Abstract
A sub-acute toxicity test was performed to investigate the effects of molybdenum (Mo) on ovarian function. ICR adult female mice were exposed to Mo by free access to distilled water containing the Mo at 5, 10, 20, and 40 mg/L for 14 days. Compared to the control group, M II oocyte morphology, ovary index, and ovulation improved within the 5 mg/L Mo group, but were negatively affected by Mo at 40 mg/L. Morphologically abnormal ovarian mitochondria were observed at ≥ 20 mg/L. These alterations accompanied the changes in superoxide dismutase (SOD), glutathione peroxidise (GPx), and malondialdehyde (MDA) levels in ovaries. In conclusion, Mo affects oocyte quality possibly through regulating ovarian oxidative stress in a dose-dependent manner. It appears that Mo may improve ovarian function at a suitable concentration, which might be a candidate for the treatment of female infertility.
Introduction
There is growing interest in the possible health threat posed by endocrine-disrupting chemicals, which have effects on male and female reproduction [Diamanti-Kandarakis et al. Citation2009]. Molybdenum (Mo) is one of the endocrine disruptors widely present in food, water [Underwood Citation1981; Yu et al. Citation2011], and industrial products [Braydich-Stolle et al. Citation2005; Ema et al. Citation2010; Pandey and Singh Citation2002]. Molybdenum is also an environmental pollutant discharged from combustion and is present in contact lens solutions and as a color additive in cosmetics [ACGIH Citation1995]. Its wide distribution greatly increases the risk of animal and human environmental exposure to Mo.
It has been established that excessive ingestion of Mo can cause male reproductive toxicity in animals and humans [Bersenyi et al. Citation2008; Lyubimov et al. Citation2004; Meeker et al. Citation2008; Pandey and Singh Citation2002; Wirth and Mijal Citation2010]. However, fewer studies and conflicting information has been presented with respect to its effect on female reproductive health. Fungwe et al. [1990] indicated that a high level of Mo caused prolonged estrous cycle and abnormal embryogenesis in rats. Similarly, Huang et al. [2011] suggested that at a high-dose (8.51 µg/mg average content levels in soil) exposure during human pregnancy was related to the increased risk of neural tube defects. In contrast, other studies showed no effects on the estrous cycle, conception rates, and reproductive indices in rats or guinea pigs [Howell et al. Citation1993; Lyubimov et al. Citation2004]. To our knowledge, the mechanism of Mo affecting female reproductive health is far from clear.
Oxidative stress is a possible mechanism by which ovarian damage could accumulate presenting as oocyte deformity and eventually female infertility [Behrman et al. Citation2001; Gupta et al. Citation2006; Hussain et al. Citation2005; Lim and Luderer Citation2011; Tessaro et al. Citation2012; Wang et al. Citation2012]. It occurs when the formation of reactive oxygen species (ROS) exceeds the ability of the cells to defend themselves from increased ROS [Gupta et al. Citation2006]. A biochemical and molecular cascade (lipids, proteins, DNA, and/or RNA) of a whole organ can be affected with ensuing detriment [Pelicano et al. Citation2003]. Antioxidants, such as superoxide dismutase (SOD), glutathione peroxidase (GPx), and catalase (CAT), are important in protecting ovarian cells from oxidative stress [Matzuk et al. Citation1998; Tilly and Tilly Citation1995]. Malondialdehyde (MDA) reflects the level of peroxidation and the degree of cell injury [Sharma 2004]. Thus, ovarian oxidative stress can be evaluated by detecting SOD and GPx, as well as the level of MDA [Bozkurt et al. Citation2012].
Mitochondrial integrity and function also reflect the degree of oxidative stress [Hagen et al. Citation2004; Lenaz Citation2001; Pelicano et al. Citation2003; Schuh et al. Citation2005]. This reflects the ROS by-product during the metabolic processes involving the mitochondrial electron transfer chain [Hagen et al. Citation2004; Lenaz Citation2001; Pelicano et al. Citation2003]. Reduced mitochondrial membrane potential, defective activity, and impaired functions damaged ovaries, germinal vesicle (GV), or metaphase II (M II) oocytes [Gupta et al. Citation2006; Hussain et al. Citation2005; Ou et al. Citation2012; Tessaro et al. Citation2012].
Thus, this study was designed to investigate the possible toxicity of Mo on the mouse ovary and to explore the possible mechanism affecting female reproductive health. At high doses alterations in M II oocyte morphology, ovarian index, ovarian oxidative stress, and ultrastructure of mitochondria in ovarian cells were detected after mice were exposed to Mo for 14 days. This may be associated with ovarian oxidative stress.
Results and Discussion
Effects of Mo exposure on ovary parameters and M II oocyte morphology
Distinct hyperemia was observed in the ovaries exposed to ≥ 20 mg/L Mo (Fig.1). This suggested that ovarian varicocele can follow Mo exposure. However, at 5 mg/L, Mo significantly improved the ovulation number () and M II oocyte morphology, but did not affect the ovary index. In contrast, at 40 mg/L the ovary index significantly decreased and reduced M II oocyte morphology (P < 0.01) () was observed but it did not significantly change the ovulation number.
Figure 1. The ovaries exposed to molybdenum (Mo) at 20 mg/L (B) and 40 mg/L (C) exhibited the obvious hyperemia (black arrows), compared to the control group (A) (white arrows).
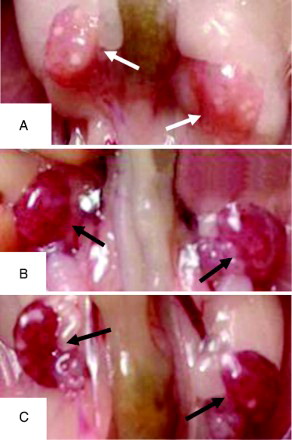
Figure 2. Morphological images of M II oocytes (×400). Compared to the control (A), the morphological abnormalities of M II oocytes were observed in mice exposed to molybdenum at ≥20 mg/L, such as no first polar body (B), distorted cytoplasm (C), transformative zona pellucida and excessive vacuolization (D), shrunken cytoplasm (E), and centrally severe granulation (F).
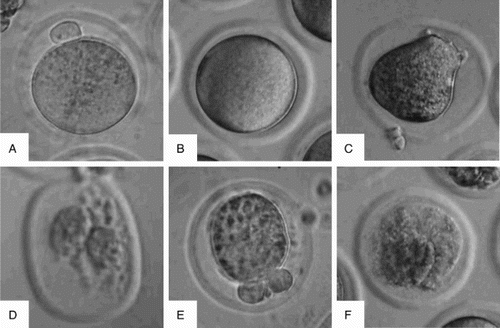
Table 1. Effects of molybdenum exposure on ovary parameters and M II oocyte morphology.
Effects of Mo on the levels of SOD, GPx, and MDA of ovaries in mice
As shown in , compared to control, Mo at 5 mg/L stimulated SOD and GPx activities, but did not obviously change the content of MDA. At 40 mg/L Mo significantly decreased the activity of SOD and GPx as the level of MDA significantly increased. In comparison, the effect of Mo was tempered on these oxidative stress parameters at 10 mg/L or 20 mg/.
Table 2. Effects of molybdenum exposure on the levels of SOD, GPx, and MDA in ovaries.
Ultra-structural changes of ovary tissue after mice exposed to Mo
Transmission electron microscopy (TEM) images () showed that the ovaries were damaged to some extent by exposure to ≥ 20 mg/L Mo. As a whole, the cytoplasm of ovarian cells contained relatively few organelles, and smaller, morphologically abnormal, swollen mitochondria with distorted cristae in the 20 mg/L and 40 mg/L group compared to the control group (C and F). The vacuolization, mild swelling, disappearance of cristae, and incomplete membrane were observed in damaged intra-ovarian oocytes (A and B) and granulosa cells (D and E).
Figure 3. Transmission electron microscopic images of the intra-ovarian oocytes (A-C) and the granulosa cells (D-F) in ovaries. Compared to the control (C, F, ×40,000), the mitochondrial vacuolization (A; ×5,000) and the swollen mitochondria with distorted cristae (B; ×40,000) in ovarian oocytes, and the mitochondrial vacuolization in granulosa cells (D, E; ×10,000) were observed in the 20 mg/L (A, D) and 40 mg/L (B, E) group. The arrow indicated the zona pellucida in A, and the arrows or cycle indicated the mitochondria in B-F.
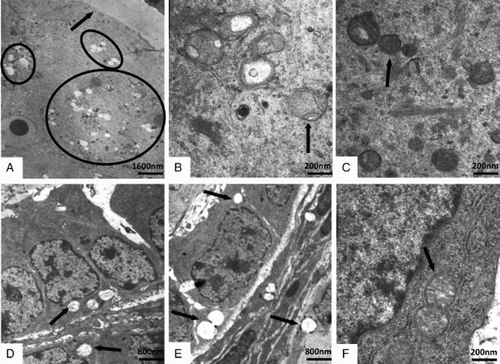
The results presented in this study suggest that Mo dually influences ovarian function in a dose-dependent manner. M II oocyte quality was significantly improved when oxidative stress was decreased with 5 mg/L Mo. On the contrary, oxidative damage increased at the 40 mg/L and M IIoocyte quality was significantly decreased.
To our knowledge, this is the first report concerning the improvement of in vivo oocyte quality by oral administration of Mo at a low dose (but not at the physiological dose). Molybdenum at 5 mg/L significantly increased ovulation as well as decreased the rate of abnormal oocytes () and ovarian oxidative stress (). This indicated that at low dose, Mo could improve ovarian function. A similar improvement in reproductive capacity was observed in our previous study. At 5 mg/L Mo improved the development of mouse embryos cultured in vitro [Bi et al. Citation2013], and at 12.5 mg/L enhanced the mouse sperm quality and testicular function in vivo [Zhai et al. Citation2013]. Additionally, in vitro plasma membrane leakage was promoted by 5 mg/L and 10 mg/L Mo [Braydich-Stolle et al. Citation2005]. These beneficial effects of Mo on the reproductive system were probably due to the biological functions of Mo at low concentrations. The results in this study are in line with the above studies.
Molybdenum at high levels significantly increased ovarian oxidative damage (), which might be due to the imbalance of trace metals. Guo et al. [2012] showed that the imbalance of trace metals is associated with oxidative stress. At ≥ 40 mg/L Mo significantly decreased the level of ovarian Cu, Zn, and Mn (unpublished results). This would likely have decreased the activities of Cu-Zn SOD and Mn SOD because that Cu and Zn and Mn are prosthetic groups of Cu-Zn SOD and Mn SOD, respectively [Sevilla et al. Citation1982]. A decrease in Mn SOD activity has been shown to promote lipid, protein, and DNA damage [Adachi et al. Citation1991].
The ovary index (weight) significantly decreased when the dose of Mo was increased to the 40 mg/L. This likely reflects ovarian apoptotic cell death that is regulated by sex steroids [Billig et al. Citation1993]. Molybdate interacts directly with steroid (androgens and estrogens) receptors, stabilizes receptors for androgens and estrogens, preventing receptor inactivation or transformation [Dahmer et al. Citation1984; Van Niekerk and Van Niekerk Citation1989]. The high dietary intake of Mo could disturb the metabolic fate of the major estrogens [Beattie and Weersink Citation1992; Murayama and Fukai Citation1985], which can affect ovary weight [Hegele-Hartung et al. Citation2004]. The association between ovarian weight and estrogen was also reported in adult female rats exposed to neonatal prazosin [Fitch and Feder Citation1992]. The results in the present study are consistent with a report by du Plessis et al. [1999] where a Mo (38 mg/kg feed) and sulphur (0.34%) supplemented diet of adult South African Mutton Merino ewes yielded reduced ovarian weight.
The M II oocyte morphology was affected by Mo at 40 mg/L in a significantly negative way (). A similar phenomenon has been observed in other types of cells. Our previous study showed that at ≥ 40 mg/L, Mo significantly decreased morphology and development of in vitro cultured embryos [Bi et al. Citation2013]. Ammonium tetrathiomolybdate at 10 mg/L resulted in a marked reduction in cellular clumping, while at 100 mg/L and higher resulted in an absence of clumping [Kendall et al. Citation2003]. In addition, ammonium molybdate at 330 mg/L inhibited cell division and differentiation in vitro [Kolesarova 2009]. The morphological changes are similar to previous observations.
Ovarian hyperemia was observed in the group of animals exposed to ≥ 20 mg/L. The exact mechanism for this is unclear and requires attention. However, our previous study and others have shown that Mo could provoke a varicocele in male mice [Zhai et al. Citation2013] or men [Makker et al. Citation2009]. Studies have suggested that the long-lasting ovarian varicocele can cause hypofunction of the ovaries, similar to testicular varicocele, and could be a cause of infertility [Galkin et al. Citation1991; Tarazov et al. Citation2011]. Ovarian hyperemia was accompanied with more morphologically abnormal M II oocytes, lighter ovaries, damaged oocytes, and granulosa cells in ovaries. These results support the above hypothesis and were consistent with our previous study on the testis toxicity of Mo [Zhai et al. Citation2013].
The decreased ovary index observed and the increased abnormal M II oocyte morphology might be mediated by the increased oxidative damage, which might cause the ovarian dysfunctions. Studies have shown that oxidative damage is a common mediator of apoptosis [Buttke and Sandstrom Citation1994] in ovarian endothelial cells and luteal cells [Faucher et al. Citation2005; Nakamura et al. Citation2001]. Further, it can arrest the ovarian cell-cycle [Clopton and Saltman Citation1995] and inhibit antral follicle growth [Wang et al. Citation2012]. In this study, the mitochondria of the intra-ovarian oocytes and granulosa cells were injured to some extent and exhibited the ultra-structural pathological changes in ≥ 20 mg/L groups (), consistent with increased oxidative damage.
In conclusion, the present study suggests that Mo has both the nutritional and toxic function on the ovarian function and M II oocyte quality in a dose-dependent manner. At a low dose, Mo improved overall quality but at a high dose it damaged the ovarian function and M II oocyte quality. The damage likely reflects the action of ovarian oxidative stress. To our knowledge, this is the first report concerning the improvement of oocyte quality in vivo by dose dependent oral administration of Mo.
Methods and Materials
Chemicals
Unless otherwise stated, all components used in this study were purchased from Sigma–Aldrich Corp. (St. Louis, MO, USA).
Animals
The study design was approved by the Animal Care and Use Committee at the Henan University of Science and Technology. Adult ICR strain female mice, aged 4 to 8 w, were used for toxicity experiments. All mice used in this study were maintained under Good Laboratory Practice (GLP) conditions. The mice had free access to a commercial standard pellet diet and distilled water ad libitum.
Exposure of mice to Mo
One hundred and twenty five healthy ICR strain mice, of proven fertility, were divided randomly into five groups of 25 animals each. The mice in different groups were respectively allowed free access to the distilled water containing the Mo (sodium molybdate dihydrate) at concentrations of 0, 5, 10, 20, or 40 mg/L for 14 d.
Transmission electron microscopy of ovaries
The ovaries were quickly removed and cut into blocks. Blocks were retrimmed into 1 mm3 and further prefixed with 2.5% glutaraldehyde in a 0.2 M phosphate buffer (pH 7.4) at 4°C for 3 h. The tissues were rinsed three times in the same buffer, postfixed with 1% OsO4 at 4°C for 2 h, and dehydrated in ethanol. The specimens for transmission electron microscopy were embedded in Epon. Ultra-thin sections cut horizontally were prepared and double stained with uranyl acetate and lead citrate. The ovarian ultrastructure was observed under a transmission electron microscope (JEM-2000EX; JEOL, Tokyo, Japan), and images were recorded. The ultrastructure position of the oocytes was identified according to the position of the zona pellucida, as shown by the arrow in A, the oocyte ultrastructure was situated on the inside of the zona pellucida.
Collection of ovaries and oocytes
The mice in the Mo-exposed groups and control were superovulated by intraperitoneal injection of 10 IU PMSG followed 48 h later by 10 IU of hCG. At 20 h post-hCG, followed by body weighing, the ovaries were quickly removed, photographed, and weighed, then stored at −20°C for measurement of biochemical indicators. At the same time, cumulus–oocyte complexes (COCs) were collected from oviducts, and the cumulus cells were dispersed in M2 medium containing 0.1% hyaluronidase, then the oocytes were washed and collected in M2 medium, and counted under a stereomicroscope.
Evaluation of oocyte quality
Oocytes were morphologically classified as normal and abnormal according to the Alpha Scientists in Reproductive Medicine and ESHRE Special Interest Group of Embryology [2011] report under an inverted phase contrast microscope at ×400 magnification (TE-2000, Nikon, Tokyo, Japan). Briefly, the normal oocyte morphology ( A) was that of a spherical structure enclosed by a uniform zona pellucida, with a uniform translucent cytoplasm free of inclusions, a size-appropriate polar body, and a size-appropriate perivitelline space. The oocytes in other morphologies (B-F) were considered abnormal. The percentage of abnormal oocytes was calculated relative to the total number of ovulations.
Measurement of biochemical indicators
Ovaries were added into precooled 0.9% saline with a ratio of 1:9 (g:mL) and made into 10% homogenate using a Ultraschallprozessor (UP200S, Dr. Hielscher Gmbh, Germany), for the measurement of biochemical indicators. The activities of SOD and GPx, and the level of MDA in ovaries were determined by using the corresponding assay kits (Nanjing Jiancheng Bioengineering Institute, Nanjing, China) according to the manufacturer's instructions.
Statistical analysis
Except for the abnormality rate of oocytes, all of the data were expressed as the mean ± standard error of the mean and statistically analyzed by single variable of the general linear model using SPSS software (SPSS 17. 0). The number of ovulations and the abnormality rate of oocytes were analyzed by the Chi-Square Test. Differences between experimental groups were considered significant at P < 0.05 or extreme at P < 0.01.
Abbreviations
Mo: | = | molybdenum |
ROS: | = | reactive oxygen species |
SOD: | = | superoxide dismutase |
GPx: | = | glutathione peroxidise |
MDA: | = | malondialdehyde |
CAT: | = | catalase |
GV: | = | germinal vesicle |
M II: | = | metaphase II |
TEM: | = | transmission electron microscopy |
Acknowledgments
Chunjie Zhang Ph.D, Yinju Li Ph.D, Yumei Liu Ph.D, and Lifang Han laboratory assistant are thanked for assistance in the usage of instruments, Long Ta Ph.D for analysis of pathology slices, and Ms. Kerry Alicia Webber for the improvement of language for the writing of this paper.
Declaration of interest: This work was supported by the National Natural Science Foundation of China (Project No. 30901026) and the Higher Education Finance Scheme for Young Backbone Teachers in Henan Province (Project No. 2010GGJS- 071), China. The authors report no declarations of interest.
Author contributions: Conceived and designed the experiments: F-JL, Y-LZ, X-LY, X-LC; Performed the experiments: X-LC, X-WZ, L-JJ, QQ; Analyzed the data: F-JL, Z-JL, X-GM; Contributed reagents/ materials/ analysis tools: R-ZS; Wrote the manuscript: F-JL, X-LC, Y-LZ, R-ZS.
References
- ACGIH (1995) (American Conference of Governmental Industrial Hygienists). 1995-1996 Threshold Limit Values (TLVs) for chemical substances and physical agents and biological exposure indices (BEIs). ACGIH, Cincinnati, OH.
- Adachi, T., Ohta, H., Hirano, K., Hayashi, K. and Marklund, S.L. (1991) Non-enzymic glycation of human extracellular superoxide dismutase. Biochem J 279:263–267.
- Kolesarova, A., Capcarova, M., Sirotkin, A. and Massanyi, P. (2009) Insulin-like growth factor-I and progesterone release by ovarian granulosa cells of hens after experimental lead and molybdenum administrations in vitro. Inter J Poult Sci 8:890–895.
- Alpha Scientists in Reproductive Medicine and ESHRE Special Interest Group of Embryology (2011) The Istanbul consensus workshop on embryo assessment: proceedings of an expert meeting. Hum Reprod 26:1270–1283.
- Beattie, J.H. and Weersink, E. (1992) Borate and molybdate inhibition of catechol estrogen and pyrocatechol methylation by catechol-O-methyltransferase. J Inorg Biochem 46:153–160.
- Behrman, H.R., Kodaman, P.H., Preston, S.L. and Gao, S. (2001) Oxidative stress and the ovary. J Soc Gynecol Investig 8:S40–42.
- Bersenyi, A., Berta, E., Kadar, I., Glavits, R., Szilagyi, M. and Fekete, S.G. (2008) Effects of high dietary molybdenum in rabbits. Acta Vet Hung 56:41–55.
- Bi, C.M., Zhang, Y.L., Liu, F.J., Zhou, T.Z., Yang, Z.J., Gao, S.Y., (2013) The effect of molybdenum on the in vitro development of mouse preimplantation embryos. Syst Biol Reprod Med 59:69–73.
- Billig, H., Furuta, I. and Hsueh, A.J. (1993) Estrogens inhibit and androgens enhance ovarian granulosa cell apoptosis. Endocrinology 133:2204–2212.
- Bozkurt, S., Arikan, D.C., Kurutas, E.B., Sayar, H., Okumus, M., Coskun, A., (2012) Selenium has a protective effect on ischemia/reperfusion injury in a rat ovary model: biochemical and histopathologic evaluation. J Pediatr Surg 47:1735–1741.
- Braydich-Stolle, L., Hussain, S., Schlager, J.J. and Hofmann, M.C. (2005) In vitro cytotoxicity of nanoparticles in mammalian germline stem cells. Toxicol Sci 88:412–419.
- Buttke, T.M. and Sandstrom, P.A. (1994) Oxidative Stress as a Mediator of Apoptosis. Immunol Today 15:7–10.
- Clopton, D.A. and Saltman, P. (1995) Low-Level Oxidative Stress Causes Cell-Cycle Specific Arrest in Cultured-Cells. Biochem Biophys Res Commun 210:189–196.
- Dahmer, M.K., Housley, P.R. and Pratt, W.B. (1984) Effects of molybdate and endogenous inhibitors on steroid-receptor inactivation, transformation, and translocation. Annu Rev Physiol 46:67–81.
- Diamanti-Kandarakis, E., Bourguignon, J.P., Giudice, L.C., Hauser, R., Prins, G.S., Soto, A.M., (2009) Endocrine-disrupting chemicals: an Endocrine Society scientific statement. Endocr Rev 30:293–342.
- du Plessis, S.S., Van Niekerk, F.E. and Coetzer, W.A. (1999) The effect of dietary molybdenum and sulphate on the oestrus cycle and ovulation in ewes after manipulation with exogenous progesterone alone or in combination with FSH and LH. Small Ruminant Res 33:63–69.
- Ema, M., Kobayashi, N., Naya, M., Hanai, S. and Nakanishi, J. (2010) Reproductive and developmental toxicity studies of manufactured nanomaterials. Reprod Toxicol 30:343–352.
- Faucher, K., Rabinovitch-Chable, H., Cook-Moreau, J., Barriere, G., Sturtz, F. and Rigaud, M. (2005) Overexpression of human GPX1 modifies Bax to Bcl-2 apoptotic ratio in human endothelial cells. Mol Cell Biochem 277:81–87.
- Fitch, R.H. and Feder, H. (1992) Neonatal prazosin exposure reduces ovarian weight and estrogen receptor binding in adult female rats. Int J Dev Neurosci 10:435–438.
- Fungwe, T.V., Buddingh, F., Demick, D.S., Lox, C.D., Yang, M.T. and Yang, S.P. (1990) The Role of Dietary Molybdenum on Estrous Activity, Fertility, Reproduction and Molybdenum and Copper Enzyme-Activities of Female Rats. Nutr Res 10:515–524.
- Galkin, E.V., Grakova, L.S. and Naumova, E.B. (1991) Roentgeno-endovascular surgery of hypofunctional ovaries in varicosities of the ovarian veins. Vestn Rentgenol Radiol 5:51–59.
- Guo, C.H., Chen, P.C., Lin, K.P., Shih, M.Y. and Ko, W.S. (2012) Trace metal imbalance associated with oxidative stress and inflammatory status in anti-hepatitis C virus antibody positive subjects. Environ Toxicol Pharmacol 33:288–296.
- Gupta, R.K., Schuh, R.A., Fiskum, G. and Flaws, J.A. (2006) Methoxychlor causes mitochondrial dysfunction and oxidative damage in the mouse ovary. Toxicol Appl Pharmacol 216:436–445.
- Hagen, T., D'Amico, G., Quintero, M., Palacios-Callender, M., Hollis, V., Lam, F., (2004) Inhibition of mitochondrial respiration by the anticancer agent 2-methoxyestradiol. Biochem Biophys Res Commun 322:923–929.
- Hegele-Hartung, C., Siebel, P., Peters, O., Kosemund, D., Muller, G., Hillisch, A., (2004) Impact of isotype-selective estrogen receptor agonists on ovarian function. Proc Natl Acad Sci USA 101:5129–5134.
- Howell, J.M., Shunxiang, Y. and Gawthorne, J.M. (1993) Effect of thiomolybdate and ammonium molybdate in pregnant guinea pigs and their offspring. Res Vet Sci 55:224–230.
- Huang, J., Wu, J.L., Li, T.J., Song, X.M., Zhang, B.Z., Zhang, P.W., (2011) Effect of exposure to trace elements in the soil on the prevalence of neural tube defects in a high-risk area of China. Biomed Environ Sci 24:94–101.
- Hussain, S.M., Hess, K.L., Gearhart, J.M., Geiss, K.T. and Schlager, J.J. (2005) In vitro toxicity of nanoparticles in BRL 3A rat liver cells. Toxicol In Vitro 19:975–983.
- Kendall, N.R., Marsters, P., Scaramuzzi, R.J. and Campbell, B.K. (2003) Expression of lysyl oxidase and effect of copper chloride and ammonium tetrathiomolybdate on bovine ovarian follicle granulosa cells cultured in serum-free media. Reproduction 125:657–665.
- Lenaz, G. (2001) The mitochondrial production of reactive oxygen species: Mechanisms and implications in human pathology. IUBMB Life 52:159–164.
- Lim, J. and Luderer, U. (2011) Oxidative damage increases and antioxidant gene expression decreases with aging in the mouse ovary. Biol Reprod 84:775–782.
- Lyubimov, A.V., Smith, J.A., Rousselle, S.D., Mercieca, M.D., Tomaszewski, J.E., Smith, A.C., (2004) The effects of tetrathiomolybdate (TTM, NSC-714598) and copper supplementation on fertility and early embryonic development in rats. Reprod Toxicol 19:223–233.
- Makker, K., Agarwal, A. and Sharma, R. (2009) Oxidative stress & male infertility. Indian J Med Res 129:357–367.
- Matzuk, M.M., Dionne, L., Guo, Q., Kumar, T.R. and Lebovitz, R.M. (1998) Ovarian function in superoxide dismutase 1 and 2 knockout mice. Endocrinology 139:4008–4011.
- Meeker, J.D., Rossano, M.G., Protas, B., Diamond, M.P., Puscheck, E., Daly, D., (2008) Cadmium, Lead, and Other Metals in Relation to Semen Quality: Human Evidence for Molybdenum as a Male Reproductive Toxicant. Environ Health Perspect 116:1473–1479.
- Murayama, A. and Fukai, F. (1985) Effect of molybdate on the molecular organization of the estrogen receptor system of porcine uterus. J Biochem 98:1083–1090.
- Nakamura, T., Ishigami, T., Makino, N. and Sakamoto, K. (2001) The down-regulation of glutathione peroxidase causes bovine luteal cell apoptosis during structural luteolysis. J Biochem 129:937–942.
- Ou, X.H., Li, S., Wang, Z.B., Li, M., Quan, S., Xing, F., (2012) Maternal insulin resistance causes oxidative stress and mitochondrial dysfunction in mouse oocytes. Hum Reprod 27: 2130–2145.
- Pandey, R. and Singh, S.P. (2002) Effects of molybdenum on fertility of male rats. Biometals 15:65–72.
- Pelicano, H., Feng, L., Zhou, Y., Carew, J.S., Hileman, E.O., Plunkett, W., (2003) Inhibition of mitochondrial respiration: a novel strategy to enhance drug-induced apoptosis in human leukemia cells by a reactive oxygen species-mediated mechanism. J Biol Chem 278: 37832–37839.
- Schuh, R.A., Kristian, T., Gupta, R.K., Flaws, J.A. and Fiskum, G. (2005) Methoxychlor inhibits brain mitochondrial respiration and increases hydrogen peroxide production and CREB phosphorylation. Toxicol Sci 88:495–504.
- Sevilla, F., Lopez-Gorge, J. and Del Rio, L.A. (1982) Characterization of a manganese superoxide dismutase from the higher plant pisum sativum. Plant Physiol 70:1321–1326.
- Sharma, S., Kaur, R. and Sandhu, H.S. (2004) Effect of subacute oral toxicity of molybdenum on antioxidant status in cross bred cow calves. Ind J Anim Sci 74:734–736.
- Tarazov, P., Prozorovskij, K. and Rumiantseva, S. (2011) Pregnancy after embolization of an ovarian varicocele associated with infertility: report of two cases. Diagn Interv Radiol 17:174–176.
- Tessaro, I., Franciosi, F., Lodde, V., Corbani, D., Luciano, A.M. and Modina, S.C. (2012) 253 oxidative stress may impair oocyte quality in dairy cows of reproductive age with a reduced antral follicle count. Reprod Fertil Dev 25:274.
- Tilly, J.L. and Tilly, K.I. (1995) Inhibitors of oxidative stress mimic the ability of follicle-stimulating hormone to suppress apoptosis in cultured rat ovarian follicles. Endocrinology 136:242–252.
- Underwood, E.J. (1981) Trace metals in human and animal health. J Hum Nutr 35:37–48.
- Van Niekerk, F.E. and Van Niekerk, C.H. (1989) The influence of experimentally induced copper deficiency on the fertility of rams. I. Semen parameters and peripheral plasma androgen concentration. J S Afr Vet Assoc 60:28–31.
- Wang, W., Craig, Z.R., Basavarajappa, M.S., Hafner, K.S. and Flaws, J.A. (2012) Mono-(2- ethylhexyl) phthalate induces oxidative stress and inhibits growth of mouse ovarian antral follicles. Biol Reprod 87:152.
- Wirth, J.J. and Mijal, R.S. (2010) Adverse effects of low level heavy metal exposure on male reproductive function. Syst Biol Reprod Med 56:147–167.
- Yu, C., Xu, S., Gang, M., Chen, G. and Zhou, L. (2011) Molybdenum pollution and speciation in Nver River sediments impacted with Mo mining activities in western Liaoning, northeast China. Int J Environ Res 5:205–212.
- Zhai, X.W., Zhang, Y.L., Qi, Q., Bai, Y., Chen, X.L., Jin, L.J., (2013) Effects of Mo on sperm quality and testis oxidative stress. Syst Biol Reprod Med Early Online:1–5 [Epub ahead of print].