theunissen, R.P.M. (2007) The importance of folate, zinc and antioxidants in the pathogenesis and prevention of sub-fertility. Human Reprod Update 13:163–174.
- Erkkilä, K., Henriksen, K., Hirvonen, V., Rannikko, S., Salo, J., Parvinen, M. and Dunkel, L. (1997) Testosterone regulates apoptosis in adult human seminiferous tubules in vitro. J Clin Endocrinol Metab 82:2314–2321.
- Erkkilä, K., Hirvonen, V., Wuokko, E., Parvinen, M. and Dunkel, L. (1998) N-Acetyl-L-Cysteine inhibits apoptosis in human male germ cells in vitro. J Clin Endocrinol Metab 83:2523–2531.
- Fanzo, J.C., Reaves, S.K., Cui, L., Zhu, L., Wu, J.J.Y., Wang, Y.R. and Lei, K.Y. (2001) Zinc status affects pp53, gadd45, and c-fos expression and caspase-3 activity in human bronchial epithelial cells. Am J Physiol Cell Physiol 281:C751–C757.
- Fesharakinia, A., Zarban, A. and Sharifzadeh, G.R. (2009) Prevalence of zinc deficiency in elementary school children of South Khorasan province East Iran. Iran J Pediatr 19:249–254.
- Fimia, G.M., De Cesau, D., Sassone-Corsi, P. (1999) CBP-independent activation of CREM and CREB by the LIM only protein ACT. Nature 398:165–169.
- Fraker, P.J. (2005) Roles for cell death in zinc deficiency. J Nutr 135:359–362.
- Frisch, S.M. and Francis, H. (1994) Disruption of epithelial cell-matrix interactions induces apoptosis. J Cell Biol 124:619–626.
- Fukamachi, Y., Karaski, Y., Sugiura, T., Itoh, H., Abe, T., Yamamura, K. and Higashi, K. (1998) Zinc suppresses apoptosis of U937 cells induced by Bcl2/ Bax ratio. Biochem Biophys Res Comm 246:364–369.
- Giannakis, C., Forbes, I.J. and Zalewski, P.D. (1991) Ca2+/Mg2+ dependent nuclease, tissue distribution, relationship to inter-nucleosomal DNA fragmentation and inhibition by Zn2+. Biochem Biophys Res Commun 181:915–920.
- Hassan, A., El-Nashar, E.M. and Mostafa, I. (2009) Programmed cell death in varicocele-bearing testes. Andrologia 41:39–45.
- Henkel, R., Bittner, J., Weber, R., Hüther, F., Miska, W. (1999) Relevance of zinc in human sperm flagella and its relation to motility. Fertil Steril 71:1138–1143.
- Henriksen, K., Hakovirta, H. and Parvinen, M. (1995) In situ quantification of stage-specific apoptosis in the rat seminiferous epithelium: Effects of short-term experimental cryptorchidism. Internat J Androl 17:394–402.
- Hesketh, J.E. (1982) Zinc stimulated microtubule assembly and evidence for zinc binding to tubulin. Internat J Biochem 14:983–990.
- Hesketh, J.E. (1984) Microtubule assembly in rat brain extracts: Further characterization of the effects of zinc on assembly and cold stability. Internat J Biochem 16:1331–1339.
- Hess, S.Y. and King, J.C. (2009) Effects of maternal zinc supplementation on pregnancy and lactation outcomes. Food Nutr Bull 30:S60–S78.
- Ho, E., Ames, B.N. (2002) Low intracellular zinc induces oxidative DNA damage, disrupts pp53, NFκB and AP1 DNA binding, and affects DNA repair in a rat glioma cell line. Proc Natl Acad Sci USA 99:16770–16775.
- Ho, E., Courtemanche, C. and Ames, B.N. (2003) Zinc deficiency induces oxidative DNA damage and increases pp53 expression in human lung fibroblasts. J Nutr 133:2543–2548.
- Hunt, C.D. and Johnson, P.E. (1990) The effects of dietary zinc on human sperm morphology and seminal mineral loss. In: Trace Element Metabolism in Man and Animals-7. eds Hurley L.S., Keen, C.L., Lonnerdal, B. Rucker, R.B. Plenum Press, New York pp35–42.
- Jankowski-Hennig, M.A., Clegg, M.S., Daston, G.P., Rogers, J.M., Keen, C.L. (2000) Zinc-deficient rat embryos have increased caspase 3–like activity and apoptosis. Biochem Biophys Res Commun 271:250–256.
- Kaji, M. (2001) Zinc in endocrinology. Internat Pediatr 16:1–7.
- Kerr, J.F.R., Wyllie, A.H. and Currie, A.R. (1972) Apoptosis: Basic biological phenomenon with wide range of implications in tissue kinetics. British J Canc 26:239–257.
- Kimura, M., Itoh, N., Takagi, S., Sasao, T., Takahashi, A., Masumori, N. and Tsukamoto, T. (2003) Balance of apoptosis and proliferation of germ cells related to spermatogenesis in aged men. J Androl 24:185–191.
- Kvist, U., Kjelberg, S., Björndahl, L., Hammar, M., Roomans, G. (1988) Zinc in sperm chromatin and chromatin stability in fertile men and men in barren unions. Scand. J Urol Nephrol 22:1–6.
- Li, J., Xu, P. and He, Z. (1998) Effect of zinc deficiency on apoptosis of spermatogenic cell of rat testis. Zhonghua Yi Xue Za Zhi 78:91–103.
- Linder, R.E., Strader, L.F. and McElroy, W.K. (1986) Measurement of epididymal sperm motility as a test variable in the rat. Bull Env Contamin Toxicol 36:317–324.
- Maret, W. (2009) Molecular aspects of human cellular zinc homeostasis: Redox control of zinc potentials and zinc signals. Biometals 22:149–157.
- Maret, W. and Sandstead, H. (2008) Possible roles of zinc nutriture in the fetal origins of disease. Exptl Gerontology 43:378–381.
- Merker, H.J. and Günther, T. (1997) Testis damage induced by zinc deficiency in rats. pJ Trace Elem Med Biol 11:19–22.
- Merrells, K.J., Blewett, H., Jaamieson, J.A., Taylor, C.G. and Suh, M. (2009) Relationship between abnormal sperm physiology induced by dietary zinc deficiency and lipid composition in testes of growing rats. British J Nutr 102:226–232.
- Nair, N., Bedwal, R.S., Prasad, S., Saini,.M.R., Bedwal, R.S. (2005) Short term zinc deficiency in diet induces increased oxidative stress in testes and epididymis of rats.. Indian J Expt Biol 43:786–794.
- Nantel, F., Monaco, L., Foulkes, N.S., Masquilier, D., LeMeur, M., Henriksen, K., (1996) Spermiogenesis deficiency and germ cell apoptosis in CREM-mutant mice. Nature 380:159–162.
- Nodera, M., Yanagisawa, H., Wada, O. (2001) Increased apoptosis in a variety of tissues of zinc deficient rats. Life Sci 14:1639–1649.
- Omu, A.E., Al-Azemi, M.K., Kehinde, E.O., Anim, J.T, Oriowa, M.A., Mathew, T.C. (2008) Indications of the mechanisms involved in improved sperm parameters by zinc therapy. Med Prin Prac 17:108–116.
- Palermo, I., Litrico, L., Emmanuele, G., Giuffrida, V., Sassone-Corsi, P., De Cesare, D., (2001) Cloning and expression of activator of CREM in testis in human testicular tissue. Biochem Biophys Res Commun 283:406–411.
- Pentikäinen, V., Erkkilä, K., Dunkel, L. (1999) Fas regulate germ cell apoptosis in the human testis in vitro. Am J Physiol 276: E310–E316.
- Rao, L., Perez, D. and White, E. (1996) Lamin proteolysis facilitates nuclear events during apoptosis. J Cell Biol 135:1441–1455.
- Russell, L.D. (1979) Spermatid Sertoli tubulobulbar complexes as devices for elimination of cytoplasm from the head region of late spermatids of the rat. Anat Rec 194:233–246.
- Saleh, R. and Agarwal, A. (2002) Oxidative stress and male infertility: From research bench to clinical practice. J Androl 23:737–752.
- Saraste, A., Pulkki, K. (2000) Morphological and biochemical hallmarks of apoptosis. Cardiovasc Res 45:528–537.
- Sassone-Corsi, P. (1998) CREM: a master-switch governing male germ cell differentiation and apoptosis. Sem Dev Biol 9:475–482.
- Saxena, R., Bedwal, R., Mathur, R.S. (1993) Histopathology of epididymis and prostate of zinc deficient Sprague Dawley rats. Trace Elem Med 10:55–59.
- Shamsi, M.B., Kumar, R. and Dada, R. (2008) Evaluation of nuclear DNA damage in human spermatozoa in men opting for assisted reproduction. Indian J Med Res 127:115–123.
- Sluka, P., O'Donnell, L., Bartles, J.R., Stanton and P.G. (2006) FSH regulates the formation of adherens junctions and ectoplasmic specialisations between rat Sertoli cells in vitro and in vivo. J Endocrinol 189:381–395.
- Song, Y., Leonard, S.W., Bruno, R.S., Traber, M.G. and Ho, E. (2009) Zinc deficiency affects DNA damage, oxidative stress, antioxidant defenses, and DNA repair in rats. J Nutr 139:1626–1631.
- Sorensen, M.B., Stoltenberg, M., Henriksen, K., Ernst, E., Dansher, G. and Parvinen, M. (1998) Histochemical tracing of zinc ions in the rat testis. Mol Human Rep 4:423–428.
- Stennicke, H.R., Salvesen, G.S. (1997) Biochemical characteristics of caspase-3, -6, -7 and -8. J Biol Chem 272:25719–25723.
- Takagi, S., Itoh, N., Kimura, M., Sasao, T. and Tsukamato, T. (2001) Spermatogonial proliferation and apoptosis in hypospermatogenesis associated with non-obstructive azoospermia. Fertil Steril 76:901–907.
- Takahashi, A., Alnemri, E.S., Lazebnik, Y.A., Fernandes-Alnemri, T., Litwack, G., Moir, R.D., (1996) Cleavage of lamin A by mch 2α but not CPP32: multiple interleukin 1β-converting enzymes related proteases with distinct substrate recognition properties are active in apoptosis. Proc Natl Acad Sci USA 93:8395–8400.
- Tramer, F., Rocco, F., Micali, F., Sandri, G. and Panfili, E. (1998) Antioxidant systems in rat epididymal spermatozoa. Biol Reprod 59:753–758
- Tuerk, M.J. and Fazel, N. (2009) Zinc deficiency. Curr Opin Gastroenterol 25:136–143
- Uriu-Adams, J.Y. and Keen, C.L. (2010) Zinc and reproduction: Effects of zinc deficiency on prenatal and early postnatal development. Birth Defects Res 89:313–325.
- Ventelä, S., Toppari, J. and Parvinen, M. (2003) Intercellular organelle traffic through cytoplasmic bridges in early spermatids of the rat: Mechanisms of haploid gene product sharing. Mol Biol Cell 14:2768–2780.
- Walingo, M.K. (2009) Indigenous food processing methods that improve zinc absorption and bioavailability of plant diets consumed by the Kenyan population. African J Food Agri Nut Dev 9:524–535.
- Wallace, E., Calvin, H.I., Salgo, M.P., Dennis, D.E. and Ploetz, K. (1984) Normal levels of zinc and sulphydryls in morphologically abnormal populations of spermatozoa from moderately zinc deficient rats. Gamete Research 9:375–386.
- Widlak, P., Palyvoda, O., Kumala, S. and Gerrard, W.T. (2002) Modeling apoptotic chromatin condensation in normal cell nuclei. J Biol Chem 277:21683–21690.
- Wolf, C.M., Morana, S.J. and Eastman, A. (1997) Zinc inhibits apoptosis upstream of ICE/CED-3 protease rather than at the level of an endonuclease. Cell Death Differ. 4:125–129
- Yan, M., Song, Y., Wong, C.P., Hardin, K. and Ho, E. (2008) Zinc deficiency alters DNA damage response genes in normal human prostate epithelial cells. J Nutr 138:667–673.
- Zalata, A.A., Ahmed, A.H., Allamaneni, S.S., Comhaire, F.H. and Agarwal, A. (2004) Relationship between acrosin activity of human spermatozoa and oxidative stress. Asian J Androl 6:313–318.
- Zalewski, P., Forbes, I., Giannakis, C. and Betts, W. (1991) Regulation of protein kinase C by Zn (2+)-dependent interaction with actin. Biochem Int 24:1103–1110.
- Zhivotovsky, B., Wade, D., Gahm, A., Orrenius, S. and Nicotera, P. (1994) Formation of 50 kbp chromatin fragments in isolated liver nuclei is mediated by protease and endonuclease activation. FEBS Lett 351:150–154.