Abstract
The aim of this study was to determine if there are any associations between impaired chromatin packaging and the origin of reactive oxygen species (ROS) production. Cytosolic ROS, mitochondrial ROS, DNA protamination, and apoptosis were evaluated with dichlorofluoresceindiacetate (DCFH-DA), dihydrorhodamine 123 (DHR123), chromomycin A3 (CMA3), and YO-Pro-1 (Y1)/propidium iodide (PI), respectively, by flow cytometry (FCM) in 40 infertile individuals. Percentages DCF+ and R123+ sperm were positively associated with percentage CMA3+ sperm and negatively associated with percentage apoptotic sperm. No correlation was observed between CMA3+ sperm and the percentage of apoptotic sperm. Under protamination of sperm is not associated with the origin of ROS production, but their relationship may suggest an association with general physiological dysfunction of sperm. Furthermore, under protamination does make sperm prone to apoptosis. Rather, it is likely that apoptosis is induced by ROS production. Considering that these conclusions are derived from correlative analyses, additional studies including an interventive approach are required.
Introduction
It is believed that understanding the mechanism of reactive oxygen species (ROS) induced DNA damage could potentially hold future importance in assisted reproductive management. Studies have shown that sperm DNA damage is highly associated with increased miscarriage, reduced fertilization, and implantation and, thereby, a decrease in the rate of fertility [Borini et al. 2006; Carrell et al. 2003; Henkel et al. 2004; Kodama et al. 1997; Lewis and Aitken 2005].
Considering the importance of DNA integrity in the transfer of the male genome to the oocyte, sperm has acquired different orders of chromatin packaging that protects it from ROS and DNA damage [Aoki and Carrell 2003]. As a result, during spermatogenesis the somatic histones are gradually replaced by protamines, to form a stable nuclear structure of mature spermatozoa [Oliva 2006; Poccia 1986]. Concomitant with this event, a substantial portion of cytoplasm is phagocytized by Sertoli cells and thereby a major source of antioxidant capacity is lost which may render the sperm more prone to oxidative stress when faced with oxidants [de Lamirande and Gagnon 1995]. To overcome this deficiency, the sperm is mainly protected by the antioxidants present in seminal fluid [Agarwal et al. 2003]. Therefore, in the absence of seminal fluid, sperm are prone to DNA damage.
Sperm DNA damage can arise by different mechanisms, among which it is believed that the excess production of ROS plays a major role [Aitken et al. 1998; Henkel et al. 2005; Oger et al. 2003; Saleh et al. 2002a; Wang et al. 2003]. Reactive oxygen species can originate from endogenous sources such as leukocytes and immature spermatozoa [Aitken and West 1990; Kessopoulou et al. 1992; Shekarriz et al. 1995], as well as, exogenous sources such as industrial compounds, smoking, and alcohol [Acharya et al. 2003; Agarwal and Saleh 2002; Kasahara et al. 2002; Koch et al. 2004; Kothari et al. 2010; Saleh et al. 2002b]. In infertile men, the mitochondrion is a major source of endogenous ROS production, which can result in DNA damage and apoptosis in spermatozoa [Barroso et al. 2000; Koppers et al. 2008]. Additionally, ROS may be generated in the cytosol of spermatozoa [Aitken et al. 1997].
Under protamination, which can be assessed indirectly by chromomycin A3 (CMA3) staining, has been considered to be a sign of sperm immaturity [Bianchi et al. 1993; Manicardi et al. 1995]. Although controversial, it is believed that there is an association between poor chromatin remodeling and ROS production [De Iuliis et al. 2009; Henkel et al. 2010; Tunc et al. 2009], however, the origin of the ROS is not clear.
We previously standardized the dihydrorhodamine 123 (DHR123) staining assay for the detection of ROS, which is mainly attributed to mitochondrial ROS [Kiani-Esfahani et al. 2012]. In addition, dichlorofluoresceindiacetate (DCFH-DA) staining has also been used to detect cytosolic ROS. The objective of this study was to investigate the origin of ROS in sperm that have poor chromatin remodeling. We aimed to evaluate the relationship between poor chromatin remodeling and the origin of ROS with apoptosis.
Results
shows the descriptive analysis of results for semen parameters, ROS, and apoptosis in 40 infertile males. represents ROS levels in flow cytometric dot plots in populations of the live and dead sperm as assessed by DCFH-DA, DHR123, and PI staining. The lower right (LR) quadrant shows live/ ROS+. The upper right (UR) quadrant shows dead/ ROS + , while the lower left (LL) and the upper left (UL) quadrant contains live/ROS- and dead/ROS- sperm, respectively (C for DCF and 1D for DHR123). Unlike percentage of dead/R123+ sperm, the percentage of dead/ DCF+ sperm is very low (see Discussion).
Figure 1. Dihydrorhodamine 123 (DHR123) and dichlorofluoresceindiacetate (DCFH-DA) staining in human spermatozoa. Flow cytometric dot plot of semen sample stained with DCFH-DA/ propidium iodide (PI) (A and C) and DHR123/ PI (B and D). Panels A and B represent PI control without DCFH-DA and DHR123 whereas panels C and D represent reactive oxygen species (ROS) production in the live or dead sperm population that were stained with DCFH-DA and DHR123, respectively. Fluorescence microscopy image of human spermatozoa shows DCFH-DA staining for cytosolic ROS detection (E), PI was used as a counter stain to assess membrane integrity (F), and DHR123 staining for mitochondrial ROS in mid-peace (G). Scale bar is equal to 100 µm.
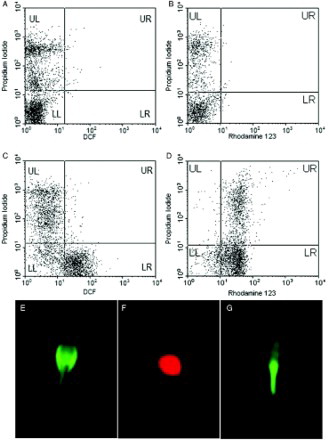
Table 1. Descriptive analyses of parameters in male patients (n=40).
To verify the location of ROS production, we used fluorescence microscopy imaging (E-G). E shows the localization of green fluorescent DCF in ROS+ sperm (cytoplasmic ROS). F shows the localization of red PI fluorescent in a dead sperm and Figure G shows accumulation of green fluorescent R123 in the sperm's mid-piece, which contains sperm mitochondria.
We used the YO-Pro-1/propidium iodide (Y1/PI) assay to assess apoptosis (), which differentiated apoptotic sperm (Y1 + /PI-) from necrotic sperm (Y1 + /PI+ or Y1-/PI+). Correlation analysis revealed a significant positive correlation between the percentage of DCF+ sperm (cytoplasmic ROS) with the percentage of CMA3+ sperm (r = +0.417, p = 0.007, ) and sperm motility (r = +0.366, p = 0.020, ) while the percentage of DCF+ sperm (cytoplasmic ROS) showed a significant negative association with apoptotic sperm [Y1 + /PI- (r = - 0.528, p < 0.001, )]. However, Y1 + /PI- (apoptotic sperm) revealed no significant relation with the percentage of CMA3+ sperm.
Figure 2. Evaluation of apoptosis with Yo-Pro-1 (Y1)/propidium iodide (PI) staining by flow cytometry in human spermatozoa. A) shows the unstained sample as a negative control, B) shows PI staining, and C) shows apoptotic sperm population in the lower right (LR) quadrant of the dot plot.
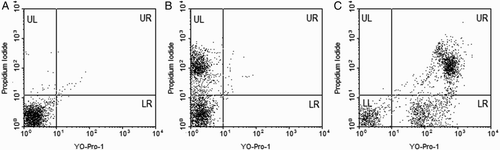
Table 2. Correlations between reactive oxygen species (ROS), apoptosis, chromomycin A3 (CMA3), and sperm parameters as measured with dichlorofluoresceindiacetate (DCFH-DA) using flow cytometry (FCM) in total, normozoospermia, and non-normozoospermia populations.
Similar to cytoplasmic ROS, the percentage of R123+ viable sperm showed a significant positive correlation with CMA3 (r = 0.317, p = 0.046, ), motility (r = 0.332, p = 0.036, ), and viability (r = 0.567, p = 0.000). There was a negative correlation between this parameter and apoptosis (r = - 0.475, p = 0.002, ). When individuals were divided into normozoospermia and non-normozoospermia groups according to WHO [2010] criteria, a similar and even stronger trend was observed in the latter group with total population while this trend was not observed with non-normozoospermia (Tables and ).
Table 3. Correlations between reactive oxygen species (ROS), apoptosis, CMA3, and sperm parameters as measured with DHR123 using flow cytometry (FCM) in total, normozoospermia, and non-normozoospermia populations.
Discussion
Oxidative stress, poor chromatin condensation, and apoptosis are among the major factors that contribute to sperm DNA damage [Agarwal and Said 2003; Shamsi et al. 2008; Sharma et al. 2004]. Although the major source of ROS is external, sperm can innately produce ROS from the mitochondria or cytoplasm [Agarwal and Saleh 2002; Koppers et al. 2008]. The cytosolic origin of ROS is mainly associated with cytoplasmic retention, which is considered a sign of immature sperm [Huszar et al. 1997]. Shedding cytoplasm is believed to take place during the late stages during spermiogenesis. Concomitant with this shedding, the sperm chromatin undergoes specific remolding during which histones are replaced with protamines, resulting in chromatin condensation [Oliva 2006]. One of the aims of this study was to determine if there is an association between protamination and ROS origin in sperm.
The results of this study have revealed a positive association between the percentage of CMA3+ sperm with the percentages of both cytoplasmic (DCF+ sperm) and mitochondrial (R123+ sperm) ROS. This result has suggested that abnormal ROS production is associated with poor chromatin condensation. Thus, this phenomenon may be associated with overall aberrant sperm function, rather than being specific to a particular sperm compartment, since the percentage of CMA3+ sperm significantly correlated with both cytoplasmic (DCF) and mitochondrial (R123) ROS. Furthermore, in agreement with our previous work, the percentage of DCF+ and R123+ sperm were strongly correlated with each other [Kiani-Esfahani et al. 2012], possibly suggesting that ROS might arise from general dysfunction of sperm. Aziz et al. [2010] also observed a positive correlation between ROS production assessed by chemiluminescence with percentage of R123+ sperm. It has been proposed that poor chromatin remodeling and increased ROS possibly render DNA more susceptible to damage [De Iuliis et al. 2009]. Henkel et al. [2010] have also obtained a positive association between ROS production and CMA3+ sperm. A significant correlation between the percentage of 8-OHdG-positive sperm with under protamination and reduced mitochondrial membrane potential has been reported [De Iuliis et al. 2009]. Taken together, our observations and these reports further reiterates the notion that under protamination makes sperm prone to DNA fragmentation. Ramos et al. [2008] have suggested that “both over oxidation and a low total number of thiol groups predestine sperm for apoptosis.” They further stated that lower total thiol group is associated with reduce protamine content. In regard to this, Tunc et al. [2009] have shown that optimal dietary antioxidant supplements improves under protamination. They further stated that the concentration of vitamin C supplements is of importance because a high concentration of vitamin C could act as a reducing agent and interfere with the disulfide bonds between the protamines. Such paradoxes are called the ‘antioxidant paradox’ (reviewed in [Henkel 2011]).
This study revealed a positive correlation between the percentage of cytoplasmic (DCF+ sperm) and mitochondrial (R123+ sperm) ROS with sperm viability and motility. This suggests that viable sperm can produce ROS, which is consistent with previous reports [Aitken et al. 2010; Kiani-Esfahani et al. 2012; Koppers et al. 2008]. However, almost all the DCF+ sperm were PI negative while unlike DCF+ sperm, a portion of R123+ sperm were PI positive. Possibly, in such spermatozoa, the membrane has lost its integrity while the mitochondria may have preserved its function, since they present mitochondrial membrane potential (MMP). This suggests that PI + /R123 + sperm may have entered the process of apoptosis and, furthermore, R123 is sensitive to minimal MMP while in dead sperm DCF leaks out of the sperm and therefore a lower number of sperm is simultaneously PI + /DCF+. Therefore, we assessed the relationship between the percentages of Y1 + /PI- (a maker of early apoptosis) with the percentage of R123+ sperm, and observed a positive correlation with the percentage of PI + /R123+ sperm, and a negative correlation with the PI-/R123+ sperm. Similarly, a negative correlation was observed between Y1 + /PI- sperm with DCF+ sperm. These results are in contrast to the expectation or general hypothesis that increased ROS is positively linked with apoptosis [Barroso et al. 2000; Grunewald et al. 2009; Paasch et al. 2004; Wang et al. 2003]. However, this observation reflects the fact that for apoptosis to take place an initial rise in ROS occurs and its deleterious effect is subsequent to this increase in ROS. Early events of apoptosis, including the loss of membrane semi-permeability occur during this period, consistent with the above results. These results collectively suggest that as sperm undergo apoptosis, they become PI+. Henkel et al. [2010] also assessed the relation between ROS production and DNA fragmentation using the TUNEL assay. Unlike the correlations observed between ROS production with apoptosis, they did not observe a correlation between ROS and DNA fragmentation [Henkel et al. 2010]. This difference might be due to the fact that TUNEL assesses late apoptosis, while Y1 + /PI- assesses early apoptosis. Therefore, the functional activity of enzymes producing ROS might have been lost by later stages of apoptosis. In this regard, according to Aitken et al. [2010]:
“(the) loss of viability is part of a progressive oxidative process that begins with the excessive generation of ROS, leads to lipid peroxidation and oxidative DNA damage, and culminates in DNA fragmentation and death. Whether oxidatively damaged cells are alive or dead is just a question of the time that has elapsed between the initiation of oxidative stress and the point of assessment” [Aitken et al. 2010, 2424].
In this study, there was no relation between protamine deficiency and apoptosis. This observation might be explained by the above hypothesis [Aitken et al. 2010] which has stated that executive events in apoptosis are time dependent or ROS production is independent of the degree of protamination, and the relation between ROS production and CMA3 is merely a concomitant event rather than a causative phenomenon.
The correlation between apoptosis and both cytoplasmic (DCF) and mitochondrial (R123) ROS suggests that apoptosis induced by ROS is independent of its origin, despite the fact that both mitochondria and cytoplasm are generally accepted as separate compartments. Cytoplasmic ROS primarily contributes to the NADPH-oxidase system at the cell membrane and should be mainly detected by DCFH-DA, while mitochondrial ROS (which is attributed to the leakage of ROS from an electron chain reaction) should be mainly detected by DHR123 [Kurozumi et al. 2005; Wei et al. 2000]. Therefore, apoptosis appears to be independent of ROS origin, since both these factors are correlated with apoptosis. However, one should consider the leakage of ROS from mitochondria to cytoplasm.
Considering the independence of ROS origin with both protamine deficiency and apoptosis, these results may suggest that general sperm dysfunction is the likely reason for sperm apoptosis. In this regard, previous studies suggest that apoptosis is a normal physiological event in germ cells for the purpose of limiting the population of such germ cells and the depletion of abnormal germ cells in spermatogenesis [Sakkas et al. 2003; Tarozzi et al. 2007]. Therefore, it is likely that ROS may directly or indirectly be involved in limiting the germ cell population. Sperm bypassing this system may produce high levels of ROS. This phenomenon could potentially explain the relationship between sperm immaturity assessed by protamine deficiency and ROS production, which may account for DNA damage in these cells.
The approach of this study is correlative analysis to determine whether DNA damage or apoptosis is a consequence of ROS production or sperm immaturity like protamine deficiency. Furthermore, when individuals were grouped according to WHO [2010] criteria to normozoospermia and non-normozoospermia, correlation analysis revealed stronger relationships between the aforementioned parameters in normozoospermic individuals compared to the total population. However, it was very interesting to note that such relations were not observed in the non-normozoospermic individuals. The difference between normozoospermic and non-normozoospermic individuals suggests that the conclusion derived in this study is valid. Such an observation on its own is very interesting and further insight into the difference observed between normozoospermia and non-normozoospermia may shed more light on the mechanism that results in aberrant semen parameters in infertile individuals.
In conclusion, the results of this study suggest that ROS production in sperm is likely to be a general event and is not uniquely related to the cytoplasm or mitochondria. The higher production of ROS in immature or protamine deficient cells, predispose cells to higher levels of ROS and thereby DNA damage. Higher ROS production is likely to be related to general apoptosis in germ cells and immature sperm that may have bypassed this system. Accordingly the underlying mechanism likely fails in individuals with abnormal semen parameters. Whether this is the cause or consequence of abnormal semen parameters remains to be determined.
Materials and Methods
Sample collection and preparation
Semen samples were collected from 40 infertile men that were referred to the andrology unit of the Isfahan Fertility and Infertility Center. Their age ranged between 25 to 47 years. Informed consent forms were obtained from all participants in this study. Ethical issues were raised with all participants, and all confidentiality rights were discussed. Semen parameters were analyzed in accordance to World Health Organization criteria [WHO 2010]. Initially, samples were washed twice in phosphate buffer saline (PBS, Gibco, USA), at 400 × g for 5 min and then diluted according to experimental procedure. Semen samples with more than 1 million leucocytes, or a sign of genital infection were excluded from the study. The study received approval by the Royan Ethics Committee.
Assessment of cytosolic ROS by DCFH-DA
DCFH-DA (Sigma Co, USA) detects cytosolic ROS including hydrogen peroxide [Carter et al.1994; Kurozumi et al. 2005; LeBel et al. 1992; Teixeira et al. 1998; Wei et al. 2000]. DCFH-DA staining was carried out according to our previous work [Kiani-Esfahani et al. 2012]. Briefly, one million sperm per ml PBS was incubated with 0.5 µM DCFH-DA at room temperature in a dark setting for 40 min, after which we used flow cytometry to determine the percentage of DCF+ sperm.
Assessment of mitochondrial ROS by DHR123
DHR123 (Sigma Co, USA) was employed to measure mitochondrial ROS (superoxide, hydrogen peroxide, and peroxynitrite) [Kurozumi et al. 2005; Lievre et al. 2001; O'Connell et al. 2002; Rego et al. 1999; Wei et al. 2000]. DHR123 is able to freely penetrate cell membrane and is oxidized by ROS in mitochondria to produce the green fluorescence R123. Measurement of mitochondrial ROS by DHR123 was carried out according to our previously established protocol [Kiani-Esfahani et al. 2012]. Briefly, one million sperm per ml PBS was incubated with 0.05 µM DHR123 at room temperature in the dark for 20 min.
Assessment of apoptosis
The fluorophore Y1 (Sigma Co, USA) was used to evaluate early changes in sperm membrane integrity for an early sign of apoptosis. Yo-Pro-1 with PI (Sigma Co, USA) can differentiate between apoptotic, necrotic, and live cells [Idziorek et al. 1995; Martin et al. 2005; Martinez-Pastor et al. 2008]. Apoptosis was measured by assessment of the percentage of Y1 + /PI-. For this purpose, 15 µl of 0.01 µM Y1 solution was added to one million sperm per ml PBS at room temperature in a dark setting for 15 min. Next, 1 µl of PI solution (50 µg/ml) was added to the sperm suspension and flow cytometry (FCM) analysis was performed.
Assessment of DNA protamination
The CMA3 (Sigma Co, USA) assay was assessed according to a previous report [Tavalaee et al. 2010]. In this manner, the sperm suspension was adjusted to 2 × 106 sperm/ml PBS. The samples were centrifuged at 400 × g for 5 min and fixed with Carnoy's solution, after which we added 200 µl of CMA3 solution (0.25 µg/ml PBS) to the sperm pellet at room temperature for 60 min. After washing, the specimens were analyzed by FCM.
Flow cytometry analysis
We used a FACS Calibur flow cytometer (Becton Dickinson, San Jose, CA, USA) equipped with a 488- nm argon laser to analyze all fluorescence signals of the labeled spermatozoa. A total of 10,000 events were recorded at a light scatter to exclude debris and aggregate with a flow rate of less than 200 cells/second for each assay. Green fluorescence (DCF and Y1) and red fluorescence (CMA3 and PI) were detected in FL-1 channel with 530/30 nm band-pass filter and FL-2 channel with 585/42 nm band-pass filter, respectively. The compensations and the settings were adjusted according to the assay utilized. Data were analyzed using BD CellQuest Pro software.
Statistical methods
All statistical analyses were assessed using SPSS 18.0 software. The associations between ROS variation and other variables were carried out using Pearson correlation coefficients. A p-value of p < 0.05 was considered statistically significant.
Abbreviations
ROS: | = | reactive oxygen species |
DCFH-DA: | = | dichlorofluoresceindiacetate |
DHR123: | = | dihydrorhodamine 123 |
CMA3: | = | chromomycin A3 |
Y1/PI: | = | YO-Pro-1/propidium iodide |
FCM: | = | flow cytometry. |
Acknowledgments
We would like express our gratitude to our colleagues at Royan Institute for their financial support and at the Isfahan Fertility and Infertility Center for their kind co-operation.
Declaration of interests: The authors have no financial or commercial conflicts with this project. Royan Institute is an NGO (Nongovernmental organization) belong to ACECR (Iranian Academic Center for Education, Culture & Research). The authors state that no authors are employed in any respect by the Government of Iran.
Author contributions: Experimental design, flow cytometry analyses, preparation of manuscript: AK-E; Experimental analyses and obtaining consent form: SB; Experimental analyses: MT; Experimental analyses: MRD; Supervision and writing the manuscript; MHN-E.
References
- Acharya, U.R., Acharya, S. and Mishra, M. (2003) Lead acetate induced cytotoxicity in male germinal cells of Swiss mice. Ind Health 41(3):291–294.
- Agarwal, A. and Said, T.M. (2003) Role of sperm chromatin abnormalities and DNA damage in male infertility. Hum Reprod Update 9(4):331–345.
- Agarwal, A. and Saleh, R.A. (2002) Role of oxidants in male infertility: rationale, significance, and treatment. Urol Clin North Am 29(4):817–827.
- Agarwal, A., Saleh, R.A. and Bedaiwy, M.A. (2003) Role of reactive oxygen species in the pathophysiology of human reproduction. Fertil Steril 79:829–843.
- Aitken, R.J. and West, K.M. (1990) Analysis of the relationship between reactive oxygen species production and leukocyte infiltration in fractions of human semen separated on Percoll gradients. Int J Androl 3:433–451.
- Aitken, R.J., De Iuliis, G.N., Finnie, J.M., Hedges, A. and McLachlan, R.I. (2010) Analysis of the relationships between oxidative stress, DNA damage and sperm vitality in a patient population: development of diagnostic criteria. Hum Reprod 25(10):2415–2426.
- Aitken, R.J., Fisher, H.M., Fulton, N., Gomez, E., Knox, W., Lewis, B., (1997) Reactive oxygen species generation by human spermatozoa is induced by exogenous NADPH and inhibited by flavoprotein inhibitors diphenylene iodinium and quinacrine. Mol Reprod Dev 47:468–482.
- Aitken, R.J., Gordon, E., Harkiss, D., Twigg, J.P., Milne, P., Jennings, Z., (1998) Relative impact of oxidative stress on the functional competence and genomic integrity of human spermatozoa. Biol Reprod 59:1037–1046.
- Aoki, V.W. and Carrell, D.T. (2003) Human protamines and the developing spermatid: their structure, function, expression and relationship with male infertility. Asian J Androl 5:315–324.
- Aziz, N., Novotny, J., Oborna, I., Fingerova, H., Brezinova, J. and Svobodova, M. (2010) Comparison of chemiluminescence and flow cytometry in the estimation of reactive oxygen and nitrogen species in human semen. Fertil Steril 94(7):2604–2608.
- Barroso, G., Morshedi, M. and Oehninger, S. (2000) Analysis of DNA fragmentation, plasma membrane translocation of phosphatidylserine and oxidative stress in human spermatozoa. Hum Reprod 15:1338–1344.
- Bianchi, P.G., Manicardi, G.C., Bizzaro, D., Bianchi, U. and Sakkas, D. (1993) Effect of deoxyribonucleic acid protamination on fluorochrome staining and in situ nick-translation of murine and human mature spermatozoa. Biol Reprod 49:1083–1088.
- Borini, A., Tarozzi, N., Bizzaro, D., Bonu, M.A., Fava, L., Flamigni, C., (2006) Sperm DNA fragmentation: paternal effect on early post-implantation embryo development in ART. Hum Reprod 21:2876–2881.
- Carrell, D.T., Liu, L., Peterson, C.M., Jones, K.P., Hatasaka, H.H., Erickson, L., (2003) Sperm DNA fragmentation is increased in couples with unexplained recurrent pregnancy loss. Arch Androl 49:49–55.
- Carter, W.O., Narayanan, P.K. and Robinson, J.P. (1994) Intracellular hydrogen peroxide and superoxide anion detection in endothelial cells. J Leukoc Biol 55:253–258.
- De Iuliis, G.N., Thomson, L.K., Mitchell, L.A., Finnie, J.M., Koppers, A.J., Hedges, A., (2009) DNA Damage in Human Spermatozoa Is Highly Correlated with the Efficiency of Chromatin Remodeling and the Formation of 8-Hydroxy-2'-Deoxyguanosine, a Marker of Oxidative Stress. Biol Reprod 81:517–524.
- de Lamirande, E. and Gagnon, C. (1995) Impact of reactive oxygen species on spermatozoa: a balancing act between beneficial and detrimental effects. Hum Reprod 10:15–21.
- Grunewald, S., Sharma, R., Paasch, U., Glander, H.J. and Agarwal, A. (2009) Impact of caspase activation in human spermatozoa. Microsc Res Tech 72:878–888.
- Henkel, R.R. (2011) Leukocytes and oxidative stress: dilemma for sperm function and male fertility. Asian J Androl 13(1):43–52.
- Henkel, R., Bastiaan, H.S., Schüller, S., Hoppe, I., Starker, W. and Menkveld, R. (2010) Leucocytes and intrinsic ROS production may be factors compromising sperm chromatin condensation status. Andrologia 42(2):69–75.
- Henkel, R., Hajimohammad, M., Stalf, T., Hoogendijk, C., Mehnert, C., Menkveld, R., (2004) Influence of deoxyribonucleic acid damage on fertilization and pregnancy. Fertil Steril 81:965–972.
- Henkel, R., Kierspel, E., Stalf, T., Mehnert, C., Menkveld, R., Tinneberg, H.R., (2005) Effect of reactive oxygen species produced by spermatozoa and leukocytes on sperm functions in nonleukocytospermic patients. Fertil Steril 83:635–642.
- Huszar, G., Sbracia, M., Vigue, L., Miller, D.J. and Shur, B.D. (1997) Sperm plasma membrane remodeling during spermiogenic maturation in men: relationship among plasma membrane beta 1,4-galactosyltransferase, cytoplasmic creatine phosphokinase and creatine phosphokinase isoform ratios. Biol Reprod 56:1020–1024.
- Idziorek, T., Estaquier, J., De Bels, F. and Ameisen, J.C. (1995) YOPRO-1 permits cytofluorometric analysis of programmed cell death (apoptosis) without interfering with cell viability. J Immunol Methods 185:249–258.
- Kasahara, E., Sato, E.F., Miyoshi, M., Konaka, R., Hiramoto, K., Sasaki, J., (2002) Role of oxidative stress in germ cell apoptosis induced by di(2-ethylhexyl)phthalate. Biochem J 365(Pt 3):849–856.
- Kessopoulou, E., Tomlinson, M.J., Banat, C.L., Bolton, A.E. and Cooke, I.D. (1992) Origin of reactive oxygen species in human semen spermatozoa or leukocytes. J Reprod Fertil 94:463–470.
- Kiani-Esfahani, A., Tavalaee, M., Deemeh, M.R., Hamiditabar, M. and Nasr-Esfahani, M.H. (2012) DHR123: an alternative probe for assessment of ROS in human spermatozoa. Syst Biol Reprod Med 58(3):168–174.
- Koch, O.R., Pani, G., Borrello, S., Colavitti, R., Cravero, A., Farrè, S., (2004) Oxidative stress and antioxidant defenses in ethanol-induced cell injury. Mol Aspects Med 25(1–2):191–198.
- Kodama, H., Yamaguchi, R., Fukuda, J., Kasai, H. and Tanaka, T. (1997) Increased oxidative deoxyribonucleic acid damage in the spermatozoa of infertile male patients. Fertil Steril 68:519–524.
- Koppers, A.J., De Iuliis, G.N., Finnie, J.M., McLaughlin, E.A. and Aitken, R.J. (2008) Significance of mitochondrial reactive oxygen species in the generation of oxidative stress in spermatozoa. J Clin Endocrinol Metab 93(8):3199–3207.
- Kothari, S., Thompson, A., Agarwal, A. and du Plessis, S.S. (2010) Free radicals: their beneficial and detrimental effects on sperm function. Indian J Exp Biol 48(5):425–435.
- Kurozumi, R., Takahashi, M. and Kojima, S. (2005) Involvement of mitochondrial peroxynitrite in nitric oxide-induced glutathione synthesis. Biol Pharm Bull 28(5):779–785.
- LeBel, C.P., Ischiropoulos, H. and Bondy, S.C. (1992) Evaluation of the probe 2',7'- dichlorofluorescein as an indicator of reactive oxygen species formation and oxidative stress. Chem Res Toxicol 5:227–231.
- Lewis, S.E. and Aitken, R.J. (2005) DNA damage to spermatozoa has impacts on fertilization and pregnancy. Cell Tissue Res 322:33–41.
- Lievre, V., Becuwe, P., Bianchi, A., Bossenmeyer-Pourie, C., Koziel, V., Franck, P., (2001) Intracellular generation of free radicals and modifications of detoxifying enzymes in cultured neurons from the developing rat forebrain in response to transient hypoxia. Neuroscience 105:287–297.
- Manicardi, G.C., Bianchi, P.G., Pantano, S., Azzoni, P., Bizzaro, D., Bianchi, U., (1995) Presence of endogenous nicks in DNA of ejaculated human spermatozoa and its relationship to chromomycin A3 accessibility. Biol Reprod 52:864–867.
- Martin, G., Sabido, O., Durand, P. and Levy, R. (2005) Phosphatidylserine externalization in human sperm induced by calcium ionophore A23187: relationship with apoptosis, membrane scrambling and the acrosome reaction. Hum Reprod 20:3459–3468.
- Martinez-Pastor, F., Fernandez-Santos, M.R., del Olmo, E., Dominguez-Rebolledo, A.E., Esteso, M.C., Montoro, V., (2008) Mitochondrial activity and forward scatter vary in necrotic, apoptotic and membrane-intact spermatozoan subpopulations. Reprod Fertil Dev 20:547–556.
- O'Connell, M., McClure, N. and Lewis, S.E. (2002) The effects of cryopreservation on sperm morphology, motility and mitochondrial function. Hum Rep 17:704–709.
- Oger, I., Da Cruz, C., Panteix, G. and Menezo, Y. (2003) Evaluating human sperm DNA integrity: relationship between 8-hydroxydeoxyguanosine quantification and the sperm chromatin structure assay. Zygote 11:367–371.
- Oliva, R. (2006) Protamines and male infertility. Hum Reprod Update 12:417–435.
- Paasch, U., Grunewald, S., Agarwal, A. and Glandera, H.J. (2004) Activation pattern of caspases in human spermatozoa. Fertil Steril 81( Suppl 1):802–809.
- Poccia, D. (1986) Remodeling of nucleoproteins during gametogenesis, fertilization and early development. Int Rev Cytol 105:1–65.
- Ramos, L., van der Heijden, G.W., Derijck, A., Berden, J.H., Kremer, J.A.M., van der Vlag, J., (2008) Incomplete nuclear transformation of human spermatozoa in oligoastheno-teratozoospermia: characterization by indirect immunofluorescence of chromatin and thiol status. Hum Reprod 23:259–270.
- Rego, A.C., Santos, M.S. and Oliveira, C.R. (1999) Influence of the antioxidants vitamin E and idebenone on retinal cell injury mediated by chemical ischemia, hypoglycemia, or oxidative stress. Free Radic Biol Med 26(11–12):1405–1417.
- Sakkas, D., Seli, E., Bizzaro, D., Tarozzi, N. and Manicardi, G.C. (2003) Abnormal spermatozoa in the ejaculate: abortive apoptosis and faulty nuclear remodelling during spermatogenesis. Reprod Biomed Online 7(4):428–432.
- Saleh, R.A., Agarwal, A., Kandirali, E., Sharma, R.K., Thomas, A.J., Nada, E.A., (2002a) Leukocytospermia is associated with increased reactive oxygen species production by human spermatozoa. Fertil Steril 78:1215–1224.
- Saleh, R.A., Agarwal, A., Sharma, R.K., Nelson, D.R. and Thomas, A.J., Jr. (2002b) Effect of cigarette smoking on levels of seminal oxidative stress in infertile men: a prospective study. Fertil Steril 78(3):491–499.
- Shamsi, M.B., Kumar, R. and Dada, R. (2008) Evaluation of nuclear DNA damage in human spermatozoa in men opting for assisted reproduction. Indian J Med Res 127:115–123.
- Sharma, R.K., Said, T. and Agarwal, A. (2004) Sperm DNA damage and its clinical relevance in assessing reproductive outcome. Asian J Androl 6:139–148.
- Shekarriz, M., Sharma, R.K., Thomas, A.J., J.r. and Agarwal, A. (1995) Positive myeloperoxidase staining (Endtz Test) as an indicator of excessive reactive oxygen species formation in semen. J Assist Reprod Genet 12:70–74.
- Tarozzi, N., Bizzaro, D., Flamigni, C. and Borini, A. (2007) Clinical relevance of sperm DNA damage in assisted reproduction. Reprod Biomed Online 14(6):746–757.
- Tavalaee, M., Kiani, A., Arbabian, M., Deemeh, M.R. and Nasr Esfahani, M.H. (2010) Flow Cytometry: A New Approach for Indirect Assessment of Sperm Protamine Deficiency. Int J Fertil Steril 3(4):177–184.
- Teixeira, H.D., Schumacher, R.I. and Meneghini, R. (1998) Lower intracellular hydrogen peroxide levels in cells overexpressing CuZn-superoxide dismutase. Proc Natl Acad Sci USA 95(14):7872–7875.
- Tunc, O., Thompson, J. and Tremellen, K. (2009) Improvement in sperm DNA quality using an oral antioxidant therapy. Reprod Biomed Online 18(6):761–768.
- Wang, X., Sharma, R.K., Sikka, S.C., Thomas, A.J., Jr., Falcone, T. and Agarwal, A. (2003) Oxidative stress is associated with increased apoptosis leading to spermatozoa DNA damage in patients with male factor infertility. Fertil Steril 80:531–535.
- Wei, T., Chen, C., Hou, J., Xin, W. and Mori, A. (2000) Nitric oxide induces oxidative stress and apoptosis in neuronal cells. Biochim Biophys Acta 1498(1):72–79.
- WHO (2010) World Health Organization laboratory manual for the examination and processing of human semen. 5th ed. WHO press, Geneva, Switzerland.