Pre-eclampsia continues to be the leading cause of maternal and fetal morbidity and mortality in the Western world. It is a pregnancy-specific disorder that occurs almost exclusively in humans [Palmer et al. Citation1979]. The latter observation has focused studies on the etiology of pre-eclampsia toward the rather unusual, highly invasive, hemochorial human placenta. To date, a single unifying hypothesis explaining pre-eclampsia remains elusive, likely because the disease may represent a downstream manifestation of several maternal and/or fetal factors, many of which result in inadequate placentation and abnormal placental perfusion. The disease appears to manifest in two major forms. Early onset disease is typically more severe and appears to be more closely linked to intrauterine growth retardation and abnormal placentation. Late onset disease is milder and may reflect maternal hypersensitivity to several metabolic changes that normally occur during pregnancy [Burton Citation2009].
Several authors have argued that oxidative stress is central to the pathogenesis of pre-eclampsia, particularly early-onset disease [Burton Citation2009; Kudo et al. Citation2003; Redman and Sargent Citation2009; Roberts and Hubel Citation2009]. Much of this hypothesis is based on a series of elegant experiments by Burton and Jauniaux [2004] showing that the human fetus typically develops in an environment that is quite hypoxic. In fact, maternal blood flow into the human placental intervillous space does not appear to start until approximately 9-10 weeks of gestation and is not fully established until 11-14 weeks of gestation [Burton and Jauniaux Citation2004]. Prior to that, the maternal spiral arteries supplying the fetus are plugged by endovascular trophoblast cells [Jauniaux et al. Citation1992; Meekins et al. Citation1997]. These invasive trophoblast cells will ultimately replace many of the cells within the walls of the maternal spiral arteries feeding the intervillous space. Such remodeling makes these arteries resistant to maternal vasoactive substances and ensures delivery of maternal blood to the fetus despite changes in maternal blood volume/flow. Remodeling is most extensive in the central placenta, with decreased invasion near the placental margins [Burton and Jauniaux Citation2004; Steegers et al. Citation2010]. Proponents of the placental oxidative stress model for pre-eclampsia posit that poor trophoblast invasion and remodeling of the maternal spiral arteries may lead to premature exposure of the fetus to maternal blood and premature elevation of oxygen and oxygen radical exposure for placental villi [Burton Citation2009; Burton and Jauniaux Citation2004; Jauniaux et al. Citation2000; Steegers et al. Citation2010]. Still others hypothesize that oxygen sensing is impaired in pre-eclamptic placentas. That is, pre-eclamptic placentas fail to sense higher oxygen levels, fail to properly react to elevations in oxygen and oxygen radicals and sustain oxidative damage [Rajakumar et al. Citation2003, Rolfo et al. Citation2010]. Regardless of the underlying pathophysiology, when most severe, these aberrations could lead to spontaneous pregnancy loss. When less severe, they could lead to abnormally shallow placentation. Further, shallow or incomplete maternal spiral artery remodeling could result in maternal spiral arteries that can continue to respond to maternal vasoactive substances, allowing for periods of unwanted hypoxia and re-oxygenation within the placenta. Reductions in organ blood flow that are followed by reperfusion are a common source of reactive oxygen species [Lum and Roebuck Citation2001].
In this issue of Systems Biology in Reproductive Medicine, Nishizawa et. al. [2011] expand on their prior work that demonstrates a reduction in indoleamine 2,3-dioxygenase (IDO) activity in the placentas of severe pre-eclamptic patients [Nishizawa et al. Citation2007; Nishizawa et al. Citation2010]. The authors have previously related this finding to abnormalities in maternal tolerance of the implanting fetus [Nishizawa et al. Citation2007, Nishizawa et al. Citation2010]. Here, they report three additional significant findings: 1) IDO co-localizes with the marker for oxidative DNA damage, 8-hydroxy-2'-deoxy-guanosine (8-OHdG), in placental endothelial and syncytiotrophoblast cells, 2) 8-OHdG levels in pre-eclamptic placentas are higher than those in normotensive patients, and 3) placental IDO activity and 8-OHdG levels are inversely correlated. In their present study, IDO was localized to the cell cytoplasm and 8-OHdG was localized to the nuclei of the same cell types. The authors conclude that oxidative stress in pre-eclamptic placentas is associated with decreased IDO activity and hypothesize an anti-oxidant role for IDO in the pathophysiology of pre-eclampsia.
This hypothesis represents a fairly fresh look at the role of tryptophan and IDO in pregnancy. This relationship was first addressed in the seminal work of Munn et al. [1998], who demonstrated that IDO blockade by 1-metyl-tryptophan results in the rejection of allogeneic, but not syngeneic murine fetuses and that maternal T cells are involved in this rejection. These findings are consistent with a great deal of literature generated before and after their work that placed tryptophan and its metabolites as important regulators of immune function. Tryptophan is the least abundant of the essential amino acids, so regulation of its production and breakdown would represent an efficient mechanism for controlling physiologic processes. Interferon gamma (IFNγ) enhances IDO activity [Werner-Felmayer et al. 1989], depleting tryptophan stores and increasing the production of tryptophan metabolites (). Tryptophan starvation and the production of kyneurenine and its metabolites decreases T cell proliferation and induces activated T cell apoptosis [Lee et al. Citation2002; Terness et al. Citation2002]. Tryptophan deprivation inhibits virus, bacteria, parasite, and intracellular pathogen growth [Thomas et al. Citation1993; Thomas and Stocker Citation1999]. Growth of tumor cells is inhibited by IDO induction in vitro [Ozaki et al. Citation1988]. Interestingly, and somewhat surprisingly, the reproductive phenotype in IDO knock-out mice is normal [Baban et al. Citation2004].
Figure 1. Tryptophan metabolism through the kynurenine pathway. Tryptophan is the least abundant of the essential amino acids brought into the body as dietary proteins or through dietary supplements. Tryptophan can be converted into the neurotransmitter serotonin or degraded through a variety of pathways. Degradation of tryptophan thru the kynurenine pathway is most common and begins with conversion of tryptophan to N-formyl kynurenine, the rate-limiting reaction of the catabolic pathway. This can be accomplished by the action of tryptophan 2,3-deoxygenase (TDO) or of indoleamine 2,3-deoxygenase (IDO). TDO is present in the liver while IDO is present in many tissues, including the placenta. IDO is induced by interferon gamma and inhibited by nitric oxide (NO). IDO can use superoxide (O2.-) as a substrate or as a co-factor. Catabolism of N-formylkynurenine results in the production of kynurenine, 3-HKyn, 3-HAA and several other metabolites. 3-HAA and 3-HKyn can function as peroxyl scavengers.
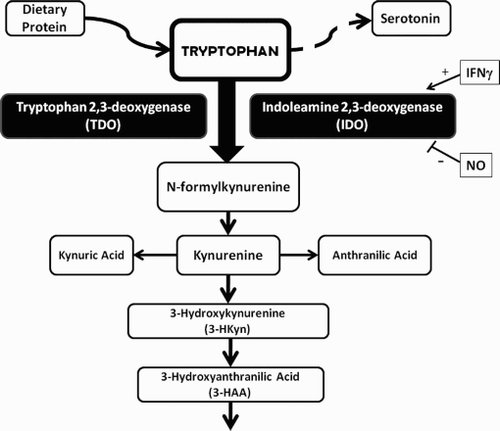
Oxidative stress is defined as an imbalance between the production of reactive oxygen species (ROS) and their clearance by antioxidants and can result in DNA, protein, and lipid damage. This is a fairly local effect, as ROS cannot cross the cell membrane. In this issue of SBiRM, Nishizawa et al. [2011], demonstrate cellular co-localization of IDO and DNA oxidative damage, supporting the hypothesis that these two findings may be related. IDO can utilize either oxygen (O2) or superoxide (O2.-) for its activity and superoxide may serve as both substrate and co-factor for IDO [Thomas and Stocker Citation1999]. IDO scavenges superoxide while 3-HAA and 3-HKyn scavenge peroxyl radicals, suggesting that induction of IDO by IFNγ may function as an important antioxidant [Christen et al. Citation1990]. This also supports Nishizawa and colleagues' hypothesis that decreased IDO activity may increase oxidative stress in the pre-eclamptic placenta. Others have shown that kynurenine:tryptophan ratios are increased in normotensive pregnant women but not in pre-eclamptic women [Kudo et al. Citation2003]. Still others have confirmed immunohistologically that IDO levels are lower in pre-eclamptic placentas when compared to controls [Santoso et al. Citation2002]. Invasive ctytotrophoblast cells have been shown to exhibit markers for oxidative stress in pre-eclamptic placentas [Many et al. Citation2000]. Finally tryptophan exposure attenuates macrophage-induced apoptosis of trophoblast-derived cells in vitro [Reister et al. Citation2001]. This could possibly protect the pre-eclamptic placenta from the pronounced macrophage infiltration in and around the maternal spiral arteries seen in vivo [Reister et al. Citation1999].
Cause versus effect in the pathophysiology of pre-eclampsia, however, remains elusive. The Nishizawa et al. manuscript reveals correlation between IDO activity and oxidative DNA damage in the pre-eclamptic placenta and may help re-direct our interest in placental IDO activity toward anti-oxidant rather than pure immunologic mechanisms. That said, IDO, tryptophan, and kyurenine have been implicated in the development of regulatory T cells [Puccetti and Grohmann Citation2007], which, in turn have been implicated in the pathogenesis of pre-eclampsia [Santner-Nanan et al. 2009]. Further, tryptophan depletion and increased IDO activity have been negatively correlated with tissue remodeling, an effect that can be reversed by tryptophan repletion [Varga et al. Citation1995]. Poor tissue remodeling is certainly central to pre-eclampsia, but is it a cause or an effect? The chicken versus egg dilemma in pre-eclampsia continues.
References
- Baban, B., Chandler, P., McCool, D., Marshall, B., Munn, D.H. and Mellor, A.L. (2004) Indoleamine 2,3–dioxygenase expression is restricted to fetal trophoblast giant cells during murine gestation and is maternal genome specific. J Reprod Immunol 61:67–77.
- Burton, G.J. (2009) Oxygen, the Janus gas; its effects on human placental development and function. J Anat 215:27–35.
- Burton, G.J. and Jauniaux, E. (2004) Placental oxidative stress: from miscarriage to preeclampsia. J Soc Gynecol Investig 11:342–352.
- Christen, S., Peterhans, E. and Stocker, R. (1990) Antioxidant activities of some tryptophan metabolites: possible implication for inflammatory diseases. Proc Natl Acad Sci USA 87:2506–2510.
- Jauniaux, E., Jurkovic, D., Campbell, S. and Hustin, J. (1992) Doppler ultrasonographic features of the developing placental circulation: Correlation with anatomic findings. Am J Obstet Gynecol 166:585–587.
- Jauniaux, E., Watson, A.L., Hempstock, J., Bao, Y.P., Skepper, J.N. and Burton, G.J. (2000) Onset of maternal arterial blood flow and placental oxidative stress. A possible factor in human early pregnancy failure. Am J Pathol 157:2111–2122.
- Kudo, Y., Boyd, C.A., Sargent, I.L. and Redman, C.W. (2003) Decreased tryptophan catabolism by placental indoleamine 2,3–dioxygenase in preeclampsia. Am J Obstet Gynecol 188:719–726.
- Lee, G.K., Park, H.J., Macleod, M., Chandler, P., Munn, D.H. and Mellor, A.L. (2002) Tryptophan deprivation sensitizes activated T cells to apoptosis prior to cell division. Immunology 107:452–460.
- Lum, H. and Roebuck, K.A. (2001) Oxidant stress and endothelial cell dysfunction. Am J Physiol Cell Physiol 280:C719–741.
- Many, A., Hubel, C.A., Fisher, S.J., Roberts, J.M. and Zhou, Y. (2000) Invasive cytotrophoblasts manifest evidence of oxidative stress in preeclampsia. Am J Pathol 156:321–331.
- Meekins, J.W., Luckas, M.J., Pijnenborg, R. and McFadyen, I.R. (1997) Histological study of decidual spiral arteries and the presence of maternal erythrocytes in the intervillous space during the first trimester of normal human pregnancy. Placenta 18:459–464.
- Munn, D.H., Zhou, M., Attwood, J.T., Bondarev, I., Conway, S.J., Marshall, B., (1998) Prevention of allogeneic fetal rejection by tryptophan catabolism. Science 281:1191–1193.
- Nishizawa, H., Hasegawa, K., Suzuki, M., Kamoshida, S., Kato, T., Saito, K., (2007) The etiological role of allogeneic fetal rejection in pre–eclampsia. Am J Reprod Immunol 58:11–20.
- Nishizawa, H., Kato, T., Ota, S., Nishiyama, S., Pryor–Koishi, K., Suzuki, M., (2010) Genetic variation in the indoleamine 2,3–dioxygenase gene in pre–eclampsia. Am J Reprod Immunol 64:68–76.
- Nishizawa, H., Suzuki, M., Pryor–Koishi, K., Sekiya, T., Tada, S., Kurahashi, H. and Udagawa, Y. (2011) Impact of indoleamine 2,3–dioxygenase on the antioxidant system in the placentas of severely pre–eclamptic patients. Syst Biol Reprod Med 57:174–178.
- Ozaki, Y., Edelstein, M.P. and Duch, D.S. (1988) Induction of indoleamine 2,3–dioxygenase: a mechanism of the antitumor activity of interferon gamma. Proc Natl Acad Sci USA 85:1242–1246.
- Palmer, A.E., London, W.T., Sly, D.L. and Rice, J.M. (1979) Spontaneous preeclamptic toxemia of pregnancy in the patas monkey (Erythrocebus patas). Lab Anim Sci 29:102–106.
- Puccetti, P. and Grohmann, U. (2007) IDO and regulatory T cells: a role for reverse signalling and non–canonical NF–kappaB activation. Nat Rev Immunol 7:817–823.
- Rajakumar, A., Doty, K., Daftary, A., Harger, G. and Conrad, K.P. (2003) Impaired oxygen–dependent reduction of HIF–1alpha and –2alpha proteins in pre–eclamptic placentae. Placenta 24:199–208.
- Redman, C.W. and Sargent, I.L. (2009) Placental stress and pre–eclampsia: a revised view. Placenta 30 Suppl A:S38–42.
- Reister, F., Frank, H.G., Heyl, W., Kosanke, G., Huppertz, B., Schroder, W., (1999) The distribution of macrophages in spiral arteries of the placental bed in pre–eclampsia differs from that in healthy patients. Placenta 20:229–233.
- Reister, F., Frank, H.G., Kingdom, J.C., Heyl, W., Kaufmann, P., Rath, W., (2001) Macrophage–induced apoptosis limits endovascular trophoblast invasion in the uterine wall of preeclamptic women. Lab Invest 81:1143–1152.
- Roberts, J.M. and Hubel, C.A. (2009) The two stage model of preeclampsia: variations on the theme. Placenta 30 Suppl A:S32–37.
- Rolfo, A., Many, A., Racano, A., Tal, R., Tagliaferro, A., Ietta, F., (2010) Abnormalities in oxygen sensing define early and late onset preeclampsia as distinct pathologies. PLoS One 5:e13288.
- Santner–Nanan, B., Peek, M.J., Khanam, R., Richarts, L., Zhu, E., Fazekas de St Groth, B., (2009) Systemic increase in the ratio between Foxp3+ and IL–17–producing CD4+ T cells in healthy pregnancy but not in preeclampsia. J Immunol 183:7023–7030.
- Santoso, D.I., Rogers, P., Wallace, E.M., Manuelpillai, U., Walker, D. and Subakir, S.B. (2002) Localization of indoleamine 2,3–dioxygenase and 4–hydroxynonenal in normal and pre–eclamptic placentae. Placenta 23:373–379.
- Steegers, E.A., von Dadelszen, P., Duvekot, J.J. and Pijnenborg, R. (2010) Pre–eclampsia. Lancet 376:631–644.
- Terness, P., Bauer, T.M., Rose, L., Dufter, C., Watzlik, A., Simon, H., (2002) Inhibition of allogeneic T cell proliferation by indoleamine 2,3–dioxygenase–expressing dendritic cells: mediation of suppression by tryptophan metabolites. J Exp Med 196:447–457.
- Thomas, S.M., Garrity, L.F., Brandt, C.R., Schobert, C.S., Feng, G.S., Taylor, M.W., (1993) IFN–gamma–mediated antimicrobial response. Indoleamine 2,3–dioxygenase–deficient mutant host cells no longer inhibit intracellular Chlamydia spp. or Toxoplasma growth. J Immunol 150:5529–5534.
- Thomas, S.R. and Stocker, R. (1999) Redox reactions related to indoleamine 2,3–dioxygenase and tryptophan metabolism along the kynurenine pathway. Redox Rep 4:199–220.
- Varga, J., Yufit, T. and Brown, R.R. (1995) Inhibition of collagenase and stromelysin gene expression by interferon–gamma in human dermal fibroblasts is mediated in part via induction of tryptophan degradation. J Clin Invest 96:475–481.
- Werner–Felmayer, G., Werner, E.R., Fuchs, D., Hausen, A., Reibnegger, G. and Wachter, H. (1989) Characteristics of interferon induced tryptophan metabolism in human cells in vitro. Biochim Biophys Acta 1012:140–147.