Abstract
Sperm cells are complicated in vitro models. Their viability is limited, and physiology is complex. The study of their properties is of great application in the animal production as viable and functional gametes are essential. It has been shown that the decrease of sperm cell viability parallels an increase of the reactive oxygen species (ROS). Reactive oxygen species is secondary to normal metabolic processes of the cell-like flagellar movement. There is evidence of strategies that reduce ROS levels by using exogenous or endogenous antioxidants with the intention that seminal plasma protects the sperm cells and increases viability. Perhaps viability can increase by reducing that flagellar movement which is regulated by calcium. The phenomenon has not been fully characterized, but it is established that in certain mammalian models, the entrance of calcium via specific channels such as CATsper or voltage-dependent channels, signals flagellar movement. Previous reports have indicated that a change in the concentration of calcium or if the temperature is altered, the function of mammal sperm cells is reduced or blocked and viability prolonged. Fish sperm can remain immobile for several weeks but when activated the number of mobile and viable sperm is reduced at a faster rate. However, if the cells are not mobilized the semen can be preserved for longer periods. As presented in this paper, this supports the notion that by modulating calcium channels to reduce motility the viability of these cells can increase.
Sperm capacitation
Fertilization is a unique and amazing process involving two morphologically distinct cells, the sperm and the oocyte, which are recognized and fused together. This process begins when the sperm starts to penetrate the oocyte envelope and plasma membrane and ends in the exchange of maternal and paternal chromosomes, forming the zygote [Patrat et al. Citation2006]. The sperm must undergo functional changes following its genesis and subsequent maturation in the epididymis. Only sperm that have become capacitated can recognize and bind to the zona pellucida (ZP). The interaction between the sperm and the ZP initiates a signal transduction process resulting in exocytosis of the acrosomal contents during the acrosome reaction (AR) [Breitbart Citation2003; Rossato et al. Citation2001]. However, this is only a general picture of the AR phenomenon, and some reports have suggested that an intact ZP is not sufficient to induce acrosomal exocytosis [Baibakov et al. Citation2007]. Furthermore, according to the work of Dr. Yanagimachi’s group, some mouse sperm passing through the cumulus layers are already undergoing or have completed the acrosome reaction [Knobil and Neill Citation1994]. In shrews, the acrosome reaction is induced by cumulus cells, but not by the ZP [Bedford et al. Citation2004]. The available evidence suggests a general but not unique mechanism of penetration, and it is important to consider particular species adaptations when manipulating different samples in vitro. The sperm must penetrate physical barriers imposed by the oocyte, including cumulus oophorus cells, the plasma membrane, and the ZP, for which hydrolytic enzymes such as glycohydrolases and proteinases are necessary. During capacitation, the sperm undergoes functional biochemical and biophysical modifications, including changes in the activity of membrane enzymes and motility patterns, enabling it to undergo the AR prior to fertilization. These modifications include the removal of roadblocks to capacitation factors from the sperm surface and increased membrane fluidity, cholesterol efflux, intracellular calcium, cAMP, and protein tyrosine phosphorylation [Aitken and McLaughlin Citation2007]. All of these processes are regulated by the entry of calcium into the cells, but another key factor is the motility of the sperm. The complete process had previously been described as a single step: the entry of calcium increases motility and the AR. However, recently, the calcium wave concept has been incorporated (see ; [Navarrete et al. Citation2010]), indicating that in sperm cells, the first part of the calcium wave generates an increase in motility, while the second part induces the AR. Furthermore, this process can be manipulated while the cells maintain a healthy state [Darszon et al. Citation2011].
Figure 1. Calcium wave. The upper panel shows a bovine sperm with a calcium probe exposed to a high potassium concentration, while the lower panel shows a graphic representation of the fluorescence intensity, both in control conditions and when sperm are exposed to potassium. The figure indicates that there is a wave from the middle piece to the head when the sperm are depolarized. Modified figure from Navarrete et al. 2010.
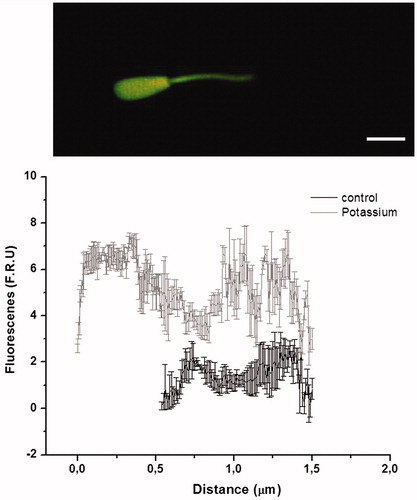
Capacitation factors
The reduction of factors inhibiting capacitation (disinhibit factor, DF) due to the seminal flow involves a gradual release of these factors, from the sperm surface. Their release results in a transient state of sperm DF capacitation. This ensures the maximum capacity of fertilization at the appropriate location [Acott and Carr Citation1984; Zhong et al. Citation1993]. Once the DF binds to the sperm surface, it activates a calcium ATPase, thus maintaining a low calcium concentration. When the DF is released from the sperm surface, an increase in intracellular calcium levels is initiated. In vitro studies in which the calcium ATPase was inhibited revealed acceleration of capacitation [Perry et al. Citation1997].
Plasma membrane and ion channels
The plasma membrane is a lipoprotein interface that acts as a permeability barrier allowing the cell to maintain a different composition in the intracellular in comparison to the extracellular medium. The most abundant components of the plasma membrane are phospholipids and proteins, which together form the fluid mosaic pattern [Hasdemir Citation2007]. The resting potential is a particular state of the membrane potential in which the sum of ion currents through the membrane is zero. This is due to the presence of transmembrane electrochemical gradients resulting from selective permeability to ions and secondary various structures such as transmembrane channels, pumps, and ion exchangers. From the resting potential, cell excitation can generate an action potential that allows the cell to respond to different stimuli. During this process, each ion tends to draw the membrane potential towards its own electrochemical equilibrium potential (Nernst equation) [Hille Citation1992]. Ionic currents through channels determine transmembrane bioelectric phenomena related to the membrane potential in addition to modulating enzyme activity, metabolism, and cellular genetics activity. Specifically, in sperm cells, the transmembrane ionic currents and their potential, among other factors, regulate the intracellular concentration of calcium and the genesis of second messengers. These factors are essential for fertilization-associated processes, such as sperm motility, capacitation, and the AR. Therefore, the study of ion channels is extremely valuable for understanding the electrophysiological processes and biological responses of both excitable cells and isolated cells. In particular, determining the roles of these channels in the mammalian sperm membrane is essential to understand the processes involved in fertilization. The main tool for investigating the characteristics and distribution of ion channels in the plasma membrane is the patch-clamp technique [Neher and Sakmann Citation1976; Neher et al. Citation1978], which is a high-resolution method that is currently used to determine the electrophysiological and pharmacological properties of the cell structure.
Sperm cell viability and function
One must be careful during the various procedures in which sperm are manipulated as alterations can cause premature sperm capacitation [Gomez et al. Citation1997]. This leads to the AR impacting the longevity of the sperm. A decrease in fertilization capacity can result from the presence of large amounts of ROS following ejaculation. Kirchhoff and associates [Citation1998] and Alvarez and Agarwal [Citation2006] indicated that sperm produce and export ROS to the extracellular environment, most of which are generated by the mitochondria, secondary to the flagellar activity of the cells. The loss of sperm function, i.e., the fertilization capacity, results from the presence of high levels of ROS, either following ejaculation or secondarily to high levels of motility. These studies have indicated that the sperm produced and exported ROS to the extracellular environment are the product of the monovalent reduction of molecular oxygen during oxidative phosphorylation [Alvarez and Agarwal Citation2006; Kirchhoff et al. Citation1998]. Observations made in the laboratory in models of immobile sperm cells (salmon or trout) have suggested the existence of long-term viability, lasting for days or weeks, while retaining a high rate of fertilization. The common feature of such models is the inactive state of the cells, without metabolic changes, i.e., ROS production. In mammalian sperm cells, we have observed only a one-hour period of viability and function, and these cells show a high motility and metabolism. Temperature is important for the regulation of cell function, and the preservation and the quality of sperm. For example, fish semen display extreme temperature control [Alavi and Cosson Citation2005], and in porcine sperm, temperature conservation increases the time of preservation of a sample [Althouse et al. Citation1998]. Furthermore, in fowl, temperature regulates calcium influx [Thomson and Wishart Citation1991]. These lines of evidence suggest the importance of temperature control during the in vitro manipulation of sperm cells, which is correlated with changes during travel in the oviduct or in fresh water, in the case of the aquatic species. In particular, there may be temperature gradients within the oviduct of animals in estrous [Bahat and Eisenbach Citation2006; Hunter and Nichol Citation1986]. Values presented in the literature suggest that these gradients can be on the order of 1–2 °C or more between the caudal portion of the isthmus and the cranial portion of the ampulla in the hours before ovulation. This has been proposed to contribute to reducing sperm motility and the sperm storage function of the caudal isthmus [Hunter and Nichol Citation1986]. The magnitude of the temperature gradient may change according to the stage of the cycle and, especially, according to the time of ovulation [Hunter Citation2012]. Therefore, there is a possible influence of temperature on the viscosity and viscoelasticity of female tract fluids and on the ZP, as in other cell models, membrane viscosity is affected by temperature [Stokke et al. Citation1985]. This factor must be considered, and it might be most significant at the time when viable spermatozoa are expected to be found in the oviduct [Coy et al. Citation2009]. Temperature is a key factor in the function of sperm cells, and we can control it in vitro. Thus, we observed natural changes in the oviduct when the sperm cells are swimming towards the oocyte. Moreover, in aquatic species, environmental conditions are vital to fecundity.
Kv currents identified in sperm
A previous study revealed the presence of different types and differentially localized potassium channels [Darszon et al. Citation2006; Hagiwara and Kawa Citation1984]. An example is the delayed rectifier K+ type channel found in rat spermatogenic cells, which shows a trend that is independent of extracellular calcium and is blocked by tetraethylammonium chloride (TEA) [Hagiwara and Kawa Citation1984]. Based on these characteristics, we identified an inward rectifier K+ channel referred to as Kir [Munoz-Garay et al. Citation2001]. This channel is also regulated by the intracellular pH, with an acidic intracellular pH (6.3) inhibiting the current in spermatogenic cells, while a rising intracellular pH (7.4) significantly increases conductance in these cells. We further identified a third type of K+ channel, designated mSlo3, which was cloned in rat spermatogenic cells and has been expressed in Xenopus laevis oocytes for biophysical analyses. Recent studies using electrophysiological methods allowed an output current from the sperm midpiece that is sensitive to TEA to be detected [Marconi et al. Citation2008], and depolarization regulating calcium entry was described.
Regulation of calcium voltage channels (CAVs) during capacitation
During capacitation, ionic channels are susceptible to being activated when a change in the configuration of these channels occurs and are mediated by a change in the membrane potential. In rat and bovine sperm, the membrane potential is between −10 and −50 mV [Clapham et al. Citation2003; Darszon et al. Citation2005]. Low voltage calcium is inactivated at these voltages and therefore does not respond to depolarizing stimuli. Analysis of the membrane potential of rat spermatozoa showed that only cells that maintain hyperpolarization are able to generate an increased flow of calcium secondary to contact with the ZP (likely secondary CAVs) and carry out the RA [Arnoult et al. Citation1999]. Capacitation, resulting in hyperpolarization, changes the configuration of the CAV in a manner that is open to the agonist-mediated ion flow only at a specific stage, thus avoiding early RA. Studies in sperm conducted using electrophysiological methods have demonstrated the role of calcium channel functional are keys in capacitation, which are dependent on the membrane potential [Darszon et al. Citation2005; Wennemuth et al. Citation2000]. However, the complete mechanism underlying this phenomenon and its regulation via calcium entry is not completely understood. In this context, it was recently suggested that calcium entry occurs via depolarization and the regulation of motility, with a second entry event occurring due to pH regulation and depolarization, and this second calcium influx is mediated by the AR [Escoffier et al. Citation2007]. These findings have led to new models in which not only the type of CatSper channel is responsible for this phenomenon [Xia et al. Citation2007] but have further allowed the electrophysiological investigation of new phenomena, such as depolarization, that are also involved in the regulation of these voltage-dependent calcium channels.
Cationic sperm (CatSper) channels
Four members of the CatSper channels have been described (CatSper1-4) in murine sperm [Quill et al. Citation2001; Ren et al. Citation2001]. These channels consist of 6 transmembrane domains (6TM1) that are voltage-dependent and calcium-permeable and appear to be found only in sperm cells. CatSper1 and 2 channels have been reported to be essential for sperm hyperactivation and fertility. However, reports concerning these channels still mainly result from studies of humans and mice [Clapham and Garbers Citation2005].
Functional features of the plasma membrane of the sperm tail have been described [Ren et al. Citation2001]. Other reports have localized these proteins to the principal piece of the flagellum [Kirichok et al. Citation2006; Qi et al. Citation2007]. Additional evidence regarding the distribution of CatSper in different species and its localization in sperm cells is being obtained through ongoing investigations, which is important for designing solutions for the manipulation of samples. Studies in which the expression of this protein has been manipulated have led to the generation of a male sterile phenotype in a normal mouse model. While the mating behavior, sperm counts, and sperm cell morphology of these mutant mice are indistinguishable from those of wild type mice, the CatSper1 mutant sperm cells are slow, exhibit a reduced basal rate, and have no effect on the bathing or the bending of the tail region. The mutant sperm cells cannot fertilize the eggs with an intact zone pellucid but can fertilize eggs when the outer layers have been enzymatically removed [Ren et al. Citation2001], suggesting changes in some cell functions. Male mice lacking CatSper2 are also infertile due to a lack of the hyperactivated motility required for penetration of the extracellular matrix of the egg [Quill et al. Citation2003]. In a study in humans, subfertile men with deficient sperm motility showed significantly reduced expression of CatSper1 [Nikpoor et al. Citation2004]. Little is known about CatSper3 and CatSper4, but they appear to be involved in supporting cell functions in the sperm [Clapham and Garbers Citation2005].
The above leads to two questions: (1) can these channels explain all of the phenomena observed in the sperm cells? (2) is there sufficient evidence to support the idea that CatSper channels explain the entire model of the sperm activity? It is accepted that CatSper channels and their various isoforms are responsible for cellular functions in sperm. Additionally, the relationship between CatSper and progesterone has been described, and the authors indicated the effect of progesterone on increasing intracellular calcium levels [Blackmore Citation1993; Turner and Meizel Citation1995]. While the relationship between progesterone and CatSper has been described [Lishko et al. Citation2011; Strunker et al. Citation2011], the mechanism underlying the regulation of CatSper function by progesterone is not completely understood, although the intracellular PI3K-AKT signaling pathway was recently implicated in this process. However, progesterone may be associated with other receptors in sperm cells, such as GABAa [Shi and Roldan Citation1995], or in the regulation of another channel, such as potassium [Kumar et al. Citation2000], or voltage-dependent calcium channels [Bonaccorsi et al. Citation2001]. Progesterone has been described to play a role in the specific functions of sperm cell channels [Sagare-Patil et al. Citation2013]. Additionally, CatSper is modulated by pH [Fraire-Zamora and Gonzalez-Martinez Citation2004] and bicarbonate [Wennemuth et al. Citation2003]. Nevertheless, additional events must be coordinated for fecundation to occur successfully, including the AR, the regulation of membrane stability, calcium signaling, and mitochondrial function, among others, beyond Catsper modulation. However, these events are not described in all models, and other electrical phenomena can cooperate in the cellular events described in sperm. A complete table of ion channels, indicating the presence of voltage-dependent calcium channels and CatSper, in humans and mice is available [Darszon et al. Citation2011]. This review indicates that we lack a complete understanding of the localization of these channels, and there are other mechanisms that may alter intracellular calcium.
Changes observed in the membrane potential of sperm cells
An increase in the membrane potential, described as hyperpolarization, occurs during capacitation in rat, bovine, and human spermatozoa [Arnoult et al. Citation1996; Brewis et al. Citation2001; Zeng et al. Citation1996]. In rat sperm, hyperpolarization is the result of increased permeability to K+ [Zeng et al. Citation1995], leading to a change in the membrane potential. During capacitation, there is an increase in the pHi of more than 0.2 units [Zeng et al. Citation1996], which is sufficient to induce an increase of 0.5 to 3 times in the probability of the opening of Kir channels found in other tissues [Gutman et al. Citation2003]. Thus, under physiological conditions, an increase in pHi activates Kir channels. It has been suggested that this process hyperpolarizes the sperm membrane [Krasznai et al. Citation2000]. Furthermore, Kv-activated intracellular calcium is modulated by the increase in the concentration of intracellular calcium that occurs during capacitation, thus contributing to hyperpolarization [Jagannathan et al. Citation2002]. Together these observations confirm the role of K+ currents in the hyperpolarization of the sperm membrane and its effect on capacitation and the subsequent AR. However, in other cell models, the mechanism reflects blocking the K+ channel shaft, depolarization, and calcium channel opening [Baker et al. Citation1973; Wellman et al. Citation2001]. In sperm models, it is accepted that Kir channels are able to hyperpolarize the membrane, but these channels are controlled by physiological phenomena, leading to changes in the membrane potential and correcting this potential, allowing positive charges to be relocated to restore balance and maintain a physiological membrane potential [Gutman et al. Citation2003]. Kv-type channels are present in sperm [Marconi et al. Citation2008], and their current is modulated by peptides, suggesting a means to modulate currents in sperm [Parodi et al. Citation2010]. This model is sensitive to ASD and can be applied to generate depolarization in other cell models, leading to an increase in intracellular calcium levels and consequent cellular changes [Navarrete et al. Citation2010]. Some evidence suggests that this mechanism is part of a complex mechanism of regulation that also includes the hyperpolarization and depolarization described in sperm [Fraire-Zamora and Gonzalez-Martinez Citation2004; Gonzalez-Martinez Citation2003; Neri-Vidaurri Pdel et al. Citation2006], which can generate changes in the membrane potential, causing an influx of calcium and alterations in the physiology of sperm [Babcock and Pfeiffer Citation1987; Linares-Hernandez et al. Citation1998]. It is not hyperpolarization alone that mediates this phenomenon. The control of the membrane potential of sperm cells can block calcium entry and the associated secondary signaling. Many drugs can block changes in the membrane potential; could these drugs be used as potential regulators of sperm motility? A high concentration of potassium can induce changes in intracellular calcium levels, in the form of a wave from the middle piece to the head of the sperm. shows the effect of high potassium on intracellular calcium levels in bovine sperm (from [Navarrete et al. Citation2010]).
Calcium as a second messenger
The processes that generate second messengers that regulate cellular physiology have been studied for several years. Calcium is important for the regulation of kinase activity, phosphatases, gene activation, and protein translation. It is required at high concentrations for short periods of time, and cells display various mechanisms for finely regulating its intracellular concentration and maintaining a physiological calcium gradient [Hurwitz Citation1996; Stewart Citation1985]. Thus, various signals transiently increase intracellular calcium, which is indicative of activation of cellular processes, whereas a sustained increase in the cellular concentration indicates cell toxicity [Pounds Citation1984]. These changes in intracellular calcium concentrations may vary depending on cell type and the type of stimulation involved. Furthermore, they have been correlated with the process of vesicular exocytosis based on observations made through different techniques for measuring calcium currents and cell capacitance [Trifaro et al. Citation2000], including fluorometric measurements of calcium levels and amperometric records [Elhamdani et al. Citation1994]. For example, the normal process of vesicle release is highly dependent on calcium entry, which is crucial for the propagation of nerve impulses and the establishment of neural connections responsible for cognitive brain functions. In sperm cells, the intracellular activation of vesicles in the AR is similar to what is observed in other types of somatic cells and depends on changes in calcium levels. These findings suggest that different pathways leading to changes in calcium levels play a role in the development of different models of cell physiology.
Animal species of industrial interest
Understanding the influence of reproduction in food production is important in relation to increasing output and yield as well as maintaining and preserving genetic markers to improve productivity. Regarding the production of meat for consumption, cows, goats, pigs, and fish have been instrumental in the development of this industry. In recent years, assisted reproduction has begun to be applied in these species by preserving oocytes and sperm for later use in artificial insemination. The main reference models studied have been mice and humans, and similar techniques have been implemented in cows. Work aimed at the cryopreservation of sperm from salmon and other species was recently initiated, with sperm being frozen for transport, storage, and handling. There is high national and international demand for animal reproduction, as the meat market is steadily increasing, and the requirements for animal protein for human populations are also increasing [Food and Agriculture Organization of the United Nations, 2003]. The world population in 2030 will consume more and better food, with 3050 kilocalories (kcal) being available per person, compared to 2360 kcal per person/day in the mid-1960s and the 2800 kcal available currently. This change reflects the increase in consumption in many developing countries, whose average daily intake will be approximately 3000 kcal in 2030. For example, it has been reported that the domestic consumption of pork per person has increased [Oficina de Estudios y Politicas Agrarias, 2011], reaching values of 23 kg/capita in recent years. Thus, pork has become the second most commonly consumed meat, while poultry consumption decreased from 2000 to 2006 and has remained even at levels of 18 kg/capita over the last 4 years. The economic returns from the exploitation of animal flesh under current market conditions are based on the management of their genes and the use of high-genetic value players together with the best production techniques to obtain high-quality meat products at competitive cost. Reproduction is one of the most important aspects of the animal resource, as it allows the continuity of the species to be maintained. Additionally, the economic importance of reproductive behavior in cattle is well-known. Ingvartsen and Moyes [2013] summarized that essential studies examining the factors that affect the same traits will increase productivity in females. Thus, techniques including the control of insemination have begun to be viewed as an alternative for improving production, and the discussion regarding phenotypic traits of importance to the industry is increasing.
How do we maintain these gametes, increase cell function, and apply these techniques under various industrial conditions? This is not an easy question to answer, but the cellular functions of sperm related to generating such compounds as well as protocols and conditions applicable in this industry should be determined.
Mature sperm cells are complex cellular machines that through a series of steps and environments reach their target, the oocyte, and fulfill the purpose of delivering their genetic material via fertilization. In this review, we have highlighted flagellar motility and capacitation, which is characterized by the AR. In recent years, the function of CatSper channels as regulatory elements has shown to be indirectly involved in modulating the motility and fertilization capacity of sperm as well as calcium entry. A recent study has now demonstrated that a CatSper channel is involved in the motility but not in the AR [Sagare-Patil et al. Citation2013]. Flagellar movement generates various changes, including the production of ROS, and these increases can explain the reduction of cell viability. Moreover, some sperm cell models can remain immobile for a period of time. These sperm cells show a long period of viability and maintain their cellular functions for days. When activated, the cells become motile upon external signaling (i.e., osmotic changes). Calcium regulation is important for the general function of cells. In mammalian sperm cells, a recent study has suggested that there are two steps regulated by calcium entry: first, the motility of sperm cells, and second, the AR. Since motility generates ROS it is hypothesized here that regulation by calcium reduces the motility and the general metabolic state of the cells, leading to a reduction of cell mortality. All of these regulatory mechanisms are important for the conservation and manipulation of sperm cells. Because food production, and especially that of animal protein, has increased in recent decades, reproductive processes must be understood to provide an efficient means of control. It is vital for the development of the food industry to study these processes, yet little is known about the cells involved and the conditions that must occur. Thus, we should study other species as a reference for the development and maintenance of sperm as a function of process.
Declaration of interest
The author reports no conflicts of interest. The author alone is responsible for the content and writing of the paper.
Abbreviations | ||
ROS | = | reactive oxygen species |
ZP | = | zona pellucid |
AR | = | acrosome reaction |
DF | = | disinhibit factor |
TEA | = | tetraethylammonium chloride |
CAVs | = | calcium voltage channels |
CatSper | = | cationic sperm |
References
- Acott, T.S., and Carr, D.W. (1984) Inhibition of bovine spermatozoa by caudal epididymal fluid: II. Interaction of pH and a quiescence factor. Biol Reprod 30:926–35
- Aitken, R.J., and McLaughlin, E.A. (2007) Molecular mechanisms of sperm capacitation: progesterone-induced secondary calcium oscillations reflect the attainment of a capacitated state. Soc Reprod Fertil Suppl 63:273–93
- Alavi, S.M., and Cosson, J. (2005) Sperm motility in fishes. I. Effects of temperature and pH: a review. Cell Biol Int 29:101–10
- Althouse, G.C., Wilson, M.E., Kuster, C., and Parsley, M. (1998) Characterization of lower temperature storage limitations of fresh-extended porcine semen. Theriogenology 50:535–43
- Alvarez, J.G., and Agarwal, A. (2006) Development of a novel home sperm test - what are the limitations? Hum Reprod 21:3029–30; author reply 3030–3031
- Arnoult, C., Kazam, I.G., Visconti, P.E., Kopf, G.S., Villaz, M., and Florman, H.M. (1999) Control of the low voltage-activated calcium channel of mouse sperm by egg ZP3 and by membrane hyperpolarization during capacitation. Proc Natl Acad Sci USA 96:6757–62
- Arnoult, C., Zeng, Y., and Florman, H.M. (1996) ZP3-dependent activation of sperm cation channels regulates acrosomal secretion during mammalian fertilization. J Cell Biol 134:637–45
- Babcock, D.F., and Pfeiffer, D.R. (1987) Independent elevation of cytosolic [Ca2+] and pH of mammalian sperm by voltage-dependent and pH-sensitive mechanisms. J Biol Chem 262:15041–7
- Bahat, A., and Eisenbach, M. (2006) Sperm thermotaxis. Mol Cell Endocrinol 252:115–19
- Baibakov, B., Gauthier, L., Talbot, P., Rankin, T.L., and Dean, J. (2007) Sperm binding to the zona pellucida is not sufficient to induce acrosome exocytosis. Development 134:933–43
- Baker, P.F., Meves, H., and Ridgway, E.B. (1973) Calcium entry in response to maintained depolarization of squid axons. J Physiol 231:527–48
- Bedford, J.M., Mock, O.B., and Goodman, S.M. (2004) Novelties of conception in insectivorous mammals (Lipotyphla), particularly shrews. Biol Rev Camb Philos Soc 79:891–909
- Blackmore, P.F. (1993) Rapid non-genomic actions of progesterone stimulate Ca2+ influx and the acrosome reaction in human sperm. Cell Signal 5:531–8
- Bonaccorsi, L., Forti, G., and Baldi, E. (2001) Low-voltage-activated calcium channels are not involved in capacitation and biological response to progesterone in human sperm. Int J Androl 24:341–51
- Breitbart, H. (2003) Signaling pathways in sperm capacitation and acrosome reaction. Cell Mol Biol (Noisy-le-grand) 49:321–7
- Brewis, I.A., Morton, I.E., Moore, H.D., and England, G.C. (2001) Solubilized zona pellucida proteins and progesterone induce calcium influx and the acrosome reaction in capacitated dog spermatozoa. Mol Reprod Dev 60:491–7
- Cabrita, E., Anel, L., and Herraez, M.P. (2001) Effect of external cryoprotectants as membrane stabilizers on cryopreserved rainbow trout sperm. Theriogenology 56:623–35
- Clapham, D.E., and Garbers, D.L. (2005) International Union of Pharmacology. L. Nomenclature and structure-function relationships of CatSper and two-pore channels. Pharmacol Rev 57:451–4
- Clapham, D.E., Montell, C., Schultz, G., and Julius, D. (2003) International Union of Pharmacology. XLIII. Compendium of voltage-gated ion channels: transient receptor potential channels. Pharmacol Rev 55:591–6
- Coy, P., Gadea, J., Rath, D., and Hunter, R.H. (2009) Differing sperm ability to penetrate the oocyte in vivo and in vitro as revealed using colloidal preparations. Theriogenology 72:1171–9
- Darszon, A., Acevedo, J.J., Galindo, B.E., Hernandez-Gonzalez, E.O., Nishigaki, T., Trevino, C.L., et al. (2006) Sperm channel diversity and functional multiplicity. Reproduction 131:977–88
- Darszon, A., Nishigaki, T., Beltran, C., and Trevino, C.L. (2011) Calcium channels in the development, maturation, and function of spermatozoa. Physiol Rev 91:1305–55
- Darszon, A., Nishigaki, T., Wood, C., Trevino, C.L., Felix, R., and Beltran, C. (2005) Calcium channels and Ca2+ fluctuations in sperm physiology. Int Rev Cytol 243:79–172
- Elhamdani, A., Bossu, J.L., and Feltz, A. (1994) Evolution of the Ca2+ current during dialysis of isolated bovine chromaffin cells: effect of internal calcium. Cell Calcium 16:357–66
- Escoffier, J., Boisseau, S., Serres, C., Chen, C.C., Kim, D., Stamboulian, S., et al. (2007) Expression, localization and functions in acrosome reaction and sperm motility of Ca(V)3.1 and Ca(V)3.2 channels in sperm cells: an evaluation from Ca(V)3.1 and Ca(V)3.2 deficient mice. J Cell Physiol 212:753–63
- Food and Agriculture Organization of the United Nations. (2003) Protein and amino acid requirements in human nutrition: report of a joint FAO/WHO/UNU expert consultation. Author; Geneva
- Fraire-Zamora, J.J., and Gonzalez-Martinez, M.T. (2004) Effect of intracellular pH on depolarization-evoked calcium influx in human sperm. Am J Physiol Cell Physiol 287:C1688–96
- Gomez, M.C., Catt, J.W., Gillan, L., Evans, G., and Maxwell, W.M. (1997) Effect of culture, incubation and acrosome reaction of fresh and frozen-thawed ram spermatozoa for in vitro fertilization and intracytoplasmic sperm injection. Reprod Fertil Dev 9:665–73
- Gonzalez-Martinez, M.T. (2003) Induction of a sodium-dependent depolarization by external calcium removal in human sperm. J Biol Chem 278:36304–10
- Gutman, G.A., Chandy, K.G., Adelman, J.P., Aiyar, J., Bayliss, D.A., Clapham, D.E., et al. (2003) International Union of Pharmacology. XLI. Compendium of voltage-gated ion channels: potassium channels. Pharmacol Rev 55:583–6
- Hagiwara, S., and Kawa, K. (1984) Calcium and potassium currents in spermatogenic cells dissociated from rat seminiferous tubules. J Physiol 356:135–49
- Hasdemir, U. (2007) The role of cell wall organization and active efflux pump systems in multidrug resistance of bacteria. Mikrobiyol Bul 41:309–27
- Hille, B. (1992) Ionic channels of excitable membranes. Sinauer; Sunderland, MA, Chapter 3, 68p
- Hunter, R.H. (2012) Temperature gradients in female reproductive tissues. Reprod Biomed Online 24:377–80
- Hunter, R.H., and Nichol, R. (1986) A preovulatory temperature gradient between the isthmus and ampulla of pig oviducts during the phase of sperm storage. J Reprod Fertil 77:599–606
- Hurwitz, S. (1996) Homeostatic control of plasma calcium concentration. Crit Rev Biochem Mol Biol 31:41–100
- Ingvartsen, K.L. and Moyes, K. (2013) Nutrition, immune function and health of dairy cattle. Animal 7(Suppl 1):112--122
- Jagannathan, S., Publicover, S.J., and Barratt, C.L. (2002) Voltage-operated calcium channels in male germ cells. Reproduction 123:203–15
- Kirchhoff, C., Osterhoff, C., Pera, I., and Schroter, S. (1998) Function of human epididymal proteins in sperm maturation. Andrologia 30:225–32
- Kirichok, Y., Navarro, B., and Clapham, D.E. (2006) Whole-cell patch-clamp measurements of spermatozoa reveal an alkaline-activated Ca2+ channel. Nature 439:737–40
- Knobil, E. and Neill, J.D. (1994) The Physiology of reproduction. Raven Press; New York, 2nd ed, Chapter 1
- Krasznai, Z., Marian, T., Izumi, H., Damjanovich, S., Balkay, L., Tron, L., et al. (2000) Membrane hyperpolarization removes inactivation of Ca2+ channels, leading to Ca2+ influx and subsequent initiation of sperm motility in the common carp. Proc Natl Acad Sci USA 97:2052–7
- Kumar, S., Ying, Y.K., Hong, P., and Maddaiah, V.T. (2000) Potassium increases intracellular calcium simulating progesterone action in human sperm. Arch Androl 44:93–101
- Linares-Hernandez, L., Guzman-Grenfell, A.M., Hicks-Gomez, J.J., and Gonzalez-Martinez, M.T. (1998) Voltage-dependent calcium influx in human sperm assessed by simultaneous optical detection of intracellular calcium and membrane potential. Biochim Biophys Acta 1372:1–12
- Lishko, P.V., Botchkina, I.L., and Kirichok, Y. (2011) Progesterone activates the principal Ca2+ channel of human sperm. Nature 471:387–91
- Marconi, M., Sanchez, R., Ulrich, H., and Romero, F. (2008) Potassium current in mature bovine spermatozoa. Syst Biol Reprod Med 54:231–9
- Martinez-Lopez, P., Santi, C.M., Trevino, C.L., Ocampo-Gutierrez, A.Y., Acevedo, J.J., Alisio, A., et al. (2009) Mouse sperm K+ currents stimulated by pH and cAMP possibly coded by Slo3 channels. Biochem Biophys Res Commun 381:204--209
- Munoz-Garay, C., De la Vega-Beltran, J.L., Delgado, R., Labarca, P., Felix, R., and Darszon, A. (2001) Inwardly rectifying K(+) channels in spermatogenic cells: functional expression and implication in sperm capacitation. Dev Biol 234:261–74
- Navarrete, P., Martinez-Torres, A., Gutierrez, R.S., Mejia, F.R., and Parodi, J. (2010) Venom of the Chilean Latrodectus mactans alters bovine spermatozoa calcium and function by blocking the TEA-sensitive K(+) current. Syst Biol Reprod Med 56:303–10
- Neher, E., and Sakmann, B. (1976) Noise analysis of drug induced voltage clamp currents in denervated frog muscle fibres. J Physiol 258:705–29
- Neher, E., Sakmann, B., and Steinbach, J.H. (1978) The extracellular patch clamp: a method for resolving currents through individual open channels in biological membranes. Pflugers Arch 375:219–28
- Neri-Vidaurri Pdel, C., Torres-Flores, V., and Gonzalez-Martinez, M.T. (2006) A remarkable increase in the pHi sensitivity of voltage-dependent calcium channels occurs in human sperm incubated in capacitating conditions. Biochem Biophys Res Commun 343:105–9
- Nikpoor, P., Mowla, S.J., Movahedin, M., Ziaee, S.A., and Tiraihi, T. (2004) CatSper gene expression in postnatal development of mouse testis and in subfertile men with deficient sperm motility. Hum Reprod 19:124–8
- Oficina de Estudios y Politicas Agrarias. (2011) Existencia de cerdos en criaderos por tipo, según semestre. Available at: http://www.odepa.cl/articulos/MostrarDetalle.action;jsessionid=0144A1D0090E0066E0DB26825978CE0C?idcla=12&idn=4120 [last accessed 6 Dec 2013]
- Parodi, J., Navarrete, P., Marconi, M., Gutierrez, R.S., Martinez-Torres, A., and Mejias, F.R. (2010) Tetraethylammonium-sensitive K(+) current in the bovine spermatozoa and its blocking by the venom of the Chilean Latrodectus mactans. Syst Biol Reprod Med 56:37–43
- Patrat, C., Auer, J., Fauque, P., Leandri, R.L., Jouannet, P., and Serres, C. (2006) Zona pellucida from fertilised human oocytes induces a voltage-dependent calcium influx and the acrosome reaction in spermatozoa, but cannot be penetrated by sperm. BMC Dev Biol 6:59
- Perry, R.L., Barratt, C.L., Warren, M.A., and Cooke, I.D. (1997) Response of human spermatozoa to an internal calcium ATPase inhibitor, 2,5-di(tert-butyl) hydroquinone. J Exp Zool 279:284–90
- Pounds, J.G. (1984) Effect of lead intoxication on calcium homeostasis and calcium-mediated cell function: a review. Neurotoxicology 5:295–331
- Qi, H., Moran, M.M., Navarro, B., Chong, J.A., Krapivinsky, G., Krapivinsky, L., et al. (2007) All four CatSper ion channel proteins are required for male fertility and sperm cell hyperactivated motility. Proc Natl Acad Sci USA 104:1219–23
- Quill, T.A., Ren, D., Clapham, D.E., and Garbers, D.L. (2001) A voltage-gated ion channel expressed specifically in spermatozoa. Proc Natl Acad Sci USA 98:12527–31
- Quill, T.A., Sugden, S.A., Rossi, K.L., Doolittle, L.K., Hammer, R.E., and Garbers, D.L. (2003) Hyperactivated sperm motility driven by CatSper2 is required for fertilization. Proc Natl Acad Sci USA 100:14869–74
- Ren, D., Navarro, B., Perez, G., Jackson, A.C., Hsu, S., Shi, Q., et al. (2001) A sperm ion channel required for sperm motility and male fertility. Nature 413:603–9
- Rossato, M., Di Virgilio, F., Rizzuto, R., Galeazzi, C., and Foresta, C. (2001) Intracellular calcium store depletion and acrosome reaction in human spermatozoa: role of calcium and plasma membrane potential. Mol Hum Reprod 7:119–28
- Sagare-Patil, V., Vernekar, M., Galvankar, M., and Modi, D. (2013) Progesterone utilizes the PI3K-AKT pathway in human spermatozoa to regulate motility and hyperactivation but not acrosome reaction. Mol Cell Endocrinol 374:82–91
- Shi, Q.X., and Roldan, E.R. (1995) Evidence that a GABAA-like receptor is involved in progesterone-induced acrosomal exocytosis in mouse spermatozoa. Biol Reprod 52:373–81
- Stewart, A.F. (1985) Calcium metabolism without anguish. Understanding the body's homeostatic ‘black box’. Postgrad Med 77:283–91, 294
- Stokke, B.T., Mikkelsen, A., and Elgsaeter, A. (1985) Human erythrocyte spectrin dimer intrinsic viscosity: temperature dependence and implications for the molecular basis of the erythrocyte membrane free energy. Biochim Biophys Acta 816:102–10
- Strunker, T., Goodwin, N., Brenker, C., Kashikar, N.D., Weyand, I., Seifert, R., et al. (2011) The CatSper channel mediates progesterone-induced Ca2+ influx in human sperm. Nature 471:382–6
- Thomson, M.F., and Wishart, G.J. (1991) Temperature-mediated regulation of calcium flux and motility in fowl spermatozoa. J Reprod Fertil 93:385–91
- Trifaro, J., Rose, S.D., Lejen, T., and Elzagallaai, A. (2000) Two pathways control chromaffin cell cortical F-actin dynamics during exocytosis. Biochimie 82:339–52
- Turner, K.O., and Meizel, S. (1995) Progesterone-mediated efflux of cytosolic chloride during the human sperm acrosome reaction. Biochem Biophys Res Commun 213:774–80
- Wellman, G.C., Cartin, L., Eckman, D.M., Stevenson, A.S., Saundry, C.M., Lederer, W.J., et al. (2001) Membrane depolarization, elevated Ca(2+) entry, and gene expression in cerebral arteries of hypertensive rats. Am J Physiol Heart Circ Physiol 281:H2559–67
- Wennemuth, G., Carlson, A.E., Harper, A.J., and Babcock, D.F. (2003) Bicarbonate actions on flagellar and Ca2+ -channel responses: initial events in sperm activation. Development 130:1317–26
- Wennemuth, G., Westenbroek, R.E., Xu, T., Hille, B., and Babcock, D.F. (2000) CaV2.2 and CaV2.3 (N- and R-type) Ca2+ channels in depolarization-evoked entry of Ca2+ into mouse sperm. J Biol Chem 275:21210–7
- Xia, J., Reigada, D., Mitchell, C.H., and Ren, D. (2007) CATSPER channel-mediated Ca2+ entry into mouse sperm triggers a tail-to-head propagation. Biol Reprod 77:551–9
- Zeng, Y., Clark, E.N., and Florman, H.M. (1995) Sperm membrane potential: hyperpolarization during capacitation regulates zona pellucida-dependent acrosomal secretion. Dev Biol 171:554–63
- Yanagimachi, R. (2011) Mammalian sperm acrosome reaction: where does it begin before fertilization? Biol Reprod 85:4--5
- Zeng, Y., Oberdorf, J.A., and Florman, H.M. (1996) pH regulation in mouse sperm: identification of Na(+)-, Cl(−)-, and HCO3(−)-dependent and arylaminobenzoate-dependent regulatory mechanisms and characterization of their roles in sperm capacitation. Dev Biol 173:510–20
- Zhong, C.L., Xin, X.H., and Shi, Q.X. (1993) Inhibition of spermine on calcium influx during capacitation of guinea pig spermatozoa in vitro. Zhongguo Yao Li Xue Bao 14:141–4