Abstract
Non-obstructive azoospermia (NOA) is considered to be a severe infertility factor due to impaired spermatogenesis with the consequent absence of spermatozoa in the ejaculate. However, the underlying etiology and mechanism(s) remain elusive. The aim of this study was to investigate the mutation and association of single nucleotide polymorphisms (SNPs) of the phosphoglycerate mutase 4 (PGAM4) gene in Chinese Han infertile men with NOA. The entire coding region of PGAM4 gene was sequenced from 214 participants including 103 infertile men with NOA and 111 controls with proven fertility. Screening was carried out using PCR and DNA sequencing to identify novel mutations and SNPs of the entire coding region of PGAM4. No mutation, including A138C or G539A, was detected in the coding region of PGAM4. One novel synonymous mutation (G111A, rs20100573) in control individuals was identified. There was no significant difference between NOA patients and controls in the G75C (rs138178131) frequencies (1.9% (2/103) and 4.5% (5/111), respectively, P = 0.292 and P = 0.374, adjusted by age). PGAM4 coding region mutations were not observed and the G75C polymorphism is not associated with NOA susceptibility among the Chinese Han population.
Introduction
Infertility affects approximately 15% of couples worldwide with equivalent male and female factor [Aston et al. Citation2010]. Absence of spermatozoa in the ejaculated semen (azoospermia) is one of the etiologies of male infertility. About 10–15% of infertile men present with azoospermia, and non-obstructive azoospermia (NOA) is the cause in about 60% of these men [Marmar Citation2011]. With the application of intracytoplasmic sperm injection (ICSI) and testicular sperm extraction techniques, sperm can be retrieved in NOA patients [Yang and Liu Citation2012]. Several genetic aberrations have been defined in NOA, including numerical and structural chromosomal abnormalities and Y-chromosomal microdeletions [Dohle et al. Citation2002]. However, the causes of NOA are not fully understood. Although SNPs affecting male infertility have been reported, SNPs in the glucide metabolic pathway remain unknown.
Phosphoglycerate mutase (PGAM) is an evolutionarily conserved enzyme of intermediary metabolism that catalyzes the reversible isomerization of 3- phosphoglycerate (3PG) and 2- phosphoglycerate (2PG), a key step in both glycolytic and gluconeogenic pathways. Two distinct forms of PGAM are cofactor–dependent PGAM (dPGAM) and cofactor–independent PGAM (iPGAM) [Foster et al. Citation2010]. As an important enzyme in energy metabolism, PGAM also participates in carbohydrates transportation, catalytic activity, growth, and development. Williams and Ford [2001] showed that the level of ATP via glcolysis was critical for maintaining mammalian sperm motility and male fertility. Human sperm also obtain a high proportion of ATP from glycolysis that is correlated with motility. Multiple capacitation-associated signaling pathways including glycolysis and tyrosine phosphorylation regulate functional changes in sperm metabolism and motility that occur during fertilization [Goodson et al. Citation2012].
PGAM4, an X-linked retrogene, is one of the PGAM family members that is expressed in the postmeiotic stages of spermatogenesis and located in the principal piece of the flagellum and acrosome in ejaculated spermatozoa. The majority of such retrogenes are expressed specifically in the testis and also play an evolved functional role in spermatogenesis [Marques et al. Citation2005]. PGAM4 G75C (rs138178131) causes amino acid substitution and impaired enzymatic activity, leading to male infertility [Okuda et al. Citation2012]. Other non synonymous coding variants, rs19995586, rs191844393, rs147487697, rs141834933, and rs182803196 have been observed (http://www.1000genomes.org/).
Recently, Okuda et al. [2012] reported a statistically significant correlation between the non synonymous SNP G75C and male infertility susceptibility in the Japanese population. The main aim of this study was to investigate the mutation and influence of the PGAM4 polymorphism of NOA in Chinese Han individuals.
Results
The 214 participants enrolled in our study were aged from 22 to 37 years old, with a mean age of 29.2 years. The clinical characteristics and biochemical parameters of 103 patients with NOA and 111 healthy controls are summarized in . No obvious differences were observed between case and control groups with regard to their alcohol status (P = 0.132). However, smoking status, environmental radiation, and high temperature exposure had a negative effect on male reproduction (P < 0.05). There was a significant difference (P < 0.001; P < 0.05; P < 0.001) between the frequency of the G75C polymorphism and the serum FSH, LH, PRL levels in the case group ().
Table 1. Clinical characteristics of 214 participants.
In the present study, we screened 5 known SNPs rs19995586, rs191844393, rs147487697, rs141834933, and rs182803196. However, we failed to detect any significant difference between case and control group at these sites. The frequencies of PGAM4 G75C (rs138178131) and the association with the risk of NOA are shown in . No insertions, deletions, missense and nonsense mutations were detected in either the case or control groups. One novel synonymous mutation (G111A, rs20100573) among the control subjects was identified. In addition five controls and two cases of G75C, which were previously reported as polymorphic sites, were identified. In addition five controls and two cases of G75C, which were previously reported as polymorphic sites, were identified. However, no significant difference P = 0.292 (P = 0.374, adjusted by age) was detected between NOA cases and controls (). In order to extend the Okuda study [Okuda et al. Citation2012] in the Chinese Han population, PCR amplified samples shown in were sequenced revealing G75C, A138C, and G539A transitions/trasversions and representative results and are presented in .
Fig. 1 Electophoretic analysis of the polymerase chain reaction (PCR) product of PGAM4 (Lanes 1-8, 1389 bp amplicon). Lane M, DL-2000 Marker. PCR was performed with the isolated DNA using the 5’-CCAATAGCCAGGTAAGTGAAC-3’ (forward) and 5’- CCATCTGCAGCTACAACTC-3’(reverse) primers. The products were analyzed by 1.5% agarose gel electrophoresis and the results were visualized under UV illumination. Lane M, 250 ng DNA marker (TaKaRa Biotechnology, Dalian, China). Lanes 1-8 were the expected PCR product which size was 1389 bp.
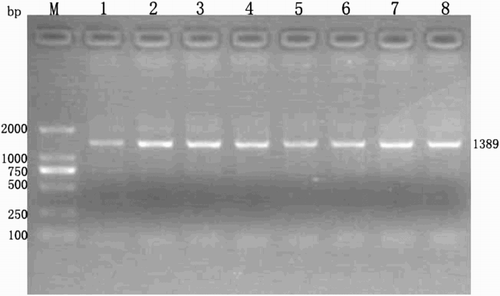
Fig. 2 Reverse sequencing. The PCR products were analyzed by reverse sequencing primed with GATGGGAATACCAGTCGG. A tryptophan (Trp) to cysteine (Cys) substitution was detected by comparing: A) 5’-…TGG TAC GAC GCC…-3’ to B) 5’-…TGC TAC GAC GCC…-3’. Arrows indicate the PGAM4 G75C (rs138178131) G→C transversion. C) The result of A→C transversion retained (Arg). The arrow indicates the site of PGAM4 A138C at which a synonymous mutation was identified in the Japanese population but not the Chinese Han population. D) The reverse sequencing of PGAM45’ G539A. The arrow indicates the site of the previously reported G→A transition within the Japanese population. This yields an arginine (Arg)→ histidine (His) substitution. However, the G→A was not detected among the 214 Chinese Han subjects.
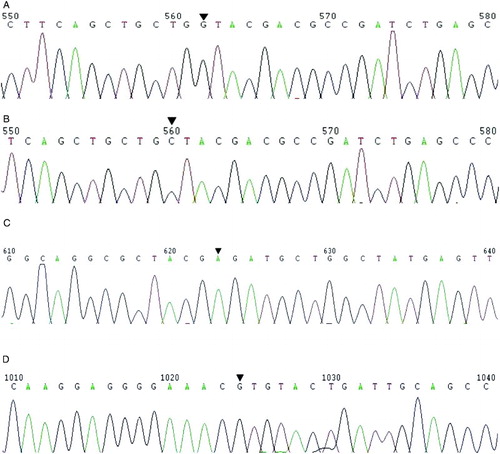
Table 2. The frequency distribution of the PGAM4 genotypes in patients and controls.
Discussion
Spermatogenesis is a dramatic complicated strictly regulated process that occurs in continuous mitotic, meiotic, and post-meiotic phases. Any defects during differentiation and maturation may cause infertility. However, the molecular pathways underlying the genetic defects in spermatogenesis are not well-established [Okada et al. Citation2008].
Non-obstructive azoospermia clinically presents as severely defective spermatogenesis [Kolettis Citation2002]. PGAM, a muscle-specific gene, is also expressed in meiotic and haploid male germ cells during testis maturation [Broceno et al. Citation1995]. PGAM-M, a subunit of PGAM, is expressed in cardiac muscle and testis. Broceno et al. [1999] demonstrated that it is transcriptionally activated during spermatogenesis. Recently, PGAM4's role in sperm motility has been confirmed [Okuda et al. Citation2012]. However, the exact function of PGAM4 in spermatogenesis remains to be determined. Therefore, in order to provide a theoretical basis for subsequent functional experiments, this study investigated the possible association between the G75C polymorphism at the PGAM4 gene and NOA in Chinese men.
Our preliminary study shows, for the first time, that PGAM4 G75C is not associated with NOA in the Chinese Han population. The results suggested that PGAM4 mutations and polymorphisms can be excluded as a genetic cause of NOA in humans or perhaps plays a small role in spermatogenesis. These results are in contrast to Okuda et al. [2012] that reported that PGAM4 G75C polymorphism was associated with male infertility.
These divergent observations may resolve as the following factors. First, the frequency distribution of G75C genotype in Chinese individuals was much lower than Japanese (1.9% in case and 4.5% in control, respectively, compared to 4.5% and 1.2% as Okuda reported [Okuda, et al. Citation2012] ), which may indicate an ethnic difference between the two populations. Second, we exclusively selected patients with NOA as the case group. However, men with severe oligozoospermia, asthenozoospermia, NOA, and idopathic male infertility were all previously included [Okuda et al. Citation2012]. Therefore, the different sample composition may lead to the diverse conclusion of these two studies. Third, as male infertility is a complex heterogeneous disorder, we should not underestimate the importance of genotype-environment interaction on male reproductive health [Aydemir et al. Citation2006]. Other genetic variants and local environmental factors may alter the phenotype in different geographical regions.
This small group of 103 patients represents only a subgroup of men suffering from NOA in the Chinese Han population. To ascertain the association and function of PGAM4 in spermatogenesis and its contribution to NOA, a variety of population screens and well-designed studies based on larger sample sizes are required.
Materials and Methods
Study sample
A total of 214 unrelated Chinese Han subjects were recruited from the Institute of Reproductive Medicine between September 2010 and May 2012. This included 103 patients with non-obstructive azoospermia and 111 fertile men. All subjects in the case group received a physical, andrological examination, semen analyses, serum hormone determination of follicle-stimulating hormone (FSH), luteinizing hormone (LH), prolactin(PRL), estradiol (E2), testosterone (T), urinary ultrasound, karyotyping, and Y chromosome microdeletion screening. Infertile men with NOA were defined as demonstrated by at least two separate semen analyses performed strictly adhering to WHO [1999] criteria (in 2010, ISBN:9789241547789). Exclusion criteria included obstructive azoospermia, cryptorchidism, varicocele, hypogonadotropic hypogonadism, genital trauma, reproductive tract infections, karyotype abnormalities, and Y chromosome microdeletions. The control individuals consisted of fertile men who have fathered at least one child and have normal semen quality as defined by WHO. All the participants in the study signed informed consent for genomic DNA analysis of their blood samples, and also completed a questionnaire which included information about medical history, occupation, reproductive history, alcohol drinking, cigarette smoking, and radiation exposure. The study was approved by the Ethics Committee of Wannan Medical College and conducted according to Declaration of Helsinki principles. The clinical characteristics of the 214 participants are displayed in .
PGAM4 mutation scanning
Three independent seminal fluid examinations were carried out after at least 3 d of sexual abstinence. In addition, 5 ml of EDTA anticoagulated venous blood was collected from each participant and stored at −80°C. Serum hormone levels were assessed by magnetic-separation ELISA. The results were considered within the reference range: FSH, 1.67–11.98 mIU ml−1; LH, 3.0–12.0 mIU ml−1; prolactin, 5.0–17.0 ng ml−1; E2, 41.42 pg ml−1; and T, 2.41–11.41 ng ml−1. Genomic DNA was extracted from peripheral blood lymphocytes by using QIAamp DNA Blood Mini kit (QIAGEN, Germany). The sequences of primers designed for amplifying the coding regions of PGAM4 were 5'-CCAATAGCCAGGTAAGTGAAC-3' (forward) and 5'- CCATCTGCAGCTACAACTC-3'(reverse). The PCR initiated with the 3 min denaturation at 95°C, then continued for 35 cycles (50 s at 95°C, 50 s at 55°C, 55s at 72°C), and ended with the 7min extension at 72°C. The PCR products were visualized by gel electrophoresis on 1.5% agarose gels (). The 5'- GATGGGAATACCAGTCGG -3' primer was used for reverse sequencing. The PCR products of PGAM4 were sequenced by the ABI 3730xl DNA analyzer (Applied Biosystems, Foster City, CA, USA) in Beijing Genomics Institute. The obtained sequence was analyzed using Seqman of DNASTAR package (Software for Molecular Biology, http://www.dnastar.com/) and compared with standard sequence by MegAlign for mutation scanning.
Statistical analysis
Statistical analysis was carried out using SPSS statistical software (version 10.0; SPSS, Inc., Chicago, IL, USA). The differences in genotype distributions of involved individuals between case and control groups were evaluated using chi-squared test or Fisher's exact test if one of the obtained frequencies was less than five. P < 0.05 was considered as statistical significance.
Abbreviations
PGAM4: | = | phosphoglycerate mutase 4 |
NOA: | = | non-obstructive azoospermia |
SNPs: | = | single nucleotide polymorphisms |
ICSI: | = | intracytoplasmic sperm injection |
3PG: | = | 3-phosphoglycerate |
2PG: | = | 2-phosphoglycerate |
dPGAM: | = | cofactor–dependent PGAM |
iPGAM: | = | cofactor – independent PGAM |
FSH: | = | follicle-stimulating hormone |
LH: | = | luteinizing hormone |
PRL: | = | prolactin |
E2: | = | estradiol |
T: | = | testosterone. |
Acknowledgments
We are grateful to the volunteer participants for their significant contributions.
Declaration of interests: This work was supported by National Natural Science Foundation of China (No. 30973197), National Basic Research Program of China (No. 2010CB945102), National Science & Technology Pillar Program of China (No. 2008BAH24B05) and National Infrastructure Program of Chinese Genetic Resources (No. 2006DKA21300). The authors have declared that no competing interests exist. We warrant that this article is the Authors' original work that has not received prior publication or under consideration for publication elsewhere.
Author contributions: Main investigator, participated in the design of the study, performed the experiment, and drafted the manuscript: QJ; Participated in the execution of the study: HP; Conceived and designed the experiments: BW; Analyzed the data and revised the manuscript: JW; Collected all the samples: TL, XY; Participated in the semen analysis: CJ, XF; Designed the experiments and are the corresponding authors of the article: YP, XM. All the authors have read and approved the final manuscript.
References
- Aston, K.I., Krausz, C., Laface, I., Ruiz-Castane, E. and Carrell, D.T. (2010) Evaluation of 172 candidate polymorphisms for association with oligozoospermia or azoospermia in a large cohort of men of European descent. Hum Reprod 25:1383–1397.
- Aydemir, B., Kiziler, A.R., Onaran, I., Alici, B., Ozkara, H. and Akyolcu, M.C. (2006) Impact of Cu and Fe concentrations on oxidative damage in male infertility. Biol Trace Elem Res 112:193–203.
- Broceno, C., Ruiz, P., Reina, M., Vilaro, S. and Pons, G. (1995) The muscle-specific phosphoglycerate mutase gene is specifically expressed in testis during spermatogenesis. Eur J Biochem 227:629–635.
- Broceno, C., Walsh, K. and Pons, G. (1999) A 1.3-kb upstream 5' region of the rat phosphoglycerate mutase m gene confers testis and skeletal muscle-specific expression in transgenic mice. Biochem Biophys Res Commun 263:244–250.
- Dohle, G.R., Halley, D.J., Van Hemel, J.O., van den Ouwel, A.M., Pieters, M.H., Weber, R.F., (2002) Genetic risk factors in infertile men with severe oligozoospermia and azoospermia. Hum Reprod 17:13–16.
- Foster, J.M., Davis, P.J., Raverdy, S., Sibley, M.H., Raleigh, E.A., Kumar, S., (2010) Evolution of bacterial phosphoglycerate mutases: non-homologous isofunctional enzymes undergoing gene losses, gains and lateral transfers. PLoS One 5:e13576.
- Goodson, S.G., Qiu, Y., Sutton, K.A., Xie, G., Jia, W. and O'Brien, D.A. (2012) Metabolic Substrates Exhibit Differential Effects on Functional Parameters of Mouse Sperm Capacitation. Biol Reprod. 87:1–15.
- Kolettis, P.N. (2002) The evaluation and management of the azoospermic patient. J Androl 23:293–305.
- Marmar, J.L. (2011) Techniques for microsurgical reconstruction of obstructive azoospermia. Indian J Urol 27:86–91.
- Marques, A.C., Dupanloup, I., Vinckenbosch, N., Reymond, A. and Kaessmann, H. (2005) Emergence of young human genes after a burst of retroposition in primates. PLoS Biol 3:e357.
- Okada, H., Tajima, A., Shichiri, K., Tanaka, A., Tanaka, K. and Inoue, I. (2008) Genome-wide expression of azoospermia testes demonstrates a specific profile and implicates ART3 in genetic susceptibility. PLoS Genet 4:e26.
- Okuda, H., Tsujimura, A., Irie, S., Yamamoto, K., Fukuhara, S., Matsuoka, Y., (2012) A single nucleotide polymorphism within the novel sex-linked testis-specific retrotransposed PGAM4 gene influences human male fertility. PLoS One 7:e35195.
- WHO (2010) WHO laboratory manual for the examination and processing of human semen. 5th Edition. WHO press, Geneva, Switzerland.
- Williams, A.C. and Ford, W.C. (2001) The role of glucose in supporting motility and capacitation in human spermatozoa. J Androl 22:680–695.
- Yang, J. and Liu, J.H. (2012) Microdissection testicular sperm extraction for non-obstructive azoospermia. Zhonghua Nan Ke Xue 18:551–555.