Abstract
Inflammation is known to be involved in the postoperative adhesion development. Interleukin (IL)-6 and tumor necrosis factor (TNF)-α are cytokines that stimulate the acute-phase reaction, which leads to a systemic reaction including inflammation, fever, and activation of the complement and clotting cascades. The goal of this study was to examine the expression of these inflammatory markers, under normal and hypoxic conditions, in normal and adhesion fibroblasts. Primary cultures of fibroblasts were established from normal peritoneum and adhesion tissues from the same patient(s) and cultured under 20% O2 or hypoxic 2% O2 conditions for 24 hours. Cells were harvested and total RNA was isolated. Complimentary DNA was generated by reverse transcription and subjected to real-time RT-PCR using specific primers for IL-6 and TNF-α. Both normal peritoneal and adhesion fibroblasts expressed IL-6 and TNF-α. Adhesion fibroblasts exhibited significantly higher levels of IL-6 and TNF-α mRNA as compared to normal peritoneal fibroblasts (p < 0.05). Both IL-6 and TNF-α mRNA levels were upregulated in response to hypoxia in both normal peritoneal and adhesion fibroblasts. The increase in IL-6 and TNF-α mRNA levels of normal fibroblasts reached the levels observed in adhesion fibroblasts. Our results suggest that hypoxia promotes the development of the adhesion phenotype by the induction of inflammatory markers, which may contribute to the development of postoperative adhesions. The inhibition of inflammation may be a potential therapeutic approach in the prevention and/or reduction of postoperative adhesion development.
Keywords:
Introduction
Postoperative adhesion development remains a nemesis to surgeons and their patients. Adhesions are the most common complication of abdominal and pelvic surgery [Diamond Citation2001]. They are a major initiating cause of small bowel obstruction [Ellis Citation1997], pelvic pain [Steege and Stout Citation1991], and infertility [Caspi et al. Citation1979]. When present in re-operative procedures, they can prolong operative duration and increase the risk of intraoperative and postoperative complications [Diamond and Hershlag Citation1990]. Postoperative adhesions form and reform frequently because peritoneal repair is a pro-fibrotic event, allowing the development of attachments between different tissues.
Injury of the peritoneum leads to an inflammatory response consisting of hyperemia, fluid exudation, recruitment of floating mesothelial cells, and release of white blood cells and platelets into the cavity peritoneal [Corona et al. Citation2011; diZerega Citation1997; Pismensky et al. Citation2011; ten Broek et al. Citation2011]. Injury to the mesothelial cell layer exposes the underlying connective tissue to the peritoneal fluid. This results in an increase in leukotriene B4 and prostaglandin E2 (PGE(2)) levels and inhibition of plasminogen activator activity (PAA) in the peritoneal fluid [Liakakos et al. Citation2001]. The former stimulates adhesiogenesis, while the inhibition of the latter diminishes the degradation of fibrin, resulting in an imbalance that favors adhesion formation [Liakakos et al. Citation2001]. Injury also results in the release of thromboplastin and the activation of clotting cascades that end in formation of fibrin [Gomel et al. Citation1996]. If fibrin is not lysed, it provides the matrix for adhesion formation. The peritoneal fluid also contains a small amount of leukocytes, mainly macrophages, which dramatically increase in number and activity in response to peritoneal injury [diZerega and Campeau Citation2001]. Macrophages phagocytize cellular debris, and secrete a variety of substances, including cytokines, specifically interleukin (IL)-1, IL-6, and tumor necrosis factor (TNF)-α [diZerega Citation1997]. Additionally, cytokines secreted by macrophages at the site of peritoneal injury play an integral role in regulating coagulation and fibrin formation, thereby influencing the development of adhesions [Holmdahl and Ivarsson Citation1999]. In particular, proinflammatory cytokines, (i.e., IL-1, IL-6, transforming growth factor (TGF)-β, and granulocyte-macrophage colony stimulating factor) regulate inflammatory and immunologic responses, and are activated during peritoneal wound healing [Holmdahl and Ivarsson Citation1999].
Adhesion formation, caused by surgical trauma to the tissues and ensuing inflammatory processes, is not likely to be adequately controlled unless the pathophysiological mechanism at the cellular and molecular levels are understood. Many studies delineating the mechanisms by which hypoxia induces the development of adhesions have been reported [Alpay et al. Citation2008; Atta Citation2011]. Hypoxia, through the production of superoxide (O2•−) and the formation of peroxynitrite (ONOO−), causes normal peritoneal fibroblasts to acquire the adhesion phenotype, which is characterized by increased α-smooth muscle actin, increased transforming growth factors and cytokines and extracellular matrix production, decreased turnover via effects on matrix-degrading enzymes and their inhibitors, as well as decreased apoptosis [Alpay et al. Citation2008; Fletcher et al. Citation2008; Idell et al. Citation1992; Imudia et al. Citation2008; Saed and Diamond Citation2003; Citation2004a; Saed et al. Citation2003; Saed et al. Citation2000; Sandell Citation1996]. Scavenging O2•−, even in the presence of hypoxia, prevented the development of the adhesion phenotype [Fletcher et al. Citation2008]. More importantly, hypoxia irreversibly induced the development of the adhesion phenotype, resulting in lower apoptosis through a mechanism involving the S-nitrosylation of caspase-3 [Jiang et al. Citation2009]. Therefore, this study investigated the effect of hypoxia on the expression of IL-6 and TNF-α, powerful inflammatory markers, in fibroblasts isolated from human normal peritoneum and adhesion tissues, providing further characterization of the adhesion phenotype.
Results
Adhesion fibroblasts exhibited significantly higher levels of IL-6 mRNA (35%) as compared to normal peritoneal fibroblasts (, p < 0.05). Hypoxia treatment increased IL-6 mRNA levels by 39% in normal and by 14% in adhesion fibroblasts (, p < 0.05). In the hypoxia group, adhesion fibroblasts exhibited higher levels of IL-6 mRNA than their normal peritoneal counterparts; however, the effect was less pronounced than under normoxic conditions (11% increase versus the aforementioned 39% increase; , p < 0.05). Additionally, normal fibroblasts exposed to hypoxia expressed levels of IL-6 observed in untreated adhesion fibroblasts.
Figure 1. IL-6 expression in normal peritoneal and adhesion fibroblasts. Adhesion fibroblasts had increased IL-6 expression as compared to normal peritoneal fibroblasts. Exposure to hypoxia increased IL-6 expression in both normal and adhesion fibroblasts. (*, ** p < 0.05 as compared to normal peritoneal fibroblasts, *** p < 0.05 as compared to untreated adhesion fibroblasts and compared to hypoxia treated normal peritoneal fibroblasts).
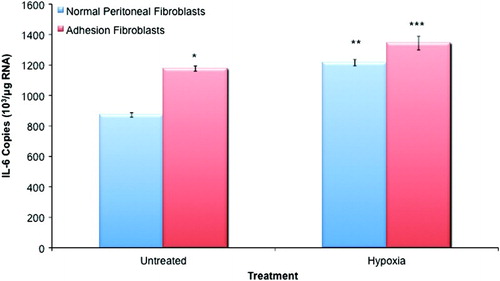
Similarly, TNF-α was expressed in both normal peritoneal and adhesion fibroblasts. Adhesion fibroblasts exhibited significantly higher levels of TNF-α mRNA as compared to normal peritoneal fibroblasts (58% increase; , p < 0.05). Treatment with hypoxia increased TNF-α mRNA levels by 45% in normal and by 36% in adhesion fibroblasts (, p < 0.05). Hypoxia increased TNF-α mRNA by 25% in adhesion as compared to normal peritoneal fibroblasts; however, the effect was less pronounced than observed under normoxic conditions (25% increase versus the aforementioned 58% increase; , p <0.05). Once again, normal peritoneal fibroblasts subject to hypoxia exhibited similar TNF-α levels observed in the untreated adhesion fibroblasts.
Figure 2. TNF-α expression in normal peritoneal and adhesion fibroblasts. Adhesion fibroblasts had increased TNF-α expression as compared to normal peritoneal fibroblasts. Exposure to hypoxia increased TNF-α expression in both normal and adhesion fibroblasts. (*, ** p < 0.05 as compared to normal peritoneal fibroblasts, *** p < 0.05 as compared to untreated adhesion fibroblasts and compared to hypoxia treated normal peritoneal fibroblasts).
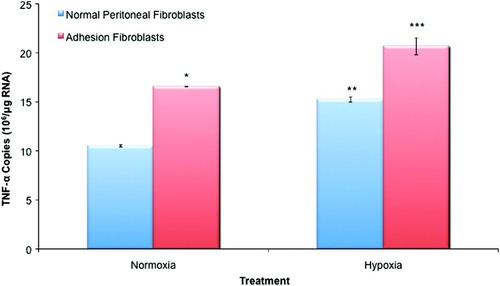
Figure 3. The adhesion phenotype. The adhesion phenotype can be induced during in vitro culture of human peritoneal fibroblasts by exposure to hypoxia. When compared to normal peritoneal fibroblasts, these changes include increases in interferon (IFN)-γ, TGF-β1, ECM markers including type I and III collagens as well as fibronectin, COX2, VEGF, iNOS, and decreases in TGF-β3, MMPs, the ratio of tPA to PAI-1, apoptosis, and NO.
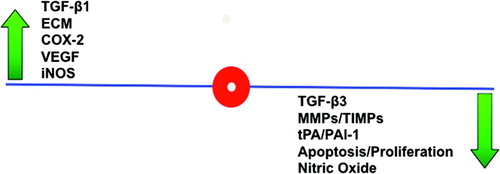
Discussion
Pelvic adhesions may form in response to intraperitoneal inflammatory processes, as a result of tissue handling, introduction of foreign bodies, tissue dryness, and size of the midline incision [Corona et al. Citation2011; Pismensky et al. Citation2011; ten Broek et al. Citation2011]. Trauma to the peritoneal surface is known to decrease tissue plasminogen activator (tPA) and increase the concentrations of plasminogen activator inhibitor-1 (PAI-1), which collectively result in a reduction of PAA and as a consequence, a reduction in the lysis of the fibrinous mass deposited within the abdominal cavity [Whawell and Thompson Citation1995]. These fibrin deposits, if they persist, are thought to be the origins of permanent fibrous adhesions. The inflammatory cytokines TNF, IL-6, and IL-1 individually increase the levels of PAI-1 released by endothelial cells [Cheong et al. Citation2001; Liakakos et al. Citation2001; Whawell and Thompson Citation1995]. These observations suggest that production of PAI-1 during peritoneal inflammation is controlled in part by multiple interactions between cytokines and cells producing tPA and PAI-1, leading to adhesion formation and reformation [Whawell and Thompson Citation1995].
Cytokines have important roles in regulating the inflammatory and immunological response to challenges [Holmdahl et al. Citation1997]. IL-6 is a multifunctional cytokine able to behave as a growth and differentiation factor and can induce the expression of other genes [Badia Citation1997]. The expression and production of IL-6 is indicative of cytokine-networking, whereby one cell population is dependent on mediators synthesized by a neighboring cell [Lanfrancone et al. Citation1992]. IL-6 has been considered to be an early maker of tissue damage, known to be stimulated by IL-1 and TNF-α in mesothelial cells in both a time and dose-dependent manner [Lanfrancone et al. Citation1992; Topley et al. Citation1993]. IL-6 stimulates the acute phase inflammation reaction that leads to a systemic response, including inflammation, fever, and activation of both the complement and clotting cascades. Thus, it has potential effects on a variety of adhesion-related processes including angiogenesis, fibrinolysis, and extracellular matrix remodeling. TNF-α has been shown to have several functions within the abdominal cavity. In vitro, it induces the production of interleukins (IL-1α, IL-1β, and IL-8) from mesothelial cell cultures, which suggests TNF-α may modulate the inflammatory response [Holmdahl and Ivarsson Citation1999]. It increases the expression of adhesion molecules in mesothelial cells, which allows interaction with leukocytes, promotes coagulation by the induction of tissue factor, and promotes the persistence of fibrin by reduction of tPA protein expression as well as increasing the release of PAI-1 from mesothelial cells [Whawell and Thompson Citation1995]. In this study we have shown that hypoxia induced the expression of IL-6 and TNF-α in normal peritoneal fibroblasts to levels observed in adhesion fibroblasts. The mechanisms by which these cytokines contribute to overall adhesion development are not yet known as other sources of these cytokines may also contribute to the development of adhesions (i.e., macrophages, lymphocytes, etc). However, this study clearly shows that these cytokines are indeed made by normal peritoneal fibroblasts and are possibly utilized in an autocrine fashion, which contributes to the persistence of the adhesion phenotype.
Further support of the role of inflammation in the development of the adhesion phenotype stems from the fact that there was a significant increase in basal cyclooxygenase (COX)-2 and PGE(2) levels in adhesion as compared to normal peritoneal fibroblasts. More importantly, hypoxia was found to increase COX-2 and PGE(2) levels in normal peritoneal fibroblasts but had no effect on adhesion fibroblasts. Inhibition of COX-2 by NS398 significantly reduced PGE(2) levels in both normal peritoneal and adhesion fibroblasts [Saed et al. Citation2005b]. Additionally, COX-2 inhibitors, such as celecoxib, tenoxicam, and pentoxifylline, have been reported to reduce postoperative adhesions through their anti-angiogenic, anti-inflammatory, and antioxidant effects in animal studies [Ezberci et al. Citation2006; Greene et al. Citation2005; Tarhan et al. Citation2006].
The findings of this study add to the molecular biological rationale for the process of adhesion development, and provide an opportunity for further in vivo and in vitro studies to reduce postoperative adhesion formation and reformation. Understanding how TNF-α and IL-6 function at the site of peritoneal injury and what factors modulate its activity will help delineate the mechanism of postoperative adhesion development, thereby revealing new pathways for pharmacological and/or biological modulation. Based on prior extensive studies characterizing the adhesion phenotype, and in view of these observations, we now hypothesize that hypoxia modulates TNF-α and IL-6 expression in normal peritoneal fibroblasts, thereby creating an inflammatory-like milieu, which in part contributes to postoperative adhesion [Alpay et al. Citation2008; Awonuga et al. Citation2009; Fletcher et al. Citation2008; Jiang et al. Citation2008; Saed and Diamond Citation2006; Saed et al. Citation2009; Saed et al. Citation2008b; Saed et al. Citation2010]. In support of this hypothesis, it was recently found that acute inflammation in the mouse peritoneal cavity was associated with increased incidence and severity of adhesion development [Corona et al. Citation2011]. Additionally, minimizing inflammation, which results from surgery, was also found to significantly reduce adhesion development [Pismensky et al. Citation2011; ten Broek et al. Citation2011].
In several previous studies, we have suggested that hypoxia, a result of vascular injury and loss, is a major contributor in the development of adhesions [Saed and Diamond Citation2002; Citation2004b; Saed et al. Citation2001]. Hypoxia leads to a coordinated series of molecular events that promote an inflammatory response leading to enhanced tissue fibrosis. The result of these alterations is an intracellular molecular milieu that favors adhesion development, we have termed the adhesion phenotype. Evidence for the association of the adhesion phenotype with the development of adhesions is supported by both animal and clinical studies [Ambler et al. Citation2012; Diamond et al. Citation2003; Freeman et al. Citation2003; Hershlag et al. Citation1991; Ivarsson et al. Citation2003; Saed et al. Citation2001]. Consistent with prior observations, this study has identified two additional markers that show increased levels of mRNA expression when exposed to hypoxia: the inflammatory cytokines IL-6 and TNF-α. Understanding the role of inflammation and hypoxia in the pathogenesis of postoperative adhesions will contribute future therapeutic interventions for the treatment of this disease.
Materials and Methods
Source and culture of human fibroblasts and mesothelial cells
Primary cultures of fibroblasts were established from normal peritoneal and adhesion tissues. Normal parietal peritoneal tissues from the anterior abdominal wall lateral to the midline incision were obtained from three patients who were undergoing laparotomy [Saed et al. Citation2004; Saed et al. Citation2002; Saed and Diamond Citation2004b]. The sampled normal peritoneum was, at a minimum, 3 in (7.62 cm) from any adhesions. The subjects did not have any active abdominal or pelvic infections and were not pregnant. Patients were given informed written consent to tissue collection, according to an approved protocol from the Wayne State University Institutional Review Board. Tissue samples were placed immediately in standard media (Dulbecco's Modified Eagle's Medium (DMEM) that contained 10% fetal bovine serum (FBS), 2% penicillin and streptomycin). Samples were then transferred into a T-25 flask with DMEM and put into an incubator at 37 °C (95 % air and 5 % CO2). Fibroblast growth generally took two w. When confluence was reached, cells were seeded into a 90-mm tissue culture dishes containing DMEM with 10% FBS. Cells utilized for this study were from passages three to five to maintain comparability.
Hypoxic culture conditions
All hypoxic experiments were performed as previously described [Caspi et al. Citation1979; Steege and Stout Citation1991]. Cultures were placed in an airtight Plexiglas chamber (Bello Glass, Vineland, NJ, USA) that was deoxygenated by positive infusion of 2% O2 in carbon dioxide-nitrogen gas mixture, for 24 h [Caspi et al. Citation1979; Steege and Stout Citation1991]. Although normal peritoneal tissue oxygen levels are known to be approximately 8%, our control cells were cultured in 20% O2 as we have previously found no differences in the expression of adhesion phenotype markers in an oxygen gradient range between 8 – 20% [Saed et al. Citation2005a; Saed et al. Citation2001]. Cells were then collected for RNA extraction.
RNA isolation and real-time reverse transcription–polymerase chain reaction (RT-PCR)
RNA isolation
Total RNA was extracted using the RNeasy Mini Kit (Qiagen, Valencia, CA, USA) per the manufacturer's protocol. The QuantiTect Reverse Transcription Kit (Qiagen, Valencia, CA, USA) provides a fast and convenient procedure for efficient reverse transcription and effective genomic DNA elimination. This kit is dedicated for use in real-time, two-step RT-PCR, and provides high cDNA yields for sensitive quantification of even low-abundance transcripts. The real-time RT-PCR method was used to measure mRNA levels of TNF-α and IL-6 in all samples.
Removal of DNA contamination from RNA
The purified RNA sample was briefly incubated in genomic DNA Wipeout Buffer and RNase-free water at 42°C for 2 min to effectively remove contaminating DNA. In contrast to other methods, the RNA sample was then used directly in reverse transcription.
cDNA synthesis
After gDNA elimination, the RNA sample is ready for reverse transcription using a master mix prepared from Quantiscipt Reverse Transcriptase, Quantiscript RT Buffer, and RT primer mix. The entire reaction takes place at 42°C and is the inactivated at 95°C. In contrast to other methods, additional steps for RNA denaturation, primer annealing and RNase H digestion are not necessary. Quantiscipt RT has a high affinity for RNA and is optimized for efficient and sensitive cDNA synthesis from 10 pg to 1 µg of RNA. This high RNA affinity, in combination with Quantiscript RT Buffer and RT primer mix, enables high cDNA yields.
PCR amplification
The QuantiTect SYBR Green RT-PCR Kit (Qiagen) and a Cepheid 1.2 f Detection System (Cepheid, Sunnyvale, CA, USA) were utilized to perform real-time RT-PCR as previously described [Saed et al. Citation2001]. The three-step polymerase chain reaction protocol applied for TNF-α and IL-6 reactions consisted of 35 cycles of 95°C for 15 s, 60°C (TNF- α and β-actin), and 55°C (IL-6), for 1 min and 72°C for 30 s. A melting curve analysis was performed to demonstrate the specificity of the PCR product as a single peak. A control, containing all the reaction components except for the cDNA template, was included in all experiments. mRNA was normalized to the housekeeping gene, β-actin.
Primer design and controls
Optimal oligonucleotide primer pairs for real-time RT-PCR amplification of oligo dT-primed reverse-transcribed cDNA were selected as previously described [Saed and Diamond Citation2006]. shows the human oligonucleotide primers used. Because quantitative application of this method is contingent on the analysis of the PCR products during amplification phase, prior to the plateau, cycle relationships and dilution curves for cDNA for each molecule and the housekeeping gene β-actin were determined.
Table 1. Human oligonucleotide primers for β-actin, IL-6, and TNF-α.
Statistical analysis
A Wilcoxsen Signed Ranks test for related samples was conducted using Statistical Package for Social Studies (SPSS Inc., Chicago, IL, USA) for Windows. Data are expressed as mean + SD. A p value < 0.05 was considered statistically significant.
Declaration of interest: This study was supported in part by grant number 1RO1 GM069941-01A3, National Institutes of Health, Bethesda, Maryland to Ghassan M. Saed. There are no conflicts of interest.
Author contributions: Conceived and designed experiment, wrote the manuscript: DA, GMS, MPD, NMF. Data analysis: DA, GMS; Performed the experiment: DA, NMF
Abbreviations:
IL: | = | interleukin |
TNF-α: | = | necrosis factor-alpha |
PGE(2): | = | prostaglandin E2 |
PAA: | = | plasminogen activator activity |
O2•−: | = | superoxide |
ONOO−: | = | peroxynitrite |
PAI-1: | = | plasminogen activator inhibitor-1 |
tPA: | = | tissue plasminogen activator |
COX-2: | = | cyclooxygenase-2 |
DMEM: | = | Dulbecco's Modified Eagle's Medium |
FBS: | = | fetal bovine serum. |
References
- Alpay, Z., Saed, G.M. and Diamond, M.P. (2008) Postoperative adhesions: from formation to prevention. Semin Reprod Med 26:313–321.
- Ambler, D.R., Golden, A.M., Gell, J.S., Saed, G.M., Carey, D.J. and Diamond, M.P. (2012) Microarray expression profiling in adhesion and normal peritoneal tissues. Fertil Steril 97:1158–1164 e1154.
- Atta, H.M. (2011) Prevention of peritoneal adhesions: a promising role for gene therapy. World J Gastroenterol 17:5049–5058.
- Awonuga, A.O., Saed, G.M. and Diamond, M.P. (2009) Laparoscopy in gynecologic surgery: adhesion development, prevention, and use of adjunctive therapies. Clin Obstet Gynecol 52:412–422.
- Badia, J. (1997) The peritoneal cytokine profile in acute peritonitis. Peritoneal Adhesions 197–200.
- Caspi, E., Halperin, Y. and Bukovsky, I. (1979) The importance of periadnexal adhesions in tubal reconstructive surgery for infertility. Fertil Steril 31:296–300.
- Cheong, Y.C., Laird, S.M., Li, T.C., Shelton, J.B., Ledger, W.L. and Cooke, I.D. (2001) Peritoneal healing and adhesion formation/reformation. Hum Reprod Update 7:556–566.
- Corona, R., Verguts, J., Schonman, R., Binda, M.M., Mailova, K. and Koninckx, P.R. (2011) Postoperative inflammation in the abdominal cavity increases adhesion formation in a laparoscopic mouse model. Fertil Steril 95:1224–1228.
- Diamond, M.P. (2001) Prevention of adhesions. In Operative gynecology, 2nd edn. Gershensopn, D.M and DeCherney, A.H. eds Saunders, Philadelphia, PA, USA 211–222.
- Diamond, M.P., El-Hammady, E., Wang, R. and Saed, G. (2003) Regulation of transforming growth factor-beta, type III collagen, and fibronectin by dichloroacetic acid in human fibroblasts from normal peritoneum and adhesions. Fertil Steril 79:1161–1167.
- Diamond, M.P. and Hershlag, A. (1990) Adhesion formation/reformation. Prog Clin Biol Res 358:23–33.
- diZerega, G.S. (1997) Biochemical events in peritoneal tissue repair. Eur J Surg Suppl 10–16.
- diZerega, G.S. and Campeau, J.D. (2001) Peritoneal repair and post-surgical adhesion formation. Hum Reprod Update 7:547–555.
- Ellis, H. (1997) The clinical significance of adhesions: focus on intestinal obstruction. Eur J Surg Suppl 5–9.
- Ezberci, F., Bulbuloglu, E., Ciragil, P., Gul, M., Kurutas, E.B., Bozkurt, S., (2006) Intraperitoneal tenoxicam to prevent abdominal adhesion formation in a rat peritonitis model. Surg Today 36:361–366.
- Fletcher, N.M., Jiang, Z.L., Diamond, M.P., Abu-Soud, H.M. and Saed, G.M. (2008) Hypoxia-generated superoxide induces the development of the adhesion phenotype. Free Radic Biol Med 45:530–536.
- Freeman, M.L., Saed, G.M., Elhammady, E.F. and Diamond, M.P. (2003) Expression of transforming growth factor beta isoform mRNA in injured peritoneum that healed with adhesions and without adhesions and in uninjured peritoneum. Fertil Steril 80 Suppl 2:708–713.
- Gomel, V., Urman, B. and Gurgan, T. (1996) Pathophysiology of adhesion formation and strategies for prevention. J Reprod Med 41:35–41.
- Greene, A.K., Alwayn, I.P., Nose, V., Flynn, E., Sampson, D., Zurakowski, D., (2005) Prevention of intra-abdominal adhesions using the antiangiogenic COX-2 inhibitor celecoxib. Ann Surg 242:140–146.
- Hershlag, A., Otterness, I.G., Bliven, M.L., Diamond, M.P. and Polan, M.L. (1991) The effect of interleukin-1 on adhesion formation in the rat. Am J Obstet Gynecol 165:771–774.
- Holmdahl, L. and Ivarsson, M.L. (1999) The role of cytokines, coagulation, and fibrinolysis in peritoneal tissue repair. Eur J Surg 165:1012–1019.
- Holmdahl, L., Risberg, B., Beck, D.E., Burns, J.W., Chegini, N., diZerega, G.S., (1997) Adhesions: pathogenesis and prevention-panel discussion and summary. Eur J Surg Suppl 56–62.
- Idell, S., Zwieb, C., Boggaram, J., Holiday, D., Johnson, A.R. and Raghu, G. (1992) Mechanisms of fibrin formation and lysis by human lung fibroblasts: influence of TGF-beta and TNF-alpha. Am J Physiol 263:L487–494.
- Imudia, A.N., Kumar, S., Saed, G.M. and Diamond, M.P. (2008) Pathogenesis of Intra-abdominal and pelvic adhesion development. Semin Reprod Med 26:289–297.
- Ivarsson, M.L., Diamond, M.P., Falk, P. and Holmdahl, L. (2003) Plasminogen activator/plasminogen activator inhibitor-1 and cytokine modulation by the PROACT System. Fertil Steril 79:987–992.
- Jiang, Z.L., Fletcher, N.M., Diamond, M.P., Abu-Soud, H.M. and Saed, G.M. (2009) S-nitrosylation of caspase-3 is the mechanism by which adhesion fibroblasts manifest lower apoptosis. Wound Repair Regen 17:224–229.
- Jiang, Z.L., Zhu, X., Diamond, M.P., Abu-Soud, H.M. and Saed, G.M. (2008) Nitric oxide synthase isoforms expression in fibroblasts isolated from human normal peritoneum and adhesion tissues. Fertil Steril.
- Lanfrancone, L., Boraschi, D., Ghiara, P., Falini, B., Grignani, F., Peri, G., (1992) Human peritoneal mesothelial cells produce many cytokines (granulocyte colony-stimulating factor [CSF], granulocyte-monocyte-CSF, macrophage-CSF, interleukin-1 [IL-1], and IL-6) and are activated and stimulated to grow by IL-1. Blood 80:2835–2842.
- Liakakos, T., Thomakos, N., Fine, P.M., Dervenis, C. and Young, R.L. (2001) Peritoneal adhesions: etiology, pathophysiology, and clinical significance. Recent advances in prevention and management. Dig Surg 18:260–273.
- Pismensky, S.V., Kalzhanov, Z.R., Eliseeva, M.Y., Kosmas, I.P. and Mynbaev, O.A. (2011) Severe inflammatory reaction induced by peritoneal trauma is the key driving mechanism of postoperative adhesion formation. BMC Surg 11:30.
- Saed, G.M., Abu-Soud, H.M. and Diamond, M.P. (2004) Role of nitric oxide in apoptosis of human peritoneal and adhesion fibroblasts after hypoxia. Fertil Steril 82 Suppl 3:1198–1205.
- Saed, G.M., Al-Hendy, A., Salama, S.A. and Diamond, M.P. (2008a) Adenovirus-mediated expression of cyclooxygenase-2 antisense reverse abnormal genetic profile of human adhesion fibroblasts. Fertil Steril 89(5 Suppl):1455–1460.
- Saed, G.M., Collins, K.L. and Diamond, M.P. (2002) Transforming growth factors beta1, beta2 and beta3 and their receptors are differentially expressed in human peritoneal fibroblasts in response to hypoxia. Am J Reprod Immunol 48:387–393.
- Saed, G.M. and Diamond, M.P. (2002) Hypoxia-induced irreversible up-regulation of type I collagen and transforming growth factor-beta1 in human peritoneal fibroblasts. Fertil Steril 78(1):144–147.
- Saed, G.M. and Diamond, M.P. (2003) Modulation of the expression of tissue plasminogen activator and its inhibitor by hypoxia in human peritoneal and adhesion fibroblasts. Fertil Steril 79(1):164–168.
- Saed, G.M. and Diamond, M.P. (2004a) Differential expression of alpha smooth muscle cell actin in human fibroblasts isolated from intraperitoneal adhesions and normal peritoneal tissues. Fertil Steril 82 Suppl 3:1188–1192.
- Saed, G.M. and Diamond, M.P. (2004b) Molecular characterization of postoperative adhesions: the adhesion phenotype. J Am Assoc Gynecol Laparosc 11(3):307–314.
- Saed, G.M. and Diamond, M.P. (2006) Effects of interferon-gamma reverse hypoxia-stimulated extracellular matrix expression in human peritoneal and adhesion fibroblasts. Fertil Steril 85 Suppl 1:1300–1305.
- Saed, G.M., Galijasevic, S., Diamond, M.P. and Abu-Soud, H.M. (2005a) Measurement of oxygen and nitric oxide levels in vitro and in vivo: relationship to postoperative adhesions. Fertil Steril 84:235–238.
- Saed, G.M., Jiang, Z., Diamond, M.P. and Abu-Soud, H.M. (2009) The role of myeloperoxidase in the pathogenesis of postoperative adhesions. Wound Repair Regen 17:531–539.
- Saed, G.M., Jiang, Z., Fletcher, N.M. and Diamond, M.P. (2008b) Modulation of the BCL-2/BAX ratio by interferon-gamma and hypoxia in human peritoneal and adhesion fibroblasts. Fertil Steril 90(5):1925–1930.
- Saed, G.M., Jiang, Z.L., Fletcher, N.M., Al Arab, A., Diamond, M.P. and Abu-Soud, H.M. (2010) Exposure to polychlorinated biphenyls enhances lipid peroxidation in human normal peritoneal and adhesion fibroblasts: a potential role for myeloperoxidase. Free Radic Biol Med 48:845–850.
- Saed, G.M., Munkarah, A.R., Abu-Soud, H.M. and Diamond, M.P. (2005b) Hypoxia upregulates cyclooxygenase-2 and prostaglandin E(2) levels in human peritoneal fibroblasts. Fertil Steril 83 Suppl 1:1216–1219.
- Saed, G.M., Munkarah, A.R. and Diamond, M.P. (2003) Cyclooxygenase-2 is expressed in human fibroblasts isolated from intraperitoneal adhesions but not from normal peritoneal tissues. Fertil Steril 79:1404–1408.
- Saed, G.M., Zhang, W., Chegini, N., Holmdahl, L. and Diamond, M.P. (2000) Transforming growth factor beta isoforms production by human peritoneal mesothelial cells after exposure to hypoxia. Am J Reprod Immunol 43:285–291.
- Saed, G.M., Zhang, W. and Diamond, M.P. (2001) Molecular characterization of fibroblasts isolated from human peritoneum and adhesions. Fertil Steril 75:763–768.
- Sandell, L.J. (1996) Genes and gene regulation of extracellular matrix proteins: an introduction. Connect Tissue Res 35:1–6.
- Steege, J.F. and Stout, A.L. (1991) Resolution of chronic pelvic pain after laparoscopic lysis of adhesions. Am J Obstet Gynecol 165:278–281; discussion 281–273.
- Tarhan, O.R., Barut, I., Sutcu, R., Akdeniz, Y. and Akturk, O. (2006) Pentoxifylline, a methyl xanthine derivative, reduces peritoneal adhesions and increases peritoneal fibrinolysis in rats. Tohoku J Exp Med 209:249–255.
- ten Broek, R.P., Wilbers, J. and van Goor, H. (2011) Electrocautery causes more ischemic peritoneal tissue damage than ultrasonic dissection. Surg Endosc 25:1827–1834.
- Topley, N., Jorres, A., Luttmann, W., Petersen, M.M., Lang, M.J., Thierauch, K.H., (1993) Human peritoneal mesothelial cells synthesize interleukin-6: induction by IL-1 beta and TNF alpha. Kidney Int 43:226–233.
- Whawell, S.A. and Thompson, J.N. (1995) Cytokine-induced release of plasminogen activator inhibitor-1 by human mesothelial cells. Eur J Surg 161:315–318.