Abstract
It has been previously shown that cumuli oophori around ovulated oocytes of B10.BR-Ydel female mice (sired by males with the deleted Y chromosome) are more resistant to enzymatic treatment than cumuli oophori around eggs of control B10.BR females (having fathers with the intact Y chromosome). This can imply that some genes which influence the establishment of the imprinting pattern in male gametes are located in the region covered by the deletion. We hypothesize that the Y-dependent imprinting pattern, inherited by female offspring, affects stability of periovum layers within them. In the present study, cumulus-oocyte complexes ovulated by females from consomic strains: DBA, DBA-YBR, DBA-Ydel, and CBA, CBA-YBR, CBA-Ydel were tested for their susceptibility to hyaluronidase digestion. The mean times for dispersal of cumulus cells surrounding oocytes of females from the backcross lines were convergent with the times typical for oocytes from strains being the donors of the Y chromosome (B10.BR or B10.BR-Ydel) and differed clearly from pure DBA and CBA strains. It confirmed previous findings that Y chromosomes of fathers influence the properties of cumulus-oocyte complexes ovulated by their daughters. This influence is definitely stronger than the influence of the genetic background. Additionally, it was demonstrated that the cumuli oophori surrounding oocytes of B10.BR-Ydel females exhibit increased resistance to penetration by spermatozoa in vitro, in comparison to the control B10.BR strain. This regularity was reflected in the decreased proportion of fertilized ova recovered from oviducts of B10.BR-Ydel females mated with B10.BR-Ydel males and in the lower litter sizes recorded for these pairs. The excessive stability of cumuli oophori typical for oocytes of females having Ydel fathers may negatively affect their fertility, if they have partners producing poor quality sperm.
Introduction
Today infertility is a very common health problem, which affects both men and women. One of the frequent causes of male infertility is microdeletions within the AZF (azoospermia factor) region on the long arm of the Y chromosome. Depending on their extension and location, these deletions may lead to azoospermia or oligospermia as well as to severe abnormalities in sperm morphology and motility. The development of assisted reproductive techniques, especially ICSI (intracytoplasmic sperm injection), have enabled many infertile men with the Y chromosome deletions to become biological fathers. It has been shown that sons of such fathers, inheriting the mutated Y chromosome, may present severe fertility defects. In this way problems with male fertility are transmitted to the next generation [Roberts Citation1998; Stouffs et al. Citation2005; Georgiou et al. Citation2006; Mau Kai et al. Citation2008]. As we know, however, it has yet to be determined if deletions of the Y chromosome of fathers can somehow affect the reproductive efficiency of their female offspring. Mouse is a very convenient model organism to address this issue.
Ovulated mammalian oocytes, which wait in oviducts for fertilization, are enclosed by two layers: inner zona pellucida and outer cumulus oophorus. The zona pellucida, composed of three glycoproteins: ZP1, ZP2, and ZP3, effectively prevents interspecies fertilization. It has been shown that O-linked oligosaccharides on ZP3 are responsible for species-specific sperm binding leading to the acrosome reaction and penetration of a male gamete into the perivitelline space [Singson et al. Citation2001; Primakoff and Myles Citation2002; Talbot et al. Citation2003]. Cumulus oophorus consists of numerous granulosa cells that surround the oocyte in the antral follicle and in response to the preovulatory surge of luteinizing hormone produce extensive extracellular matrix in the process named cumulus expansion. Hyaluronan is the major component of the expanded cumulus oophorus extracellular matrix. Cross-linking its molecules with proteoglycans such as versican, TSG-6 (tumor necrosis factor stimulated gene 6), PTX3 (pentraxin 3) and with heavy chains of serum-derived protein ITI (inter-alpha-trypsin inhibitor) is indispensable for the proper formation of the complex structure [Salustri et al. Citation1989; Chen et al. Citation1996; Zhuo and Kimata Citation2001; Russell et al. Citation2003; Richards Citation2005; Scarchilli et al. Citation2007; Tamba et al. Citation2008]. Expanded cumulus oophorus accompanies the oocyte during ovulation and migration to the site of fertilization fulfilling many important functions. It protects the oocyte from mechanical stress and proteolytic enzymes as well as facilitates its capture by the ciliate epithelium of the infundibulum and transport into the oviduct [Lam et al. Citation2000; Tanghe et al. Citation2002; Tamba et al. Citation2008]. In the region where oocytes cluster, the interactions between cumuli oophori and oviductal epithelium induce swelling of walls which create the ampulla [Liu et al. Citation2008; Richards et al. Citation2008]. It has been shown that the cumulus layer considerably supports fertilization by trapping spermatozoa, guiding them towards the oocyte, facilitating sperm capacitation and the acrosomal reaction, and finally by keeping the oocyte in the state enabling fusion with a male gamete [Tanghe et al. Citation2002; Van Soom et al. Citation2002; Shimada and Richards Citation2010].
Numerous studies have revealed that females from separate inbred strains of mice show significant differential egg sensitivity to the attack of enzymes [Krzanowska Citation1972; Styrna Citation1986; Bander et al. Citation1988]. The stability of periovum layers is hence the feature which remains under a strong genetic control. Bander et al. [1989], studying the phenotypes of female mice from reciprocal F1 and F2 crosses between C57BL/6 and BALB/c strains, noticed that oocyte susceptibility to the enzymatic digestion is consequently inherited on the paternal line. The observed regularity has been explained by specific imprinting of genetic information which males transmit via spermatozoa to their daughters. The predominant role of the Y chromosome in the establishment of this imprinting seems a logical consequence of such reasoning.
In our laboratory two congenic strains of mice, B10.BR and B10.BR-Ydel, are studied. Animals from both strains have identical genotypes. The only difference is that B10.BR males possess an intact Y chromosome, while B10.BR-Ydel males carry the Y chromosome with a large deletion covering about 2/3 of the long arm. The deletion reduces the number of copies of Ssty1 and Ssty2 genes [Kotula-Balak et al. Citation2004]. Because genes from the Ssty (spermatid-specific transcripts Y-encoded) family control the spermiogenesis [Conway et al. Citation1994; Toure et al. Citation2004], males having the impaired Y chromosome produce spermatozoa with very frequent morphological and functional irregularities [Styrna et al. Citation1991a; Styrna et al. Citation1991b; Styrna et al. Citation2002; Grzmil et al. Citation2007]. It has been shown, additionally, that cumuli oophori surrounding ovulated oocytes of B10.BR-Ydel females (sired by males with the mutation in the Y chromosome) are definitely more resistant to the hyaluronidase digestion in comparison with cumulus-oocyte complexes of B10.BR females (sired by males with the intact Y chromosome). This regularity likely reflects different cumulus extracellular matrix composition and/or organization. The deletion in the long arm of the Y chromosome abolishes the effect of paternal genome imprinting typical for B10.BR strain. It implies that in the region covered by the deletion there are some genes which influence epigenetic modification of the sperm genome [Styrna Citation1995].
Epigenetic modifications lead to stable, heritable changes in gene expression pattern without altering the DNA sequence. DNA methylation is the fundamental and the most deeply studied epigenetic mechanism. It plays a very important role in germ cells and embryo development as well as in the establishment of genomic imprinting (resulting in unequal transcription from paternal and maternal alleles of some genes despite their absolutely identical sequence) [Trasler Citation2006; Trasler Citation2009]. Experiments on mice have shown that during spermatogenesis DNA methylation begins to be acquired in prospermatogonia and then is completed after birth in spermatogonia and spermatocytes, before entering the pachytene phase of meiosis [Trasler Citation2006; Schaefer et al. Citation2007; Trasler Citation2009]. Since de novo DNA methylation occurs in diploid male germ cells, genes of the Y chromosome may affect genome-wide epigenetic modifications. This would include genetic material which in meiotic division separates into spermatozoa with X chromosomes and is inherited by female offspring.
The influence of the Y chromosome on genomic imprinting has been shown through the comparison of sex-specific methylation patterns in germ cells of sex-reversed and control mouse embryos as well as in pluripotent stem cell lines derived from these germ cells. The examination has revealed that, apart from the strong effect of gonadal environment on the establishment of proper imprinting, in male germ cells there is an additional autonomous methylating activity dependent on the presence of the Y chromosome. This activity is not associated with Sry expression, because it has been observed also in cells carrying the Sry deleted Y chromosome [Durcova-Hills et al. Citation2006].
We initially measured susceptibility of cumulus-oocyte complexes (COCs) to digestion by hyaluronidase for female mice from DBA and CBA strains as well as consomic strains: DBA-YBR, DBA-Ydel, CBA-YBR, CBA-Ydel with the Y chromosome transferred, by the series of backcrosses, from B10.BR males (YBR) or from B10.BR-Ydel males (Ydel). This allowed us to assess the relative contribution of the autosomal background and genes linked with the Y chromosome of fathers to the formation of periovum constitution in female mice.
The cumulus oophorus surrounding the ovulated oocyte fulfils many important functions. But once the sperm reaches the COC, the cumulus layer is disassembled through a combination of motility and hyaluronidase activity. A resistant cumulus oophorus extracellular matrix may delay or even immobilize spermatozoa within this layer and consequently prevent fertilization [Tamba et al. Citation2008; Yodoi et al. Citation2009]. Comparing females from control B10.BR strain we tested whether the increased resistance of the cumulus oophorus to hyaluronidase, characteristic of B10.BR-Ydel ovulated oocytes, is associated with less effective disintegration of this layer by spermatozoa in vitro and with decreased fertilization efficiency in vivo.
Results
Resistance of the cumuli oophori to hyaluronidase treatment
The mean times of dispersal of cumulus cells with hyaluronidase for COCs isolated from oviducts of females from consomic strains: DBA, DBA-YBR, DBA-Ydel and CBA, CBA-YBR, CBA-Ydel are shown in and . These times are compared with the results obtained for congenic B10.BR and B10.BR-Ydel strains. Both in the case of DBA and CBA genetic backgrounds, dispersal of cumulus cells in hyaluronidase was rapid for COCs of females sired by males with the Y chromosome derived from B10.BR strain (YBR). The results for COCs of females from DBA-YBR strain (12.6 min) as well as of females from CBA-YBR strain (10.56 min) were very similar to that obtained for the B10.BR strain (10.49 min). In contrast, the cumulus layer of COCs isolated from oviducts of DBA-Ydel and CBA-Ydel females (sired by males with the deletion in the Y chromosome) exhibited increased resistance to enzymatic digestion (mean times of disintegration were respectively, 17.64 and 20.21 min), statistically equal to the resistance noticed for females from B10.BR-Ydel strain (mean time of disintegration: 16.41 min). The differences in rapidity of cumulus cell dispersal with hyaluronidase between COCs of females from DBA-YBR and DBA-Ydel strains as well as CBA-YBR and CBA-Ydel strains are statistically significant (P = 0.016 and P = 0.000, respectively). The results obtained for backcross lines of mice clearly differ from the results obtained for pure DBA and CBA strains and reflect the phenotypes of the Y chromosome donor strain.
Figure 1. Time to DBA cumulus cell dispersion. The amount of time required for hyaluronidase to disperse cumulus cells surrounding ovulated oocytes of DBA, DBA-YBR and DBA-Ydel females in comparison to COCs of B10.BR and B10.BR-Ydel females is shown (mean ± SD, n = 20 groups of COCs for each strain). Values which do not share the same a, b, c, d identifiers are significantly different (P < 0.05).
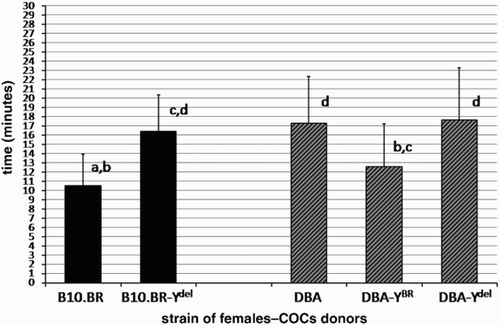
Figure 2. Time to CBA cumulus cell dispersion. The amount of time required for hyaluronidase to disperse cumulus cells surrounding ovulated oocytes of CBA, CBA-YBR and CBA-Ydel females in comparison to COCs of B10.BR and B10.BR-Ydel females is shown (mean ± SD, n = 20 groups of COCs for each strain). Values which do not share the same a, b, c, d identifiers are significantly different (P < 0.001).
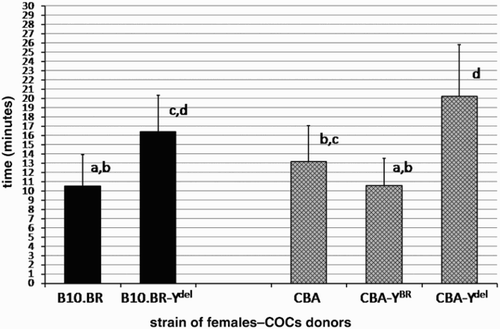
Resistance of cumuli oophori to sperm activity in vitro
Sixteen percent of the eggs of B10.BR-Ydel females were denuded of cumulus cells after a one hour incubation with B10.BR or B10.BR-Ydel spermatozoa. This result differs significantly (P = 0.000) from that obtained with control oocytes of B10.BR females, presenting cumuli oophori much more sensitive to sperm activity in vitro. After a one hour incubation as above, more than 50 % of eggs were devoid of cumulus layer ().
Table 1. Proportion of denuded oocytes with B10.BR or B10.BR-Ydel spermatozoa. The percent of B10.BR and B10.BR-Ydel oocytes denuded of cumulus cells after a one-hour incubation with B10.BR or B10.BR-Ydel spermatozoa is shown (mean ± SD, n = 12 groups of COCs for each type of the experiment).
Efficiency of in vivo fertilization
The fertilization efficiency () was significantly lower for oocytes recovered from the oviducts of females mated with B10.BR-Ydel males, as compared to those mated with B10.BR males. The comparison of the results obtained for B10.BR and B10.BR-Ydel females revealed that their oocytes were fertilized with a similar efficiency after mating with B10.BR males, but after mating with B10.BR-Ydel males a clear difference between them appeared (P = 0.038) A fertilization efficiency of approximately 73% for the ova of B10.BR females that fell to 50.6 % for eggs of females sired by fathers with the mutated Y chromosome was observed.
Table 2. Proportion of fertilized B10.BR and B10.BR-Ydel oocytes after mating with B10.BR or B10.BR-Ydel males. The percent of fertilized oocytes in oviducts of B10.BR and B10.BR-Ydel females after mating with B10.BR or B10.BR-Ydel males is shown (mean ± SD, n = 6 independent pairs for each type of mating).
The mean litter sizes calculated for all mating types is shown in . This data was more uniform compared to that obtained for the proportion of fertilized ova. Interestingly, the B10.BR females delivered the same number of pups regardless of whether they were bred with B10.BR or with B10.BR-Ydel males. B10.BR-Ydel males mated with females sired by Ydel fathers yielded a decrease of the mean litter size to the value of 5.02. This decrease is statistically significant in comparison with the control B10.BR strain (P = 0.03).
Table 3. Mean litter sizes (± SD) in mating of B10.BR and B10.BR-Ydel females with B10.BR or B10.BR-Ydel males (B10.BR females: n = 80 litters, B10.BR-Ydel females: n = 60 litters).
Discussion
Previous studies employing congenic B10.BR and B10.BR-Ydel strains imply that the Y chromosome influences the establishment of the imprinting pattern in male gametes. This pattern, inherited by female offspring, affects the stability of their oocyte investments [Styrna Citation1995]. As reported in the above we transferred the Y chromosomes of B10.BR and B10.BR-Ydel males to the genetic backgrounds of DBA and CBA strains. This allowed us to assess the degree to which the father's Y chromosome may influence COCs features within their daughters. We noticed that the sensitivity of cumuli oophori to digestion with hyaluronidase for COCs of females from backcross lines of mice clearly differs from the sensitivity observed in pure DBA and CBA strains. The resulting phenotype is reflective of the donor Y chromosome strain. Regardless of the genetic background, all examined females sired by males with YBR ovulated COCs which were very sensitive to hyaluronidase treatment. In comparison, all females having fathers with the mutated Y chromosome (Ydel) ovulated COCs with comparatively resistant cumulus layers. These observations strongly suggest that the Y chromosome contribution to paternal imprinting has a significant influence on COCs susceptibility of daughters. This influence is definitely stronger, than the influence of the genetic background.
Cells and rich extracellular matrix of expanded cumulus oophorus accompany the oocyte during ovulation and migration to the oviductal ampulla. Fertilization is facilitated with the various interactions of this layer with spermatozoa [Salustri et al. Citation1989; Zhuo and Kimata Citation2001; Tanghe et al. Citation2002; Van Soom et al. Citation2002]. Excessive firmness of postovulatory cumuli oophori may, however, negatively affect fertilization rates and may be similar to that observed for prostaglandin E receptor EP2–deficient female mice (Ptger2-/-). As a result of chronic chemokine signalling, cumulus cells around their oocytes produce extracellular matrix which is resistant to hyaluronidase treatment in vitro, as well as in vitro and in vivo sperm penetration. The excessive accumulation and stability of cumulus extracellular matrix is responsible for the severe fertilization defect characteristic for Ptger2-/- females [Tamba et al. Citation2008]. COCs of females sired by males with the deleted Y chromosome (B10.BR-Ydel) also exhibit the increased resistance to the hyaluronidase digestion, in comparison with control B10.BR strain [Styrna Citation1995]. Accordingly, we tested if the increased stability of cumuli oophori surrounding oocytes of B10.BR-Ydel females transposes on the rapidity and the effectiveness by which they are penetrated by spermatozoa. In vitro incubation of B10.BR and B10.BR-Ydel COCs with spermatozoa, clearly showed that cumuli oophori around oocytes of females sired by fathers with Ydel are resistant to dispersion by the activity of male gametes. In the case of COCs of control B10.BR females, one hour was sufficient for spermatozoa to denude more than 50% of the oocytes. In comparison, when B10.BR-Ydel COCs were examined, the great majority of oocytes remained covered with cumulus cells. This suggests that the stability of cumuli oophori around the oocytes of female mice, which is under the influence of paternal genome imprinting, may modulate oocyte availability for spermatozoa and hence affect the efficiency of fertilization.
To examine this notion, we compared the fertilization rates obtained for oocytes recovered from oviducts of B10.BR and B10.BR-Ydel females after mating with B10.BR or B10.BR-Ydel males. This confirmed previous findings that sperm of the males carrying the deleted Y chromosome are highly inefficient at fertilization both in vitro [Xian et al. Citation1992] and in vivo [Styrna et al. Citation2002]. In the present study, as in the former experiments [Styrna et al. Citation2002], we obtained a significantly lower proportion of fertilized ova after mating with B10.BR-Ydel males, than after mating with control B10.BR males. Undoubtedly, this difference results from the definitely poorer quality of gametes produced by B10.BR-Ydel males. The low quality of B10.BR-Ydel spermatozoa has been documented. It is manifested in frequent morphological and ultrastructural irregularities [Styrna et al. Citation1991a,Citationb; Styrna et al. Citation2002], delay in epididymal maturation [Styrna et al. Citation2002], decreased effectiveness of movement [Grzmil et al. Citation2007], and in displaying serious difficulty while crossing the uterotubal junction of the female reproductive tract [Styrna et al. Citation2002; Kotarska and Lenartowicz Citation2011]. It is likely that all these factors contributed to the low proportion of fertilized eggs obtained in the current investigation when mating was carried out with a Ydel male.
Comparing the fertilization efficiency between oocytes of B10.BR and B10.BR-Ydel females revealed that the increased resistance of cumuli oophori surrounding oocytes of B10.BR-Ydel females has no effect on the fertilization rates if these females are mated with B10.BR males, producing sperm of a relatively good quality. However, when crossed with B10.BR-Ydel males, the negative influence of cumuli oophori around oocytes of B10.BR-Ydel females becomes quite clear. Whereas control B10.BR females give about 73% of fertilized ova after mating with B10.BR-Ydel males, the B10.BR-Ydel females reach the fertilization rate of approximately 50%. The increased stability of cumuli oophori characteristic for oocytes of females sired by fathers with the deleted Y chromosome may hence indeed lessen fertilization efficiency, if only males producing spermatozoa of poor quality are used for mating. The resistance of cumulus extracellular matrix combined with very low parameters of sperm quality likely make the oocytes residing in oviducts to be almost unavailable for male gametes.
The proportion of oocytes successfully fertilized in oviducts influences the maximal number of pups which can be bred from a female mouse. We evaluated the mean litter sizes for the four investigated mating paradigms to determine whether the increased resistance of cumuli oophori around oocytes of females having fathers with Ydel affects their fecundity. Despite quite a large divergence in the proportions of fertilized ova in oviducts of females from different mating paradigms, the mean litter sizes appeared to be very similar. Presumably, the variations in fertilization efficiency are muted during blastocyst implantation and subsequent embryonic development. Even differences in sperm quality between B10.BR and B10.BR-Ydel males are not reflective of litter size, when these males are maintained in pairs with B10.BR females. This is in accord with Conway et al. [1994], who compared the breeding records for males carrying the R III Y-del chromosome (with a partial deletion in the long arm, very similar to this described for B10.BR-Ydel males). They did not observe any clear difference when compared to control animals. This is reflective of the capacity of the organism to compensate for various irregularities in functioning. In mice, at each estrus cycle many oocytes are simultaneously ovulated. Thus a lower fertilization efficiency can be recompensed by a higher percentage of successfully developed embryos. This is not possible in species like humans, where usually only one oocyte is released from the ovary. But when B10.BR-Ydel males were mated with B10.BR-Ydel females (sired by Ydel fathers), the fall of the average litter size became noticeable and statistically significant in comparison with pairs from control B10.BR strain. This observation allows us to conclude that in the case of mating with males producing sperm of a very poor quality, the excessive stability of cumuli oophori around oocytes of females having Ydel fathers can lower the fertilization efficiency in the degree that exceeds the compensatory ability of the female and consequently negatively impacts fertility.
The great importance of genes located in the long arm of the Y chromosome for spermiogenesis and male fertility is well-known. Our study suggests, that through the establishment of paternal genome imprinting, genes of the Y chromosome of fathers may influence the reproductive abilities of their female offspring. This may provide the means to better understand the challenges associated with using assisted reproductive techniques.
Materials and Methods
Animals and hormonal treatment
Adult, 10-12 week old female and male mice from congenic B10.BR and B10.BR-Ydel strains as well as females from consomic strains: DBA, DBA-YBR, DBA-Ydel and CBA, CBA-YBR, CBA-Ydel were used in the experiments. Mutant B10.BR-Ydel strain is charged with a partial deletion in the long arm of the Y chromosome, which distinguishes it from control B10.BR strain with the intact Y chromosome. To avoid the possibility of divergence between these two strains, both B10.BR and B10.BR-Ydel males are mated in each generation with B10.BR females.
DBA-YBR, DBA-Ydel, CBA-YBR, and CBA-Ydel strains differ from pure DBA and CBA strains only in that their Y chromosomes derive from B10.BR (YBR) or B10.BR-Ydel (Ydel) males. These strains were obtained by mating B10.BR or B10.BR-Ydel males with DBA or CBA females. Hybrid males were then backcrossed by at least 10 generations to DBA or CBA females.
To stimulate follicular growth and ovulation, female mice were injected intraperitoneally with 8 IU of pregnant mare serum gonadotropin (PMSG) and 48 h later with 8 IU of human chorionic gonadotropin (hCG).
All mice were bred at the Department of Genetics and Evolution of Jagiellonian University, Krakow, Poland maintained under a 12 h light-dark cycle, and fed on a commercial pellet diet. Experiments were performed in accordance with Polish legal requirements, under the license of the First Local Ethics Commission for Animal Experiments in Jagiellonian University.
Resistance of the cumuli oophori to hyaluronidase treatment
14 h post hCG injection B10.BR, B10.BR-Ydel, DBA, DBA-YBR, DBA-Ydel, CBA, CBA-YBR, and CBA-Ydel females were sacrificed by cervical dislocation. The COCs removed from each oviduct were transferred to separate 0.3 ml drops of hyaluronidase solution in PBS (Sigma-Aldrich, Germany, 24 IU/ml) covered with mineral oil (Sigma-Aldrich, Germany). The dishes with COCs from individual oviducts were kept on a warm plate at 37°C and observed under a light microscope. For each oocyte, the time needed to disperse the surrounding granulosa cells was noted. The median dispersion times for groups of COCs obtained from 20 single oviducts of 10 females were used to calculate the mean and SD for each strain. Groups numbering less than 10 COCs were excluded from the experiment.
Resistance of cumuli oophori to sperm activity in vitro
B10.BR or B10.BR-Ydel males were sacrificed and their vas deferens together with epididymides were resected. After gentle pressing of cauda epididymis, the content of both vas deferens was squeezed out into a 0.4 ml drop of modified Tyrode's medium (mT6) [Nagy et al. Citation2003] covered with mineral oil. The sperm in mT6 medium were then incubated for 1.5 h at 37°C in 5% CO2.
COCs isolated 14 h post hCG injection from individual oviducts of B10.BR and B10.BR-Ydel females (at least 10 COCs in each group) were introduced into separate 0.2 ml drops of mT6 medium under mineral oil. Then sperm suspension was added to the drops containing COCs in the amount giving a final concentration of 1,000 male gametes per 1 µl. After 1 h incubation (37°C, 5% CO2) with spermatozoa, oocytes were observed under a microscope. The numbers of denuded eggs and eggs still surrounded with cumulus cells were counted. In each experiment, sperm of one male were used to disperse COCs isolated from oviducts of one B10.BR female and one B10.BR-Ydel female. For both types of sperm (B10.BR and B10.BR-Ydel), 6 independent experiments were performed.
Efficiency of in vivo fertilization
Immediately after hCG injection, B10.BR and B10.BR-Ydel females were placed individually into a cage with a B10.BR or with a B10.BR-Ydel male and 2 h later checked for the presence of a vaginal plug. Females with the vaginal plugs were sacrificed 42 h after hCG injection (about 30 h after ovulation and fertilization). Their oviducts were resected and placed into a small drop of M2 medium (Sigma-Aldrich, Germany). The oviductal walls were then torn open with a needle allowing the content to flow out into the medium. For each female, the number of obtained two-cell embryos as well as undivided (unfertilized) oocytes were counted. Undivided oocytes were kept until the next day in M2 medium under mineral oil (37°C, 5% CO2) to ensure that they were unfertilized. Results for females which had less than 15 oocytes in both oviducts were excluded from the general evaluation. For each type of mating, 6 independent pairs of mice were successfully examined.
Additionally, the mean litter size for the four investigated types of mating was calculated on the basis of the breeding records kept in our laboratory. In each case, litters of at least 20 independent pairs of mice were taken into account. Especially for this evaluation B10.BR-Ydel females were maintained in pairs with males, although otherwise they are not used for reproduction.
Statistical analysis
For statistical analysis all results obtained in percentages were normalized using angular transformation. Differences between times of dispersal of cumulus cells in hyaluronidase solution were evaluated by a parametric analysis of variance (ANOVA) followed by a post-hoc Tukey's test. For the data presenting cumuli oophori resistance to sperm activity in vitro as well as proportions of fertilized oocytes in oviducts of females, a Student's t-test (for equal variances) was applied. Litter sizes were compared using a Mann-Whitney U test. The P value below 0.05 was considered statistically significant.
Declaration of Interest: This work was supported by grant 8168/B/P01/2011/40 from Polish Ministry of Science and Higher Education. The authors report no conflict of interest both financial and personal.
Abbreviations
AZF: | = | azoospermia factor |
Bik: | = | bikunin |
COC: | = | cumulus-oocyt complex |
hCG: | = | human chorionic gonadotropin |
ICSI: | = | intracytoplasmic sperm injection |
ITI: | = | inter-alpha-trypsin inhibitor |
mT6: | = | modified Tyrode's medium |
PBS: | = | phosphate buffered saline |
PMSG: | = | pregnant mare serum gonadotropin |
Ptger2: | = | prostaglandin E receptor 2 |
PTX3: | = | pentraxin 3; SD: standard deviation |
Sry: | = | sex-determining region Y |
Ssty: | = | spermatid-specific transcripts Y-encoded |
TSG-6: | = | tumor necrosis factor stimulated gene 6. |
References
- Bander, S.A., Watson, S.C. and Shire, J.G. (1988) Genetic differences in periovum sensitivity to hyaluronidase and protease between C57BL/6, BALB and CXB recombinant mice. J Reprod Fert 84:709–714.
- Bander, S.A., Watson, S.C. and Shire, J.G. (1989) Paternal inheritance of egg traits in mice: a case of genomic imprinting. Genet Res 54:213–219.
- Chen, L., Zhang, H., Powers, R.W., Russell, P.T. and Larsen, W.J. (1996) Covalent linkage between proteins of the inter-α-inhibitor family and hyaluronic acid is mediated by a factor produced by granulosa cells. J Biol Chem 271:19409–19414.
- Conway, S.J., Mahadevaiah, S.K., Darling, S.M., Capel, B., Rattigan, A.M. and Burgoyne, P.S. (1994) Y353/B: a candidate multiple-copy spermiogenesis gene on the mouse Y chromosome. Mamm Genome 5:203–210.
- Durcova-Hills, G., Hajkova, P., Sullivan, S., Barton, S., Surani, M.A. and McLaren, A. (2006) Influence of sex chromosome constitution on the genomic imprinting of germ cells. Proc Natl Acad Sci USA 103:11184–11188.
- Georgiou, I., Syrrou, M., Pardalidis, N., Karakitsios, K., Mantzavinos, T., Giotitsas, N. (2006) Genetic and epigenetic risks of intracytoplasmic sperm injection method. Asian J Androl 8:643–673.
- Grzmil, P., Gołas, A., Müller, C. and Styrna, J. (2007) The influence of the deletion on the long arm of the Y chromosome on sperm motility in mice. Theriogenology 67:760–766.
- Kotarska, K. and Lenartowicz, M. (2011) Sperm migration and selection in the reproductive tract of female mice is mostly affected by male genotype. Folia Biol 59:71–75.
- Kotula-Balak, M., Grzmil, P., Styrna, J. and Bilińska, B. (2004) Immunodetection of aromatase in mice with a partial deletion in the long arm of the Y chromosome. Acta histochem 106:55–64.
- Krzanowska, H. (1972) Rapidity of removal in vitro of the cumulus oophorus and the zona pellucida in different strains of mice. J Reprod Fert 31:7–14.
- Lam, X., Gieseke, C., Knoll, M. and Talbot, P. (2000) Assay and importance of adhesive interaction between hamster (Mesocricetus auratus) oocyte-cumulus complexes and the oviductal epithelium. Biol Reprod 62:579–588.
- Liu, Z., Shimada, M. and Richards, J.S. (2008) The involvement of the Toll-like receptor family in ovulation. J Assist Reprod Genet 25:223–228.
- Mau Kai, C., Juul, A., McElreavey, K., Ottesen, A.M., Garn, I.D., Main, K.M. (2008) Sons conceived by assisted reproduction techniques inherit deletions in the azoospermia factor (AZF) region of the Y chromosome and the DAZ gene copy number. Hum Reprod 23:1669–1678.
- Nagy, A., Gertsenstein, M., Vintersten, K. and Behringer, R. (2003) Manipulating the Mouse Embryo: A laboratory manual, Cold Spring Harbor Laboratory Press, Cold Spring Harbor, New York, USA.
- Primakoff, P. and Myles, D.G. (2002) Penetration, adhesion, and fusion in mammalian sperm-egg interaction. Science 296:2183–2185.
- Richards, J.S. (2005) Ovulation: New factors that prepare the oocyte for fertilization. Mol Cel Endocrinol 234:75–79.
- Richards, J.S., Liu, Z. and Shimada, M. (2008) Immune-like mechanisms in ovulation. Trends Endocrinol Metabol 19:191–196.
- Roberts, K.P. (1998) Y chromosome deletions and male infertility: state of the art and clinical implications. J Androl 19:255–259.
- Russell, D.L., Ochsner, S.A., Hsieh, M., Mulders, S. and Richards, J.S. (2003) Hormone-regulated expression and localization of versican in the rodent ovary. Endocrinology 144:1020–1031.
- Salustri, A., Yanagishita, M. and Hascall, V.C. (1989) Synthesis and accumulation of hyaluronic acid and proteoglycans in the mouse cumulus cell-oocyte complex during follicle-stimulating hormone-induced mucification. J Biol Chem 264:13840–13847.
- Scarchilli, L., Camaioni, A., Bottazzi, B., Negri, V., Doni, A., Deban, L. (2007) PTX3 interacts with inter-α-trypsin inhibitor: Implications for hyaluronan organization and cumulus oophorus expansion. J Biol Chem 282:30161–30170.
- Schaefer, C.B., Ooi, S.K.T., Bestor, T.H. and Bourc'his, D. (2007) Epigenetic decisions in mammalian germ cells. Science 316:398–399.
- Shimada, M. and Richards, J.S. (2010) Cumulus cells are an essential mediator of ovulation stimuli from granulosa cells to oocyte. J Mamm Ova Res 27:2–10.
- Singson, A., Zannoni, S. and Kadandale, P. (2001) Molecules that function in the steps of fertilization. Cytokine Growth Factor Rev 12:299–304.
- Stouffs, K., Lissens, W., Tournaye, H., Van Steirteghem, A. and Liebaers, I. (2005) The choice and outcome of the fertility treatment of 38 couples in whom the male partner has a Yq microdeletion. Hum Reprod 20:1887–1896.
- Styrna, J. (1986) Solubility of zonae pellucidae of unfertilized and fertilized mouse ova in different chemical reagents. Zwierzeta Lab 23:3–10.
- Styrna, J. (1995) Partial deletion of the Y chromosome removes the effect of paternal genome imprinting on periovum sensitivity to hyaluronidase in mice. Genet Res 65:229–231.
- Styrna, J., Bilińska, B. and Krzanowska, H. (2002) The effect of a partial Y chromosome deletion in B10.BR-Ydel mice on testis morphology, sperm quality and efficiency of fertilization. Reprod Fertil Dev 14:101–108.
- Styrna, J., Imai, H.T. and Moriwaki, K. (1991a) An increased level of sperm abnormalities in mice with a partial deletion of the Y chromosome. Genet Res 57:195–199.
- Styrna, J., Klag, J. and Moriwaki, K. (1991b) Influence of partial deletion of the Y chromosome on mouse sperm phenotype. J Reprod Fert 92:187–195.
- Talbot, P., Shur, B.D. and Myles, D.G. (2003) Cell adhesion and fertilization: Steps in oocyte transport, sperm-zona pellucida interactions and sperm-egg fusion. Biol Reprod 68:1–9.
- Tamba, S., Yodoi, R., Segi-Nishida, E., Ichikawa, A., Narumiya, S. and Sugimoto, Y. (2008) Timely interaction between prostaglandin and chemokine signaling is a prerequisite for successful fertilization. Proc Natl Acad Sci USA 105:14539–14544.
- Tanghe, S., Van Soom, A., Nauwynck, H., Coryn, M. and de Kruif, A. (2002) Minireview: Functions of the cumulus oophorus during oocyte maturation, ovulation, and fertilization. Mol Reprod Dev 61:414–424.
- Toure, A., Grigoriev, V., Mahadevaiah, S.K., Rattigan, A., Ojarikre, O.A. and Burgoyne, P.S. (2004) A protein encoded by a member of the multicopy Ssty gene family located on the long arm of the mouse Y chromosome is expressed during sperm development. Genomics 83:140–147.
- Trasler, J.M. (2006) Gamete imprinting: setting epigenetic patterns for the next generation. Reprod Fertil Dev 18:63–69.
- Trasler, J.M. (2009) Epigenetics in spermatogenesis. Mol Cell Endocrinol 306:33–36.
- Van Soom, A., Tanghe, S., De Pauw, I., Maes, D. and de Kruif, A. (2002) Function of the cumulus oophorus before and during mammalian fertilization. Reprod Dom Anim 37:144–151.
- Xian, M., Azuma, S., Naito, K., Kunieda, T., Moriwaki, K. and Toyoda, Y. (1992) Effect of a partial deletion of Y chromosome on in vitro fertilizing ability of mouse spermatozoa. Biol Reprod 47:549–553.
- Yodoi, R., Tamba, S., Morimoto, K., Segi-Nishida, E., Nishihara, M., Ichikawa, A. (2009) RhoA/Rho kinase signaling in the cumulus mediates extracellular matrix assembly. Endocrinology 150:3345–3352.
- Zhuo, L. and Kimata, K. (2001) Cumulus oophorus extracellular matrix: Its construction and regulation. Cell Struct Funct 26:189–196.