Abstract
Growing oocytes are arrested at the first prophase of meiosis which is morphologically identified by the presence of a large and vesicular nucleus, called the germinal vesicle. The dissolution of the germinal vesicle marks the resumption of meiosis during which the oocyte undergoes massive modifications up to the second meiotic block, which is removed at fertilization. The interval between the first and the second meiotic block is defined as maturation and the events occurring during this period are crucial for ovulation, fertilization, and embryo development. Oocytes are excitable cells that react to stimuli by modifying their electrical properties as a consequence of ion currents flowing through ion channels on the plasma membrane. These electrical changes have been largely described at fertilization whereas little information is available during oocyte maturation. The aim of this review is to give an overview on the involvement of ion channels and ion currents during oocyte maturation in species from invertebrates to mammals. The results summarized here point to the possible functional role of ion channels underlying oocyte growth and maturation.
Introduction
Oocyte maturation
Oocyte maturation is a complex process occurring at the end of oogenesis during which the oocyte completes its growth and undergoes a series of changes that are necessary for ovulation, fertilization, and early embryo development. Oocyte maturation involves two different but interlinked processes involving nuclear and cytoplasmic events [Tsafriri Citation1979; Yanagimachi Citation1994; Eppig Citation1996].
Nuclear maturation is underpinned by the meiotic process that is arrested in most species, during neonatal life, at the diplotene of the first meiotic prophase (PI) and marked by the large nucleus known as germinal vesicle (GV) in which the chromosomes are in a decondensed state. The oocyte persists in this GV stage up to the time of a stimulus that resumes meiosis inducing the breakdown of the GV (GVBD), chromosome condensation, and spindle formation. A second meiotic arrest then occurs at different stages depending on the species such as the first meiotic metaphase (MI) in ascidians, bivalves, and gastropods, whereas mammalian oocytes complete the first round of meiosis with extrusion of the first polar body and without an interphase progress to the second meiotic metaphase (MII). Apart from some exceptions, this process is completed in almost all the species studied, upon fertilization, by the extrusion of the second polar body ().
Figure 1. A schematic illustration of the resumption of meiosis in different animal models. The immature oocyte is arrested in the prophase I (PI) marked by the germinal vesicle (GV). Depending on the species, oocytes may be fertilized in PI (anellids and starfish). In response to a stimulus, the first meiotic block is removed up to a second meiotic block at the metaphase I (MI; anellids, starfish, molluscs, and ascidians) or at metaphase II (MII; amphibians, fishes, mammals). MII is marked by the presence of one polar body (yellow). Resumption from the second meiotic block occurs upon sperm penetration.
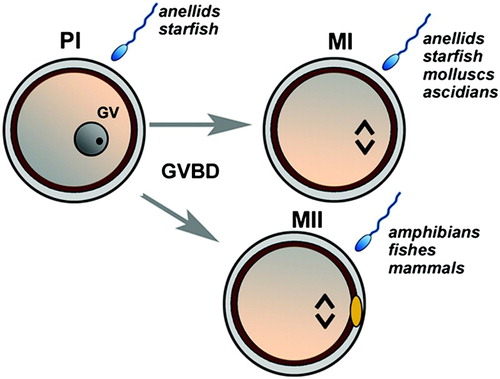
Cytoplasmic maturation is a less clear process that starts at the time of oocyte growth and can be evaluated by: i) ultrastructural organelle re-organization, ii) molecular modifications, and iii) differentiation of the calcium signalling machinery. Ultrastructural re-organization involves the redistribution of microfilaments and microtubules that in turn support the relocation of cytoplasmic organelles such as cortical granules and mitochondria [Schorderet-Slatkine Citation1972; Masui Citation1985; Iwamatsu et al. Citation1993; Voronina and Wessel Citation2003; Sun and Schatten Citation2006; Stricker Citation2006; Ferreira et al. Citation2009; Liu et al. Citation2010; De los Reyes et al. Citation2011].
Transient changes in cytoplasmic content of some molecules, such as cyclic adenosine mono-phosphate (cAMP) and some analogues, act either by preventing spontaneous maturation and/or blocking GVBD in vitro [Yoshimura et al. Citation1992; Stricker and Smythe 2001; Conti et al. Citation2002; Yi et al. Citation2002; Silvestre et al. Citation2011a; Tsafriri and Dekel Citation2011; Stricker et al. Citation2010]. Contrasting data, however, show that high levels of cAMP may transiently block GVBD [Kishimoto Citation1998] or even accompany GVBD [Lambert Citation2008; Citation2011; Yamashita et al. Citation2000]. A relevant role in promoting meiosis progression has been also attributed to M-phase promoting factor and cytostatic factors, shown for the first time in amphibian and, subsequently, in mammalian and invertebrate oocytes (for review see [Masui Citation2001; Russo et al. Citation2011]). Regulation of these molecular dynamics involves the integration of systemic and local inputs [Menezo and Elder Citation2011].
The rise in intracellular calcium at fertilization has been widely analyzed and assessed as the main signal involved in the oocyte activation [Malcuit and Fissore Citation2011]. To date, little information is available on the function of this ion during oocyte maturation; however, an integral attribute of oocyte maturation is the remodeling of calcium signaling pathways endowing the oocyte with the capacity to produce a specialized calcium transient at fertilization [Homa Citation1995; Carroll et al. Citation1996; Tosti Citation2006; Machaca Citation2007; Boni et al. Citation2007]. Recent studies have shown that cytoplasmic maturation is also accompanied by a modification of plasma membrane permeability due to ion currents flowing through ion channels located on the plasma membrane.
Ion currents
In the cell, the plasma membrane marks the borderline between the internal and external compartments and this separation is essential for cell functionality. The plasma membrane represents a barrier to ions whose passage occurs through the ion channels, specific proteins located in the lipid bilayer. Ion channels are characterized by their specificity, gating, conductance, and sensitivity to drugs, and their gating is triggered mainly in response to ligand-operated channels [Sutcliffe et al. Citation1998] or to a change in the voltage-operated channels [Terlau and Stuhmer Citation1998]. The passage of ions across the ion channels determines an electrical flux, which is called the ion current, that is associated with a change in the resting potential (RP). This is also due to the different distribution of electrical charges inside and outside the cell that creates an electrical gradient across the membrane that, in almost all cells studied, is negative, ranging from –10 mV to –100 mV [De Felice 1997]. Transient modification of the ionic concentration inside the cell shifts RP towards more positive values (depolarization) or toward more negative values (hyperpolarization). The main ion inside the cell are potassium cations whereas those externally are sodium and calcium cations. Due to its elective storage inside the cell, potassium is the ion that mainly contributes to determining and regulating the RP. This is an important parameter that, determining the steady state distribution of actively charged substances, plays a key role as a signal for many cellular processes controlled by voltage-sensitive ion channels. In comparison, sodium channels play a major role in supporting the action potentials [Stephan and Agnew Citation1991] and calcium channels are voltage-gated channels. These open in response to plasma membrane depolarization and have been shown to regulate a broad range of physiological processes (for a review see [Moosmang et al. Citation2005]).
The patch-clamp is the most popular technique used for the study of cell currents as first described by Neher and Sakmann [1992]. At present, whole-cell and single channel recordings are the most basic configurations of the patch clamp technique allowing the measurement of the activity and patterns of ion currents flowing throughout the plasma membrane.
Ion currents in invertebrate oocyte maturation
The role of ion currents in the physiology of oocytes has been described in many animal species. The oocyte is an excitable cell that during the crucial periods of maturation and subsequent fertilization and development shows transient modifications of the electrical asset due to ion currents throughout the plasma membrane [Hagiwara and Jaffe Citation1979; Tosti and Boni Citation2004; Tosti Citation2010]. Invertebrate oocytes have been extensively used for electrophysiological studies since the 1940s when Edward Chambers started to investigate sodium and potassium exchange during fertilization in the sea urchin (for review see [Chambers Citation1989]).
The mechanisms of reproduction of echinoderms have been extensively studied. In particular, starfish and sea urchin have been used to study the electrophysiological properties of the oocyte plasma membrane. In starfish, immature oocytes exhibit three types of voltage-dependent currents: an inward calcium, a fast transient potassium, and an inwardly rectifying potassium current. During the hormone-induced in vitro maturation, a gradual change in the amplitude of all three currents is observed; the calcium current becomes larger whereas both potassium currents become smaller [Moody and Lansman Citation1983; Moody and Bosma Citation1985]. These changes are also associated with a decrease in membrane conductance and a depolarization of RP due to changes in sodium and potassium conductance [Miyazaki et al. Citation1975a; Citation1975b; Moreau and Cheval Citation1976].
Interesting differences and similarities in the electrophysiological changes which occur during maturation in two different starfish (Henricia leviuscula and Asterina miniata) were described by Simoncini and Moody [1990] who found that oocytes of both species have the three major voltage-dependent currents. Apart from differences in the amplitudes, during maturation both potassium currents decreased in amplitude whereas the inward calcium currents remained constant in both species.
In the Leptasterias hexactis, two voltage-dependent potassium currents were found early in oogenesis, before the rapid growth phase, and remained stable throughout oogenesis at the same current density and kinetics. As soon as the oocyte reached its full size, the inward current increased in amplitude by about fivefold, and underwent major changes in kinetics. These changes were closely associated with the migration of the GV to the cell periphery that represents the major morphological event at maturation [Moody Citation1985]. Electrical modifications of the oocyte plasma membrane have been previously described in sea urchin by Dale and De Santis [1981]. These authors described how the high potassium permeability of immature oocytes progressively decreases along with maturation. In both starfish and sea urchin, however, the functional role of these currents has not been elucidated.
Ascidians are invertebrates largely used for studying gamete biology. Their gametes are, in fact, very suitable for electrophysiological analysis of channel function, since their extracellular membranes are easily removed leaving the nude membrane accessible for the patch clamp. In the ascidian Ciona intestinalis, ion currents show typical features characterized by inward and outward currents that follow each other during oocyte maturation. This suggests that each current may play an important role during either nuclear or cytoplasmic maturation.
By using the whole-cell voltage clamp technique, we have studied ion currents in the ascidian Ciona intestinalis oocyte plasma membranes during different stages of growth and meiosis [Tosti et al. Citation2011]. First, Cuomo et al. [2006] showed that immature oocytes underwent a spontaneous GVBD in the presence of calcium. The fact that calcium plays a role in prophase/metaphase transition was also supported by a higher calcium current activity found at the GV stage in comparison to the following meiotic stages where sodium currents start to appear (). Subsequently, we identified three stages of immature oocytes with different electrical features. The smallest oocyte class exhibited the highest L-type calcium current activity and was incompetent for meiosis resumption whereas the larger oocyte classes showed the first occurrence of sodium currents that remained high throughout maturation up to the post-vitellogenic stage [Silvestre et al. Citation2009]. L-type calcium channels, however, do not represent the unique type of calcium channels found in ascidian oocytes that may play a role during oogenesis. Recently, in other ascidian species, we observed, for the first time in oocytes, T-type calcium channels [Gallo et al. 2012], which are typically involved in sperm physiology [Jagannathan et al. Citation2002]. This novel finding suggests new insights for the mechanism of calcium currents during oocyte maturation.
Figure 2. Ion current patterns during oocyte maturation of Ciona intestinalis. L-type calcium current activity is high at the GV stage and decreases during maturation. Sodium currents first appear in mature oocytes and remain high up to the zygote.
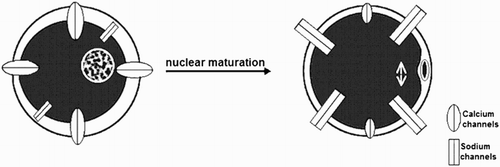
In mollusks, the influence of calcium entry through the plasma membrane channels has been related to nuclear maturation. In Spisula, nuclear maturation requires external calcium influx through voltage-gated channels in order to undergo GVBD. This is also the case for the oocytes of the surf clam, Ruditapes and Crassostrea [Dubè Citation1992; Leclerc et al. Citation2000]. The present hypothesis is that an early calcium influx is a prerequisite to allow nuclear maturation in either PI- [Colas and Dubè 1998] or MI-arrested oocytes of bivalves [Moreau et al. Citation1996]. This current seems to be mediated by L-type calcium channels [Guerrier et al. Citation1993; Tomkowiak et al. Citation1997].
Consistent with these findings, in Octopus vulgaris the accurate electrical characterization of the plasma membrane electrical proprieties of immature oocytes at GV stage showed that L-type calcium currents are higher in small pre-vitellogenic oocytes and significantly lower in early-vitellogenic oocytes. Similarly, a significant decrease of the steady-state conductance occurred from the pre- to early-vitellogenic oocytes indicating that ion and L-type calcium currents play a role in oocyte growth and cytoplasmic maturation, and may be involved in preparing the plasma membrane for the interaction with the spermatozoon [Cuomo et al. Citation2005].
Ion currents in vertebrate oocyte maturation
Amphibians
Amphibian oocytes exhibit peculiar electrical properties, such as the involvement of chloride channels and sensitivity to neurotransmitters [Jaffe and Cross Citation1984]. In Xenopus laevis, the ability to respond to neurotransmitters is related to chloride channels which generate a steady current in the early immature oocyte. Earlier studies by Robinson [1979] suggested that the electric field generated by chloride channels may serve to localize components in the growing oocyte and, more generally, these electrical currents might play a role in the establishment of cell polarity during oogenesis. In Xenopus, voltage-sensitive ion channels, selective for sodium and capable of generating action potentials, were shown to first appear during oocyte growth [Baud Citation1983]. Recently, the ability of chloride channels to induce GVBD was shown using molecular biology techniques [Reyes et al. Citation2009].
The coordinated action of chloride and sodium channels appears to modulate oocyte maturation in Rana pipiens in which chloride channels disappear throughout maturation; in contrast, the sodium channels remain active up to the full maturity of the oocyte [Baud Citation1983]. The precise timing of the appearance and disappearance of chloride channels and the increasing activity of sodium channels during oocyte growth suggested that they might play a regulatory role in maturation [Schlichter Citation1983]. It is well established that progesterone releases amphibian oocytes from the meiotic block and stimulates meiosis progression up to the mature stage [Maller Citation1985]. In Ambystoma, an interplay between the fluctuation of sodium currents and decreased hydrogen ion conductance was described during the progesterone-induced oocyte maturation [Baud and Barish Citation1984]. These findings further support the hypothesis that progesterone induced GVBD via a down-regulation of the membrane sodium-potassium ATPase and, hence, influence the ion permeability and transport properties of both nuclear and plasma membranes [Weinstein et al. Citation1982].
In the European frog Rana esculenta, together with chloride and sodium activity, voltage-dependent potassium current changes occur during oocyte maturation. These changes are considered relevant for successful fertilization [Taglietti et al. Citation1984]. By accurate electrophysiological recording in Rana perezi, three different types of chloride currents have been characterized during oocyte growth [Ivorra and Morales Citation1997]. These authors definitively suggested that the presence of most of these infrequent currents in immature oocytes could not be merely the result of random genomic expression but a programmed decision, probably related to a definite functional role in the maturation process.
In Xenopus, there is substantial support for a role for calcium, and its downstream effectors, i.e., calmodulin and calcium-calmodulin-dependent protein kinase II, in mitosis initiation and progression [Means Citation1994; Whitaker Citation1995]. Calcium signals are required for GVBD and chromosome condensation during mitosis [Steinhardt and Alderton Citation1988; Tombes et al. Citation1992; Ciapa et al. Citation1994]. In contrast to the well-defined roles for calcium signals in mitosis and after fertilization [Stricker Citation2000], the role of intracellular calcium signals during oocyte maturation remains contentious. On one hand, early reports suggested that the rise in calcium is sufficient to induce oocyte maturation [Wasserman and Masui Citation1975; Moreau et al. Citation1976]. On the other hand, oocytes treated with calcium buffers were unable to mature [Duesbery and Masui Citation1996] and injection of inositol 1,4,5-trisphosphate (IP3), which induces calcium release, did not stimulate meiotic maturation [Picard et al. Citation1985].
Distinct sources of calcium signals can be generated either due to calcium release from intracellular calcium stores or calcium influx from the extracellular compartment. These two calcium sources are mechanistically linked through the store-operated calcium channels (SOCs), which are activated in response to the depletion of calcium stores. The role of SOCs and their interaction with stromal interaction molecule 1 (STIM1), a protein involved in intracellular calcium dynamics from intracellular stores, is still controversial [Worley et al. Citation2007; DeHaven et al. Citation2009]. Some of the molecular components of SOCs have been defined. It is known that STIM1 works as the endoplasmic calcium sensor [Liou et al. Citation2005; Roos et al. Citation2005], and Orai family proteins work as the pore-forming subunits of SOCs. These channels are known as the calcium release-activated calcium channels [Feske et al. Citation2006; Vig et al. Citation2006; Zhang et al. Citation2006]. A significant percentage of total Orai1 is intracellular at steady state. The depletion of stores completely shifts endosomal Orai1 to the cell membrane [Yu et al. Citation2010]. In Xenopus oocytes, it has been shown that calcium entry through SOCs, operated by STIM1/Orai1, was responsible for filling the endoplasmic reticulum during maturation [Machaca and Haun Citation2000]. However, by manipulating intracellular calcium store load and the extent of calcium influx through SOCs, Sun and Machaca [2004] showed that calcium signals were not required for entry into meiosis. On the contrary, high intracellular calcium delays meiosis entry. Moreover, in the absence of calcium signals oocytes arrest in MI, form abnormal spindles, and do not extrude the polar body.
Mammals
In mammals, oocyte maturation may be defined as a process occurring between the first and the second meiotic block that renders the oocyte able to fertilize and to support the early stages of embryonic development. During this time, a number of events occur in which the oocyte plasma membrane plays a crucial role. Before reaching maturity, the mammalian oocyte does not operate as an independent cell. A close cooperation between the oocyte and the surrounding cumulus cells assures the passage of metabolites allowing and/or driving oocyte growth and, finally, maturation. The cumulus cells transmit stimuli to the oocyte, such as those involved in maintaining or removing meiotic arrest (for review see [Silvestre et al. Citation2011b]). In the former, the oocyte RP is maintained under hyperpolarized conditions, whereas in the latter, depolarization occurs as a consequence of the action of gonadotropins on the cumulus cells [Mattioli et al. Citation1998], leading to the progression of meiosis. Notwithstanding this interaction, cumulus cells and oocytes do maintain different membrane potentials in spite of the existence of low resistance channels, such as gap junctions [Emery et al. Citation2001]. Depolarization of granulosa cells was addressed as an essential feature of follicular maturation; this hypothesis is supported by the finding that differential granulosa cell proliferation, steroidogenic capability, and apoptosis can be influenced by selective antagonism of granulosa cell potassium channels [Manikkam et al. Citation2002]. The interaction between cumulus cells and the oocyte decreases at the end of maturation, together with cumulus expansion and the breaking of heterologous gap junction communications. However, this is not accompanied by a simultaneous decrease in electrical coupling [Racowsky and Setterlie 1987]. In bovine oocytes, we found RP variations during the progression of meiosis [Tosti et al. Citation2000]. In particular, after a preliminary hyperpolarization at the GVBD stage, RP was depolarized. The opposite pattern has also been recorded in relation to plasma membrane permeability, measured as steady-state conductance [Tosti et al. Citation2000; Murnane and De Felice Citation1993]. This parameter is high at the GV stage and decreases during meiosis with little restoration at the mature (MII) stage. Hence, the highest membrane permeability corresponds to the two meiotic arrest phases. It is feasible that the large ion exchange is related to the large metabolic activity of the GV stage or to the preparation of the plasma membrane for fertilization at the MII stage. During maturation, relevant ion dynamics occur through the oocyte plasma membrane due to either an exchange between intra- and extra-cellular compartments or intercellular communications.
Two-pore domain potassium (K2P) channels that help to set the resting membrane potential have been localized on the plasma membrane of bovine oocytes and may contribute to the electrical activities of the gamete plasma membrane during maturation [Hur et al. Citation2009]. ATP-sensitive potassium (K+ATP) channels have been found in human, bovine, and porcine oocytes at various maturational stages [Du et al. Citation2010]. In any tissues where they are expressed, K+ATP channels regulate membrane potential and, at the same time, affect intracellular calcium levels (reviewed by [Nichols Citation2006]). The link established by these channels between intracellular metabolism, membrane excitability and intracellular calcium signaling suggests a potential role in infertility due to stress injuries or metabolic diseases.
Voltage-dependent anion channels, which are reputed to play a key role in mitochondrial metabolism and ion signalling, have been recently found on the ooplasma membrane of porcine oocytes [Cassarà et al. 2009]. They are arranged as ring-like clusters of structures distributed on the cortical area at GV, but not at MII, stage oocytes. Either their localization or their absence at the end of maturation suggests a functional role during maturation and fertilization of mammalian oocytes.
Another relevant propriety of the plasma membrane is to allow the movement and the proper regulation of fluids between the internal and external side of the cell. The transport of water primarily occurs by simple diffusion through the lipid bilayer membrane [Verkman et al. Citation1996]. However, in somatic cells high water permeability cannot be explained by simple diffusion because the lipid bilayer is hydrophobic [Preston et al. Citation1993]. Aquaporins (AQPs) are a family of small membrane spanning monomer and hydrophobic integral membrane channel proteins that are expressed in plasma membranes of many cell types requiring fluid transport [Carbrey and Agre Citation2009]. In mouse oocytes, the Aqp3 mRNA is expressed in immature oocytes and decreases after either in vitro or in vivo maturation [Jo et al. Citation2011] suggesting a potential role of these channels in underlying correct maturation progress. In addition, Yamaji et al. [2011] improved the tolerance of the in vitro matured oocytes to vitrification with a glycerol-based solution by injecting AQP3 RNA in immature oocytes.
The role of calcium in oocyte maturation is still a matter of debate [Boni et al. Citation2007]. Based on the observation that cytoplasmic IP3 receptors increase during meiotic progression, IP3-induced calcium release has been suggested as a primary mechanism for maturation of bovine oocytes. A minor role has been attributed to calcium release through ryanodine receptors, which are poorly expressed in bovine oocytes [He et al. Citation1997]. In the hamster and mouse, a series of spontaneous calcium oscillations occur in the oocyte after isolation from the follicle up to the GVBD stage [Fujiwara et al. Citation1993; Carroll et al. Citation1995]. After these oscillations have subsided, calcium does not affect further meiotic progression. In bovine and pig, no calcium oscillations occur during meiotic progression; however, calcium is necessary for meiotic progression since BAPTA, a calcium chelator, causes a delay or block of meiosis [Fujiwara et al. Citation1993; He et al. Citation1997]. In addition, the calcium ionophore, A-23187, induces resumption of meiosis in oocytes blocked either at the GV [Wasserman and Masui Citation1975] or MII stage [Boni et al. Citation2002]. Because extracellular calcium is required for in vitro GVBD [De Felici and Siracusa 1982] and for the first meiotic division [Paleos and Powers Citation1981], calcium ion transport through the plasma membrane seems to play a functional role in maturation. Extracellular calcium plays a role in the progression of meiosis. In bovine, we have shown that: i) calcium depletion in the maturation medium severely affects oocyte developmental competence, ii) the activity of plasma membrane L-type calcium channel decreases during maturation, and iii) the exposure to specific inhibitors decreases in vitro maturation efficiency [Tosti et al. Citation2002]. In addition: i) BAPTA delays kinase activity and inhibits maturation [He et al. Citation1997], ii) calcium may influence protein synthesis that is essential for maturation [Levesque and Sirard Citation1996], and iii) calcium modulates gap junction functionality, allowing the drop in cumulus-mediated [cAMP]i levels [Yoshimura et al. Citation1992]. As a consequence of a net uptake of calcium through the plasma membrane, at the end of maturation, the oocyte achieves an adequate store of calcium. This storage may occur via either gap junctions or specific channels. Electrophysiological and pharmacological studies strongly suggest that ion currents underlying bovine oocyte maturation are L-type calcium channels [Tosti et al. Citation2000] whose activity decreases throughout meiotic progression. In mouse oocytes, the activity of these channels increases after puberty [Murnane and De Felice Citation1993], suggesting a role for these channels in meiotic competence.
The STIM1 and Orai1 proteins are components of the plasma membrane-resident calcium channel that are responsible for mediating calcium entry after the mobilization of intracellular calcium. Recently, they have been identified in pig and mouse oocytes and their involvement in store-operated calcium entry has also been demonstrated [Gomez-Fernandez et al. Citation2009; Koh et al. Citation2009; Yu et al. Citation2009]. In porcine, Orai1 expression decreased during oocyte maturation along with the increasing ability to generate a large calcium influx after store depletion [Wang et al. Citation2012]. In addition, down-regulation of Orai1 expression by siRNA microinjection blocked calcium influx after store depletion and subsequent calcium add-back. Over-expression of Orai1 in the oocytes modified store-operated calcium entry and had an inhibitory effect on the calcium fertilization signal. Co-over-expression of Orai1 and STIM1 led to a dramatic increase in calcium entry after store depletion [Wang et al. Citation2012].
As above reviewed, the role played by ion currents in oocyte maturation is still unclear. However, the change in plasma membrane electrical properties appear to underlie oocyte growth, meiosis progression, and the preparation of the oocyte for fertilization in all the animals studied. This information may help to develop new culture systems more adequate to the oocyte's physiological needs for proper maturation.
Declaration of interest: The authors have declared that no competing interests exist.
Abbreviations
PI: | = | first meiotic prophase |
GV: | = | germinal vesicle |
GVBD: | = | breakdown of the GV |
MI: | = | first meiotic metaphase |
MII: | = | second meiotic metaphase |
cAMP: | = | cyclic adenosine mono-phosphate |
RP: | = | resting potential |
IP3: | = | inositol 1,4,5-trisphosphate |
SOCs: | = | store-operated calcium channels |
STIM1: | = | stromal interaction molecule 1 |
K+ATP: | = | ATP-sensitive potassium |
AQPs: | = | aquaporins |
BAPTA: | = | bis-(o-aminophenoxy)-ethane-N,N,N′,N′-tetraacetic acid. |
References
- Baud, C. (1983) Developmental change of a depolarization induced sodium permeability in the oocyte of Xenopus laevis. Dev Biol 99:524–528.
- Baud, C. and Barish, M.E. (1984) Changes in membrane hydrogen and sodium conductances during progesterone-induced maturation of Ambystoma oocytes. Dev Biol 105:423–434.
- Boni, R., Cuomo, A. and Tosti, E. (2002) Developmental potential in bovine oocytes is related to cumulus-oocyte complex COC grade, calcium current activity and calcium stores. Biol Reprod 66:836–842.
- Boni, R., Gualtieri, R., Talevi, R. and Tosti, E. (2007) Calcium and other ion dynamics during gamete maturation and fertilization. Theriogenology 68 Suppl 1:S156–164.
- Carbrey, J.M. and Agre, P. (2009) Discovery of the aquaporins and development of the field. Handb Exp Pharmacol 190:3–28.
- Carroll, J., Swann, K., Whittingham, D.J. and Whitaker, M. (1995) Spatiotemporal dynamics of intracellular (Ca2+)i oscillations during the growth and meiotic maturation of mouse oocytes. Development 120:3507–3517.
- Carroll, J., Jones, K.T. and Whittingham, D. (1996) Ca2+ release and the development of Ca2+ release mechanisms during oocyte maturation: a prelude to fertilization. Rev Reprod 1:137–143.
- Cassará, M.C., Menzel, V.A., Hinsch, K.D., Wrenzycki, C. and Hinsch, E. (2009) Voltage-dependent anion channels 1 and 2 are expressed in porcine oocytes. Biosci Rep 30:193–200.
- Chambers, E.L. (1989) An autobiographical sketch. Mechanism of egg activation Nuccitelli, R.Cherr, G. and Clark, W.H.Jr. Plenum Press, NY, pp.15–28.
- Ciapa, B., Pesando, D., Wilding, M. and Whitaker, M. (1994) Cell-cycle calcium transients driven by cyclic changes in inositol trisphosphate levels. Nature 368:875–878.
- Colas, P. and Dubé, F. (1998) Meiotic maturation in mollusc oocytes. Sem Cell Dev Biol 9:539–548.
- Conti, M., Andersen, C.B., Richard, F., Mehats, C., Chun, S.Y. Horner, K., (2002) Role of cyclic nucleotide signaling in oocyte maturation. Mol Cell Endocrinol 18:153–189.
- Cuomo, A., Di Cristo, C., Di Cosmo, A., Paolucci, M. and Tosti, E. (2005) Calcium currents correlate with oocyte maturation during the reproductive cycle in Octopus vulgaris. J Exp Zool A 303:193–202.
- Cuomo, A., Silvestre, F., De Santis, R. and Tosti, E. (2006) Ca2+ and Na+ current patterns during oocyte maturation, fertilization, and early developmental stages of Ciona intestinalis. Mol Reprod Dev 73:501–511.
- Dale, B. and De Santis, A. (1981) Maturation and fertilization of the sea urchin oocyte: an electrophysiological study. Dev Biol 85: 474-484.
- DeFelice, L.J. (1997) Bioelectricity. Electrical properties of the cell: Patch clamp for biologists. Plenum Press, NY, pp.49–122.
- DeFelici, M. and Siracusa, G. (1982) Survival of isolated, fully grown mouse ovarian oocytes is strictly dependent on external Ca2+. Dev Biol 92:539–543.
- DeHaven, W.I., Jones, B.F., Petranka, J.G., Smyth, J.T., Tomita, T., Bird, G.S. and Putney, J.W.Jr. (2009) TRPC channels function independently of STIM1 and Orai1. J Physiol 587:2275–2298.
- De los Reyes, M., Palomino, J., Parraguez, V.H., Hidalgo, M. and Saffie, P. (2011) Mitochondrial distribution and meiotic progression in canine oocytes during in vivo and in vitro maturation. Theriogenology 75(2): p346-353.
- Du, Q., Jovanović, S., Sukhodub, A., Barratt, E., Drew, E., Whalley, K.M., (2010) Human oocytes express ATP-sensitive K(+) channels. Hum Reprod 25:2774–2782.
- Dubè, F. (1992) Thapsigargin induces meiotic maturation in surf clam oocytes. Biochem Biophys Res Commun 189:79–84.
- Duesbery, N.S., and Masui, Y. (1996) The role of Ca2+ in progesterone-induced germinal vesicle breakdown of Xenopus laevis oocytes: the synergic effects of microtubule depolymerization and Ca2+. Dev Genes Evol 206:110–124.
- Emery, B.R., Miller, R.L. and Carrell, D.T. (2001) Hamster oocyte membrane potential and ion permeability vary with preantral cumulus cell attachment and developmental stage. BMC Dev Biol 1:14.
- Eppig, J.J. (1996) Coordination of nuclear and cytoplasmic oocyte maturation in eutherian mammals. Reprod Fertil Dev 8:485–489.
- Ferreira, E.M., Vireque, A.A., Adona, P.R., Meirelles, F.V., Ferriani, R.A. and Navarro, P.A. (2009) Cytoplasmic maturation of bovine oocytes: Structural and biochemical modifications and acquisition of developmental competence. Theriogenology 71:836–848.
- Feske, S., Gwack, Y., Prakriya, M., Srikanth, S., Puppel, S.H., Tanasa, B., (2006) A mutation in Orai1 causes immune deficiency by abrogating CRAC channel function. Nature 441:179–185.
- Fujiwara, T., Nakada, K., Shirakawa, H. and Miyazaki, S. (1993) Development of inositol trisphosphate-induced calcium release mechanism during maturation of hamster oocytes. Dev Biol 156:69–79.
- Gallo, A., Russo, G.L. and Tosti, E. (2012) T-type Ca2+ current activity during oocyte growth and maturation in the ascidian Styela plicata. PloS One, in press.
- Gomez-Fernandez, C., Pozo-Guisado, E., Ganan-Parra, M., Perianes, M.J., Alvarez, I.S. and Martin-Romero, F.J. (2009) Relocalization of STIM1 in mouse oocytes at fertilization: early involvement of store-operated calcium entry. Reproduction 138:211–221.
- Guerrier, P., Leclerc-David, C. and Moreau, M. (1993) Evidence for the involvement of internal calcium stores during serotonin-induced meiosis reinitation in oocytes of the bivalve mollusc Ruditapes philippinarum. Dev Biol 159:474–484.
- Hagiwara, S. and Jaffe, L.A. (1979) Electrical properties of egg cell membranes. Annu Rev Biophys Bioeng 8:385–416.
- He, C.L., Damiani, P., Parys, J.B. and Fissore, R.A. (1997) Calcium, calcium release receptors, and meiotic resumption in bovine oocytes. Biol Reprod 57:1245–1255.
- Homa, S. (1995) Calcium and meiotic maturation of the mammalian oocyte. Mol Repr Dev 40:122–134.
- Hur, C.G., Choe, C., Kim, G.T., Cho, S.K., Park, J.Y., Hong, S.G., (2009) Expression and localization of two-pore domain K(+)channels in bovine germ cells. Reproduction 137:237–244.
- Ivorra, I. and Morales, A. (1997) Membrane currents in immature oocytes of the Rana perezi frog. Pflugers Arch 434:413–421.
- Iwamatsu, T., Toya, Y., Sakai, N., Terada, Y., Nagata, R. and Nagahama, Y. (1993) Effect of 5- Hydroxytryptamine on steroidogenesis and oocyte maturation in pre-ovulatory follicles of the medaka Oryzias latipes. Dev Growth Differ 35:625–630.
- Jaffe, L. and Cross, N. (1984) Electrical Properties of Vertebrate Oocyte Membranes. Biol Reprod 30:50–54.
- Jagannathan, S., Publicover, S.J. and Barratt, C.L.. (2002) Voltage-operated calcium channels in male germ cells. Reproduction 123:203–215.
- Jo, J.W., Jee, B.C., Suh, C.S., Kim, S.H., Choi, Y.M., Kim, J.G., (2011) Effect of maturation on the expression of aquaporin 3 in mouse oocyte. Zygote 19:9–14.
- Kishimoto, T. (1998) Cell cycle arrest and release in starfish oocytes and eggs. Sem Cell Dev Biol 9:549–557.
- Koh, S., Lee, K., Wang, C., Cabot, R.A. and Machaty, Z. (2009) STIM1 regulates store-operated Ca2+ entry in oocytes. Dev Biol 330:368–376.
- Lambert, C.C. (2008) Signaling pathways in ascidian oocyte maturation: the role of cyclic AMP and follicle cells in germinal vesicle breakdown. Dev Growth Differ 50(3):181–188.
- Lambert, C.C. (2011) Signaling pathways in ascidian oocyte maturation: The roles of cAMP/Epac, intracellular calcium levels, and calmodulin kinase in regulating GVBD. Mol Reprod Dev 78:726–733.
- Leclerc, C., Guerrier, P. and Moreau, M. (2000) Role of dihydropyridine-sensitive calcium channels in meiosis and fertilization in the bivalve molluscs Ruditapes philippinarum and Crassostrea gigas. Biol Cell 92:285–299.
- Levesque, J.T. and Sirard, M.A. (1996) Resumption of meiosis is initiated by the accumulation of cyclin B in bovine oocytes. Biol Reprod 55:1427–1436.
- Liou, J., Kim, M.L., Heo, W.D., Jones, J.T., Myers, J.W., Ferrell, J.E.Jr. and Meyer, T. (2005) STIM is a Ca2+ sensor essential for Ca2+-store-depletion-triggered Ca2+ influx. Curr Bioll 5:1235–1241.
- Liu, S., Li, Y., Feng, H.L., Yan, J.H., Li, M., Ma, S.Y., (2010) Dynamic modulation of cytoskeleton during in vitro maturation in human oocytes. Am J Obstet Gynecol 203:151. e1-7
- Machaca, K. (2007) Ca2+ signaling differentiation during oocyte maturation. J Cell Physiol 213:331–340.
- Machaca, K. and Haun, S. (2000) Store-operated calcium entry inactivates at the germinal vesicle breakdown stage of Xenopus meiosis. J Biol Chem 275:38710–38715.
- Malcuit, C. and Fissore, R.A. (2011) Recent advances in the understanding of the molecular effectors of mammalian egg activation. In Oocyte maturation and fertilization: A long history for a short event ed. Tosti, E. and Boni, R.. Bentham Science Publisher Ltd, Dubai, pp.121–134.
- Maller, J.L. (1985) Oocyte maturation in amphibians. Dev Biol 1:289–311.
- Manikkam, M., Li, Y., Mitchell, B.M., Mason, D.E. and Freeman, L.C. (2002) Potassium channel antagonists influence porcine granulosa cell proliferation, differentiation, and apoptosis. Biol Reprod 67:88–98.
- Masui, Y. (1985) Meiotic arrest in animal oocytes. In Biology of Fertilization volume 1 ed. Metz, C.B. and Monroy, A. Academic Press, NY, pp.189–219.
- Masui, Y. (2001) From oocyte maturation to the in vitro cell cycle: the history of discoveries of Maturation-Promoting Factor (MPF) and Cytostatic Factor (CSF). Differentiation 69:1–17.
- Mattioli, M., Gioia, L. and Barboni, B. (1998) Calcium elevation in sheep cumulus-oocyte complexes after luteinizing hormone stimulation. Mol Reprod Dev 50:361–369.
- Means, A.R. (1994) Calcium, calmodulin and cell cycle regulation. FEBS Lett 347:1–4.
- Menezo, Y.R. and Elder, K. (2011) The enhancer of oocyte competence. Oocyte maturation and fertilization: A Long History for a Short event ed. Tosti, E. and Boni, R. Bentham Science Publisher Ltd, Dubai, pp.64–70.
- Miyazaki, S., Ohmori, H. and Sasaki, S. (1975a) Action potential and non-linear current-voltage relation in starfish oocytes. J Physiol (Lond) 246:37–54.
- Miyazaki, S., Ohmori, H. and Sasaki, S. (1975b) Potassium rectifications of the starfish oocyte membrane and their changes during oocyte maturation. J Physiol (Lond) 246:55–78.
- Moody, W.J. (1985) The development of calcium and potassium currents during oogenesis in the starfish, Leptasterias hexactis. Dev Biol 112(2):405–413.
- Moody, W.J. and Lansman, J.B. (1983) Developmental regulation of Ca2+ and K+ currents during hormone-induced maturation of starfish oocytes. Proc Natl Acad Sci USA 80:3096–3100.
- Moody, W.J. and Bosma, M.M. (1985) Hormone-induced loss of surface membrane during maturation of starfish oocytes: differential effects on potassium and calcium channels. Dev Biol 112(2):396–404.
- Moreau, M. and Cheval, J. (1976) Electrical properties of the starfish oocyte membranes. J Physiol 72:293–300.
- Moreau, M., Doree, M. and Guerrier, P. (1976) Electrophoretic introduction of calcium into the cortex of Xenopus laevis oocytes triggers meiosis reinitiation. J Exp Zool 197:443–449.
- Moreau, M., Leclerc, C. and Guerrier, P. (1996) Meiosis reinitiation in Ruditapes philippinarum (Mollusca): involvement of L-calcium channels in the release of metaphase I block. Zygote 4:151–157.
- Moosmang, S., Lenhardt, P., Haider, N., Hofmann, F. and Wegener, J.W. (2005) Mouse models to study L-type calcium channel function. Pharmacol Ther 106:347–355.
- Murnane, J. and De Felice, L.J. (1993) Electrical maturation of murine oocytes: an increase in calcium current coincides with acquisition of meiotic competence. Zygote 1:49–60.
- Neher, E. and Sakmann, B. (1992) The patch-clamp technique. Sci Am 266:44–51.
- Nichols, C.G. (2006) KATP channels as molecular sensors of cellular metabolism. Nature 440:470–476.
- Paleos, G.A. and Powers, R.D. (1981) The effect of calcium on the first meiotic division of the mammalian oocyte. J Exp Zool 217:409–416.
- Picard, A., Giraud, F., Le Bouffant, F., Sladeczek, F., Le Peuch, C. and Doree, M. (1985) Inositol 1,4,5-trisphosphate microinjection triggers activation, but not meiotic maturation in amphibian and starfish oocytes. FEBS Lett 182:446–450.
- Preston, G.M., Jung, J.S., Guggino, W.B. and Agre, P. (1993) The mercury-sensitive residue at cysteine 189 in the CHIP28 water channel. J Biol Chem 268:17–20.
- Racowsky, C. and Satterlie, R.A. (1987) Decreases in heterologous metabolic and dye coupling, but not in the electrical coupling, accompany meiotic resumption in hamster oocyte cumulus complexes. Eur J Cell Biol 43:283–292.
- Reyes, R., Pulakat, L., Miledi, R. and Martínez-Torres, A. (2009) Mammalian AT2 receptors expressed in Xenopus laevis oocytes couple to endogenous chloride channels and stimulate germinal vesicle break down. Cell Physiol Biochem 24:45–52.
- Robinson, K.R.. (1979). Electrical currents through full-grown and maturing Xenopus oocytes. Proc Nat Acad Sci USA 76:837–841.
- Roos, J., DiGregorio, P.J., Yeromin, A.V., Ohlsen, K., Lioudyno, M., Zhang, S., (2005) STIM1, an essential and conserved component of store-operated Ca2+ channel function. J. Cell Biol 1 69:435–445.
- Russo, G.L., Bilotto, S. and Silvestre, F. (2011) Meiotic Regulation by Maturation Promoting Factor and Cytostatic Factor in Invertebrates. In Oocyte maturation and fertilization: A Long History for a Short event ed. Tosti, E. and Boni, R.. Bentham Science Publisher Ltd, Dubai, pp.80–92.
- Schlichter, L.C. (1983) A role for action potentials in maturing Rana pipiens oocytes. Dev Biol 98:60–69.
- Schorderet-Slatkine, S. (1972) Action of progesterone and related steroids on oocyte maturation in Xenopus laevis. An in vitro study. Cell Differ 1:179–189.
- Silvestre, F., Cuomo, A. and Tosti, E. (2009) Ion current activity and molecules modulating maturation and growth stages of ascidian (Ciona intestinalis) oocytes. Mol Repr Dev 76:1084–1093.
- Silvestre, F., Gallo, A., Cuomo, A., Covino, T. and Tosti, E. (2011a) Role of cyclic AMP in the maturation of Ciona intestinalis oocytes. Zygote 19(4):365–371.
- Silvestre, F., Boni, R., Fissore, R.A. and Tosti, E. (2011b) Ca2+ signaling during maturation of cumulus-oocyte complex in mammals. Mol Reprod Dev 78:744–756.
- Simoncini, L. and Moody, W.J. (1990) Changes in voltage-dependent currents and membrane area during maturation of starfish oocytes: species differences and similarities. Dev Biol 138(1):194–201.
- Steinhardt, R.A. and Alderton, J.M. (1988). Intracellular free calcium rise triggers nuclear envelope breakdown in the sea urchin embryo. Nature 332:364–366.
- Stephan, M. and Agnew, W.S. (1991) Voltage-sensitive Na+ channels: motifs, modes and modulation. Curr Opin Cell Biol 3:676–684.
- Stricker, S.A. (2000) Comparative biology of calcium signaling during fertilization and egg activation in animals. Dev Biol 211:157–176.
- Stricker, S.A. (2006) Structural reorganizations of the endoplasmic reticulum during egg maturation and fertilization. Semin Cell Dev Biol 17:303–313.
- Stricker, S.A. and Smythe, T.L. (2001) 5-HT causes an increase in cAMP that stimulates, rather than inhibits, oocyte maturation in marine nemertean worms. Development 128:1415–1427.
- Stricker, S.A., Swiderek, L. and Nguyen, T. (2010) Stimulators of AMP-activated kinase (AMPK) inhibit seawater- but not cAMP-induced oocyte maturation in a marine worm: Implications for interactions between cAMP and AMPK signaling. Mol Reprod Dev 77:497–510.
- Sun, L. and Machaca, K. (2004) Ca2+ cyt negatively regulates the initiation of oocyte maturation. J Cell Biol 165(1):63–75.
- Sun, Q.Y. and Schatten, H. (2006) Regulation of dynamic events by microfilaments during oocyte maturation and fertilization. Reproduction 131:193–205.
- Sutcliffe, M.J., Smeeton, A.H., Wo, Z.G. and Oswald, R.E. (1998) Molecular modelling of ligand-gated ion channels. In Methods in Enzymology, 293, Ion Channels, part B, ed., Conn, M. Academic Press, USA, pp.589–620.
- Taglietti, V., Tanzi, F., Romero, R. and Simoncini, L. (1984) Maturation involves suppression of voltage-gated currents in the frog oocyte. J Cell Physiol 121(3):576–588.
- Terlau, H. and Stuhmer, W. (1998) Structure and function of voltage-gated ion channels. Naturwissenschaften 85:437–444.
- Tombes, R.M., Simerly, C., Borisy, G.G., and Schatten, G. (1992) Meiosis, egg activation, and nuclear envelope breakdown are differentially reliant on Ca2+, whereas germinal vesicle breakdown is Ca2+ independent in the mouse oocyte. J Cell Biol 117:799–811.
- Tomkowiak, M., Guerrier, P. and Krantic, S. (1997) Meiosis reinitiation of mussel oocytes involves L-type voltage-gated calcium channel. J Cell Biochem 64:152–160.
- Tosti, E. (2006) Calcium ion currents mediating oocyte maturation events. Reprod Biol Endocrinol 4:26.
- Tosti, E. (2010) Dynamic roles of ion currents in early development. Mol Reprod Dev 77:856–867.
- Tosti, E., Gallo, A. and Silvestre, F. (2011) Ion currents involved in oocyte maturation, fertilization and early developmental stages of the ascidian Ciona intestinalis. Mol Reprod Dev 78:854–860.
- Tosti, E., Boni, R. and Cuomo, A. (2000) Ca2+ current activity decreases during meiotic progression in bovine oocytes. Am J Physiol Cell Physiol 279:C1795–1800.
- Tosti, E., Boni, R. and Cuomo, A. (2002) Fertilization and activation currents in bovine oocytes. Reproduction 124:835–846.
- Tosti, E. and Boni, R. (2004) Electrical events during gamete maturation and fertilisation in animals and human. Hum Reprod Update 10:53–65.
- Tsafriri, A. (1979) Mammalian oocyte maturation: model systems and their physiological relevance. Adv Exp Med Biol 112:269–281.
- Tsafriri, A. and Dekel, N. (2011) Intra- and Intercellular Molecular Mechanism in Regulation of Meiosis in Murid Rodents. In Oocyte maturation and fertilization: A Long History for a Short event ed. Tosti, E. and Boni, R. Bentham Science Publisher Ltd, Dubai, pp. 38–63.
- Verkman, A.S., van Hoek, A.N., Ma, T., Frigeri, A., Skach, W.R., Mitra, A., (1996) Water transport across mammalian cell membranes. Am J Physiol 270:12–30.
- Vig, M., Beck, A., Billingsley, J.M., Lis, A., Parvez, S., Peinelt, C., (2006) CRACM1 multimers form the ion-selective pore of the CRAC channel. Curr Biol 6:2073–2079.
- Voronina, E. and Wessel, G.M. (2003) The regulation of oocyte maturation. Curr Top Dev Biol 58:53–110.
- Wang, C., Lee, K., Gajdócsi, E., Papp, A.B. and Machaty, Z. (2012) Orai1 mediates store-operated Ca2+ entry during fertilization in mammalian oocytes. Dev Biol 365:414–423.
- Wasserman, W.J. and Masui, Y. (1975) Initiation of meiotic maturation in Xenopus laevis oocytes by the combination of divalent cations and ionophore A23187. J Exp Zool 193:369–375.
- Weinstein, S.P., Kostellow, A.B., Ziegler, D.H. and Morrill, G.A. (1982) Progesterone-induced down-regulation of electrogenic Na+, K+-ATPase during the first meiotic division in amphibian oocytes. J Membr Biol 69:41–48.
- Whitaker, M. (1995) Regulation of the cell division cycle by inositol trisphosphate and the calcium signaling pathway. Adv Second Messenger Phosphoprotein Res 30:299–310.
- Worley, P.F., Zeng, W., Huang, G.N., Yuan, J.P., Kim, J.Y., Lee, M.G. and Muallem, S. (2007) TRPC channels as STIM1-regulated store-operated channels. Cell Calcium 42:205–211.
- Yamaji, Y., Seki, S., Matsukawa, K., Koshimoto, C., Kasai, M. and Edashige, K. (2011) Developmental ability of vitrified mouse oocytes expressing water channels. J Reprod Dev 57:403–408.
- Yamashita, M., Mita, K., Yoshida, N. and Kondo, T. (2000) Molecular mechanisms of the initiation of oocyte maturation: general and species-specific aspects. Prog Cell Cycle Res 4:115–129.
- Yanagimachi, R. (1994) Mammalian fertilization. In The Physiology of Reproduction ed. Knobil, E. and Neil, J. Raven Press, NY, pp.189–317.
- Yi, J.H., Lefievre, L., Gagnon, C., Anctil, M. and Dube, F. (2002) Increase of cAMP upon release from prophase arrest in surf clam oocytes. J Cell Sci 115:311–320.
- Yoshimura, Y., Nakamura, Y., Oda, T., Ando, M., Ubukata, Y., Karube, M., (1992) Induction of meiotic maturation of follicle-enclosed oocytes of rabbits by a transient increase followed by an abrupt decrease in cyclic AMP concentration. J Reprod Fert 95:803–812.
- Yu, F., Sun, L. and Machaca, K. (2009) Orai1 internalization and STIM1 clustering inhibition modulate SOCE inactivation during meiosis. Proc Natl Acad Sci USA 106:17401–17406.
- Yu, F., Sun, L. and Machaca, K. (2010) Constitutive recycling of the store-operated Ca2+ channel Orai1 and its internalization during meiosis. J Cell Bioll 91:523–535.
- Zhang, S.L., Yeromin, A.V., Zhang, X.H., Yu, Y., Safrina, O., Penna, A., (2006) Genome-wide RNAi screen of Ca(2+) influx identifies genes that regulate Ca(2+) release-activated Ca(2+) channel activity. Proc Natl Acad Sci USA 103:9357–9362.