Abstract
Several studies reported that chronic alcohol consumption alters the intestinal mucosa barrier, and subsequent entrance of endotoxins into the bloodstream. In many tissues endotoxin exposure causes the expression of calprotectin (CP) and the receptor for advanced glycation -end products (RAGE). In this study we investigated whether chronic alcohol administration causes expression of CP and RAGE in mouse testis. The distribution of free and loosely bound Zn2+ (FLB-Zn2+) in the testicular tissues was also evaluated. Alcohol-induced testicular damage was documented by measuring testosterone blood levels and by light and electron microscope studies. Twenty mice were treated daily for three weeks with 3.0 g/kg of a 25% solution of alcohol. Ten mice were treated in the same period of time with a solution of maltose dextrins, isocaloric to alcohol. Twenty untreated mice were used as controls. Alcohol treated mice showed diffuse expression of CP and RAGE in the interstitial cells. RAGE was found also in the basal compartment of the seminiferous tubules. Depletion of FLB-Zn2+ was observed in the adluminal compartment of the seminiferous tubules. Expression of CP and RAGE was not found in control mice and maltose dextrin treated mice. Our results indicated novel mechanisms by which alcohol acts in testis. Indeed, CP and RAGE may cause the generation of oxidants and inflammatory mediators, with negative impact on testicular functions. Depletion of FLB-Zn2+ may contribute to the dysregulation of spermatogenesis.
Introduction
Numerous studies have documented that chronic alcohol consumption causes testis atrophy of the seminiferous tubules, morphological alterations of the Leydig cells (LC), and impaired testosterone production [Cicero et al. Citation1980; Gavaler et al. Citation1983; Giannessi et al. Citation2008; Quintans et al. Citation2005; Shi et al. Citation1998; Zhu et al. Citation2000]. It was also reported that alcohol consumption alters semen parameters. Reduction of spermatozoa number, high percentage of morphologically altered spermatozoa, and decrease in seminal volume were the more frequently found abnormalities [Condorelli et al. Citation2014]. Zinc depletion was also described [Ahmed and Russel Citation1982]. Although alcohol interferes with the hypothalamic-pituitary axis [Shi et al. Citation1998], several of its noxious effects take place in the testis. The transformation of alcohol to acetaldehyde in the LC is believed to be critical in the development of testicular injury [Cicero et al. Citation1980; Quintans et al. Citation2005]. A consequence of this reaction is the elevation of the NADH/NAD+ ratio leading to enhanced production of reactive oxygen species (ROS) [Quintans et al. Citation2005]. In addition, acetaldehyde inhibits the conversion of androstenedione to testosterone [Cicero et al. Citation1980], and causes depletion of mitochondrial glutathione [Quintans et al. Citation2005]. Besides these effects, another mechanism is involved in testicular damage. Studies reported that chronic alcohol consumption alters the intestinal mucosa barrier in mice [Uesugi et al. Citation2002], rats [Tamai et al. Citation2002], and humans [Rao et al. Citation2004]. As a consequence, endotoxins, produced by gram-negative bacteria, may have access to the bloodstream becoming a contributory factor of alcohol-induced damage observed in testis [Allen et al. Citation2004, Hales Citation2002; Reddy et al. Citation2006]. With regard to this, it was observed that the bacterial endotoxin lipopolysaccharide (LPS) activates testicular macrophages (TM), the resident population of macrophages in testis [Hales Citation2002], to produce ROS, cytokines, and chemokines. The result was the alteration of spermatogenesis and the inhibition of testosterone production [Hales Citation2002]. LPS is also known to induce the expression of calprotectin (CP). This was documented in several cell types, including neutrophils [Kido et al. Citation2004], monocytes [Kido et al. Citation2005], bronchial epithelial cells [Henke et al. Citation2006], and cardiomyocytes [Boyd et al. Citation2008]. CP is a molecular complex formed by two proteins of the S100 family, S100A8 and S100A9 [Ehrchen et al. Citation2009; Gebhardt et al. Citation2006; Striz and Trebichavsky Citation2004]. Once released by producing cells in the extracellular compartment, CP exerts numerous functions, mostly associated with inflammatory processes [Ehrchen et al. Citation2009; Gebhardt et al. Citation2006; Striz and Trebichavsky Citation2004]. Mice lacking CP were found to be protected from endotoxin-induced lethal shock, suggesting that significant effects of endotoxins are mediated by CP [Vogl et al. Citation2007]. The endotoxins also have the potential to cause the receptor expression for advanced glycation end products (RAGE), a multi-ligand receptor of the immunoglobulin family, which is found on the surface of a variety of cell types [Bierhaus et al. Citation2005; Ramasamy et al. Citation2009; Yan et al. Citation2009]. Recruitment of RAGE activates various cellular processes including inflammation and apoptosis [Bierhaus et al. Citation2005; Ramasamy et al. Citation2009; Yan et al. Citation2009]. There is evidence that CP is a RAGE ligand and that CP and RAGE are frequently co-expressed in pathological tissues [Ehlermann et al. Citation2006; Gebhardt et al. Citation2006; Ghavami et al. Citation2008; Ichikawa et al. Citation2011; Leclerc et al. Citation2009; Volz et al. Citation2012]. The aim of our study was to determine whether CP and RAGE are expressed in testis of male mice subjected to chronic alcohol administration. Alcohol-induced damage was documented by measuring serum testosterone levels and by light and electron microscopy of testicular tissues. The distribution of free and loosely bound Zn2+ (FLB-Zn2+) was also assessed by means of a fluorescent dye.
Results
Microscopy analysis
As determined by light microscopy, alcohol treatment severely altered the morphology of mice testes. Compared with the seminiferous tubules of control mice (), those of alcohol-treated mice () showed severe disarrangement of the seminiferous epithelium, consisting in reduced thickness and diffuse vacuolization. Both in control () and alcohol-treated mice (), the interstitial spaces present in each section showed from one to three TM. Similarly, as determined by electron microscopy, as compared to the control (), the LC of alcohol-treated mice showed evident alterations (). Some LC were characterized by rarefaction of the cytoplasm. Other LC were degenerated. Afflux of leukocytes was not observed.
Figure 1. Light microscopy. Trypan blue treated mice. (A) Control mouse. Some seminiferous tubules with normal appearance are shown. The arrows point to two testicular macrophages. (B) Alcohol treated mouse. The morphology of the seminiferous tubules is severely altered. The tubules show reduced thickness and vacuolization (arrowheads). The arrows point to two testicular macrophages. Scale bars: 50 µm.
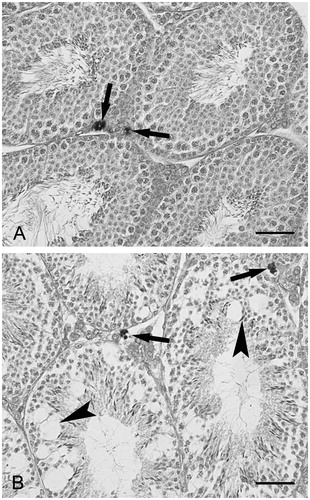
Figure 2. Ultrastructure of interstitial spaces. (A) Control mouse. Several Leydig cells and one testicular macrophage (M) are shown; scale bar: 1.3 µm. (B) Alcohol treated mouse. Several Leydig cells and one testicular macrophage (M) are shown. Two Leydig cell show rarefaction of the cytoplasm (arrows). One Leydig is degenerated (arrowhead). Leukocytes are not present; scale bar: 1.3 µm.
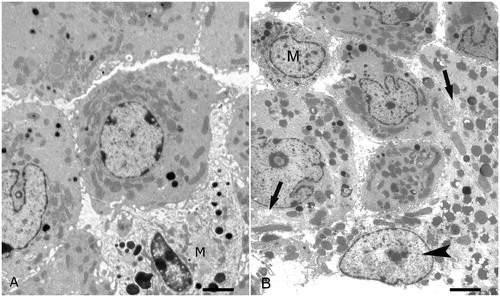
Immunohistochemistry
As determined by CP immunohistochemistry, most of the cells present in the interstitial spaces were labeled (). The reaction was not observed in the seminiferous tubules (). In control mice () and in maltose dextrin treated mice, staining for CP was not found. No labeling was observed when the primary antibody was omitted. In comparison, as determined by RAGE immunohistochemistry most of the cells present in the interstitial spaces were labeled (). Moreover, the reaction was found in the basal compartment of the seminiferous tubules (). At high magnification () the labeled cells were observed to form a single layer near the basement membrane of the seminiferous tubules. In control mice () and in maltose dextrin treated mice, labeling for RAGE was not found. No labeling was observed when the primary antibody was omitted.
Figure 3. Calprotectin immunohistochemistry. (A) Alcohol treated mouse. Diffuse labeling is present in the interstitial cells (arrows). No labeling is found in the seminiferous tubules; scale bar: 60 µm. (B) Control mouse. Labeling is lacking, both in the seminiferous tubules and the interstitial cells; scale bar: 60 µm.
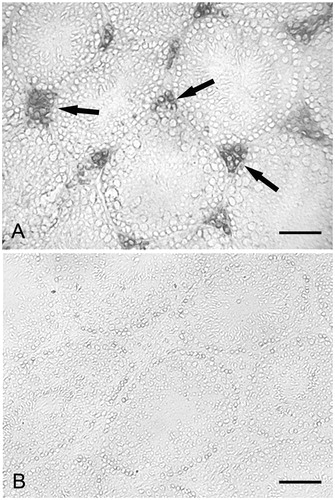
Figure 4. RAGE immunohistochemistry. (A) Small magnification picture. Diffuse labeling is observed in the interstitial cells (arrows). Labeling is also present and basal compartment of the seminiferous tubules (arrowheads); scale bar: 60 µm. (B) Larger magnification of an interstitial space showing a diffuse labeling; scale bar: 25 µm. (C) Larger magnification of seminiferous tubules showing labeled cells in the basal compartment (arrows). No labeling is present in the adluminal compartment; scale bar: 35 µm. (D) Control mouse. Labeling is lacking, both in the seminiferous tubules and interstitial cells; scale bar: 60 µm.
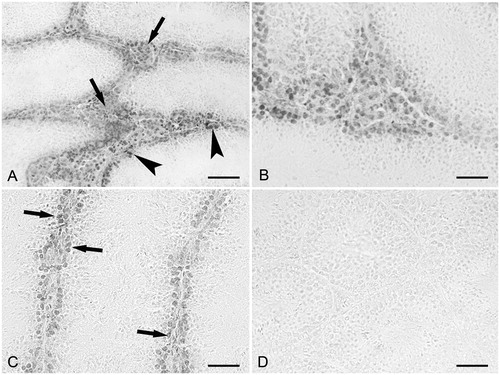
Free and bound zinc was determined by fluorescence staining. In control mice, intense fluorescence () was found either in basal and ad-luminal compartments of the seminiferous tubules (). Mature spermatids showed intense fluorescence (). Faint fluorescence was found in the interstitial cells (). In comparison with the controls, alcohol treated mice showed significant fluorescence depletion (). The fluorescence was circumscribed to basal compartment of the seminiferous tubules (). This was observed in all the seminiferous tubules, regardless of the stage of the seminiferous epithelium (). Faint fluorescence was found in the interstitial cells (). The distribution of fluorescence in maltose dextrin treated mice was similar to that found in control mice. Interestingly, the mean value of the testosterone plasma levels found in the alcohol-treated mice was significantly lower (1.6 ± 0.6 ng/mL, p < 0.01) than that in maltose dextrin treated mice (6.4 ± 1.9 ng/mL) and control mice (6.8 ± 2.1 ng/mL) (). The mean value of the testosterone plasma levels of maltose dextrin treated mice did not significantly differ from those of control mice ().
Figure 5. Fluorescent micrographs of mouse testes after staining of free and loosely bound Zn2+ with Zinpyr-1. (A) Control mouse. Intense fluorescence is observed in the seminiferous tubules. Faint fluorescence is present in the interstitial cells (arrows); scale bar: 35 µm. (B) Control mouse. Greater magnification of a seminiferous tubule in transverse section. The fluorescence is evident in the basal (arrows) and adluminal (arrowheads) compartments; scale bar: 18 µm. (C) Control mouse. Late spermatids showing intense fluorescence; scale bar: 15 µm. (D) Alcohol treated mouse. The picture shows some seminiferous tubules characterized by different stages of the seminiferous epithelium. The fluorescence is concentrated in the basal compartment of the seminiferous tubules (arrowhead). Faint fluorescence is present the interstitial cells (arrows); scale bar: 35 µm.
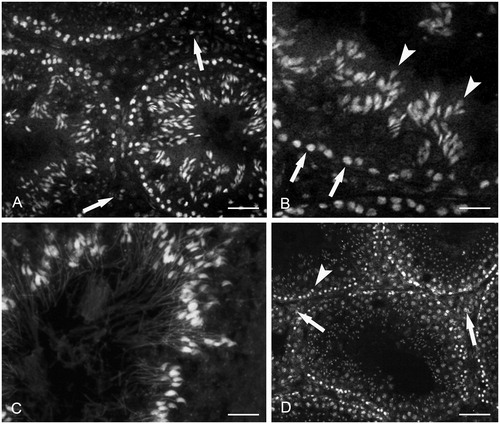
Figure 6. Testosterone plasma levels. Testosterone plasma levels of alcohol-treated mice (black) are significantly decreased when compared with those of maltose dextrins treated mice (gray) and control mice (white). Each bar represents the mean ± SD of fifteen mice. **p < 0.01 versus maltose dextrins treated and control mice.
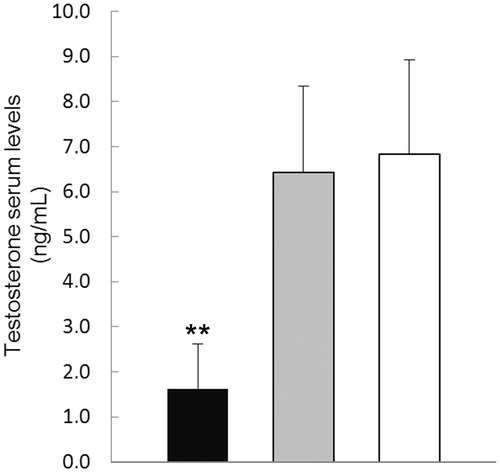
Discussion
The present study confirmed the results of previous studies [Cicero et al. Citation1980; Gavaler et al. Citation1983; Giannessi et al. Citation2008; Quintans et al. Citation2005; Shi et al. Citation1998; Zhu et al. Citation2000] that chronic alcohol administration is followed in testis by damage to the LC and the seminiferous tubules (, ) and reduced serum testosterone levels (). The ultrastructural study did not reveal the presence of leukocytes in the interstitial spaces (). Labeling with trypan blue did not reveal substantial variations in the number of macrophages in the interstitial spaces (). We demonstrated for the first time that chronic alcohol administration is associated with the expression of CP and RAGE in the interstitial cells ( and ) and with depletion of FLB-Zn2+ in seminiferous tubules (). RAGE was found also in the basal compartment of the seminiferous tubules (). Testicular tissues of control mice () and maltose-dextrin treated mice did not show any immunohistochemical staining. CP was originally described as a protein constitutively expressed in the cytosol of neutrophils, mononuclear phagocytes, and keratinocytes. Subsequent studies recognized CP in several other cell types of inflamed tissues and in tumor cells [Ehrchen et al. Citation2009; Gebhardt et al. Citation2006; Striz and Trebichavsky Citation2004; Volz et al. Citation2012]. Elevated CP levels in tissues and body fluids are considered a hallmark of pathological conditions associated with inflammation (e.g., rheumatoid arthritis, systemic lupus erythematosus, chronic inflammatory bowel diseases, cardiac diseases) [Ehrchen et al. Citation2009; Gebhardt et al. Citation2006; Striz and Trebichavsky Citation2004; Volz et al. Citation2012]. Once released by producing cells in extracellular spaces, CP triggers a plethora of molecular events. However, several of these are mediated by activation of toll-like receptors (TLR) and RAGE. TLR are a class of proteins that play crucial roles in the innate immunity [Kawai and Akira Citation2006]. With regard to this, it was recently observed that mouse LC express TLR4 and TLR3 [Shang et al. Citation2011]. Activation of these receptors was shown to initiate a response of the LC characterized by NF-kB up-regulation and consequent induction of various inflammatory mediators, including IL-1β, IL-6, and TNF-α. Suppression of testosterone biosynthesis was also observed [Shang et al. Citation2011].
These results suggest that CP could affect testicular functions through TLR signaling. However, this role of CP needs to be clarified in further studies. RAGE is absent, or it is expressed at low levels, in most normal tissues. Consistent with this, control mice did not show any immunohistochemical staining for RAGE, either in the seminiferous tubules or the interstitial cells (). High levels of RAGE were previously observed in pathological conditions, such as vascular diseases, chronic inflammation, Alzheimer’s disease, diabetes, and cancer [Clynes et al. Citation2007; Gebhardt et al. Citation2008; Ichikawa et al. Citation2011; Ramasamy et al. Citation2011; Volz et al. Citation2012; Yan et al. Citation2009]. RAGE expression was also documented in the seminiferous tubules, interstitial cells [Mallidis et al. Citation2007], and spermatozoa [Karimi et al. Citation2012] of diabetic patients. Engagement of RAGE triggers several signaling molecules, including the transcription factor NF-kB, MAP kinases, and adhesion molecules, that stimulate the inflammatory responses and increase the oxidative stress [Bierhaus et al. Citation2005; Ramasamy et al. Citation2009; Yan et al. Citation2009], with possible alteration of the testicular functions. There is evidence that CP is a RAGE ligand [Ehlermann et al. Citation2006; Gebhardt et al. Citation2006; Ghavami et al. Citation2008; Ichikawa et al. Citation2011; Leclerc et al. Citation2009; Volz et al. Citation2012], and that RAGE is activated and up-regulated by the presence of its own ligands [Clynes et al. Citation2007; Gebhardt et al. Citation2008; Ramasamy et al. Citation2011; Schmidt et al. Citation2009; Yan et al. Citation2009]. Therefore, contemporary expression of CP and RAGE in the testicular tissues likely generates a positive feed back loop resulting in high inflammation levels. Upregulation of RAGE, as a consequence of RAGE-ligand interaction, was associated with various diseases, including diabetes, vascular diseases, neurodegenerative diseases, and cancer [Clynes et al. Citation2007; Gebhardt et al. Citation2008; Ichikawa et al. Citation2011; Ramasamy et al. Citation2011; Schmidt et al. Citation2009; Volz et al. Citation2012; Yan et al. Citation2009]. Taken together, these observations allow one to suppose that the expression of CP and RAGE play a significant role in the alterations of the semen parameters occurring in chronic alcoholism [Condorelli et al. Citation2014].
We observed that depletion of FLB-Zn2+ in alcohol-treated mice occurs at specific stages of the spermatogenetic cycle. In fact, in control mice FLB-Zn2+ was widely spread in the basal and adluminal compartments of the seminiferous tubules, while in alcohol-treated mice FLB-Zn2+ was found only in the basal compartment. This appeared as a general feature, regardless of the stage of the seminiferous epithelium (). Zn2+ is involved in many aspects of germ cell physiology. It is known to promote sperm maturation, to protect germ cells from oxidative stress, and to increase sperm mobility [Bedwal and Bahuguna Citation1994; Hamdi et al. Citation1997; Oteiza et al. Citation1995]. It cannot be without consideration that depletion of FLB-Zn2+ is a consequence of the depressed spermatogenetic activity induced by alcohol. However, it was shown in previous studies that CP is a strong Zn2+ chelator. Sequestration of extracellular Zn2+ by CP may cause apoptosis in bacteria and tumor cells [Ehrchen et al. Citation2009; Gebhardt et al. Citation2006; Striz and Trebichavsky Citation2004]. Moreover, massive expression of CP may induce Zn2+ dysregulation and apoptosis in cells which are normal tissue components [Ehrchen et al. Citation2009; Gebhardt et al. Citation2006; Striz and Trebichavsky Citation2004]. An important consequence of CP overproduction is the inhibition of the Zn2+-dependent enzymes matrix metalloproteinases (MMPs) [Isaksen and Fagerhol Citation2001]. In testis, the activity of MMPs is critical to regulate the metabolism of the extracellular matrix that needs continuous remodeling during germ cells migration from the basal to the top layers of the seminiferous epithelium [Siu and Chen Citation2004]. Taken together, these observations support the hypothesis that expression of CP may be associated with the alteration of Zn2+-dependent testicular functions.
In summary, we showed that chronic alcohol administration is followed in mouse testis by expression of CP and RAGE. As a consequence, oxidant and inflammatory mediators may accumulate in testicular tissues, leading to inhibition of the spermatogenesis and the steroidogenesis. Contemporary expression of CP and RAGE likely up-regulates RAGE signaling pathways. Some effects of CP could be mediated by activation of TLR in the LC. We propose that FLB-Zn2+ depletion in the seminiferous tubules could depend, at least in part, from the Zn2+ binding property of CP. Up to now, many studies described the damage caused by alcohol in testis, but the mechanisms behind it were not fully elucidated. Results of the present study provide insight to better understand these mechanisms and, possibly, to establish novel therapeutic strategies against the alcohol-induced alterations of testicular functions.
Materials and methods
Animals
Fifty eight week old Swiss mice (Harlan, Italy) were used in this study. The animals were housed in metal wire cages at 22°C, under a 12:12 h light-dark photoperiod and allowed to acclimatize for a week before the experiment. The mice were provided with free access to standard laboratory chow and water ad libitum, and were randomly assigned to the various experimental groups. The mice were sacrificed in agreement with “Guide for Care and Use of Laboratory Animals” published by National Institutes of Health, 1996 protocol for care and use of the experimental animals, and the protocol was approved by our local Ethical Committee.
Experimental protocol
The experimental period was 21 d. Three experimental groups of mice were utilized: (1) twenty untreated mice as controls, (2) twenty mice treated daily with a 3.0 g/Kg 25% (v/v) solution of ethanol, and (3) ten mice treated daily with a solution of PBS (pH 7.2), containing maltose dextrins which were isocalorically substituted for ethanol. All the substances were administered at 12 p.m by intragastric intubation.
Light microscopy
Twenty-four hours before sacrificing the animals, 10 alcohol-treated mice and 10 untreated mice were injected subcutaneously with the vital dye trypan blue (0.5% in physiological solution). The goal was to distinguish the TM from the LC; the TM, unlike the LC, has the capacity to take on this dye [Miller et al. Citation1983]. The testes were fixed with Bouin fluid and embedded in paraffin. Serial sections were stained with eosin and examined with a light microscope.
Electron microscopy
Small fragments, about 2 mm wide, were collected from those testes of untreated and alcohol-treated mice who were not given the trypan blue. The fragments were immersed in a fixative made up of 4% paraformaldehyde and 1% glutaraldehyde in 0.1 phosphate buffer at 4°C. After washing in buffer, the specimens were post-fixed in 1.3% osmium tetroxide in phosphate buffer for 1 h, dehydrated, and embedded in Epon, following the usual procedures. Ultrathin sections about 100 nm thick were serially cut and every third one was mounted on a single-hole formvar-coated grid. The sections were double-stained with saturated uranyl acetate in 50% alcohol and with lead citrate, and then observed with a Jeol 100 SX electron microscope (JEOL USA, Inc., Peabody, MA, USA).
Immunohistochemistry
The testes from 10 alcohol treated mice, and from 10 control mice, not given with trypan blue, were rapidly removed and snap-frozen in liquid nitrogen. Frozen sections (15 µm thick) were cut at cryostat and mounted on slides. The experiments were carried out in 2 d cycles. During the first day the samples were fixed in 4% paraformaldehyde for 30 min at room temperature. Antigen retrieval was performed by treatment with trypsin (1 mg/ml) (Sigma, St. Louis, MO, USA) for 10 min at 37°C. Then, the sections were incubated in cold methanol and 0.3% hydrogen peroxide for 30 min at 4°C, in order to inhibit the activity of endogenous peroxidases, and successively treated with 10% normal swine serum (NSS) (Vector Labs., Burlingame, CA, USA) for 1 hr at room temperature. For CP immunohistochemistry, the sections were incubated overnight at 4°C with a mouse monoclonal anti-CP antibody (Biomeda, CA, USA) diluted 1:50 in PBS containing 1% bovine serum albumin (BSA, Boehringer Mannheim, Germany) and 5% NSS. For RAGE immunohistochemistry, other sections were incubated overnight at 4°C with a goat polyclonal anti-RAGE antibody (Abcam, Cambridge, UK), diluted 1:400 in PBS containing 1% BSA and 5% NSS. On the second day, the secondary biotinylated polyclonal swine antibody (Dako, Denmark, A/S), diluted 1:100 in PBS containing 1.5% NSS, was added to the sections for 30 min at room temperature. A peroxidase 3,3’ – diaminobenzidine system (Dako) was used for the staining procedure. Finally, the sections were mounted in glycerol. Negative control sections were obtained by omitting the primary antibody.
Fluorescence staining of FLB- Zn2+
Cryostat sections (15 µm thick) from control mice and alcohol-treated mice were stained with 20 µM Zinpyr-1 (Sigma) in physiological medium (mM: 147 NaCl, 4.0 KCl, 3 CaCl2, 0.9 MgCl2, 11 HEPES, 10 glucose, pH 7.4) for 1-2 min, washed in the same medium, and visualized with a conventional epifluorescence research microscope.
Testosterone measurement
Blood samples from control mice and alcohol-treated mice were obtained by cardiac puncture without hemolysis, and they were utilized for the measurement of testosterone plasma levels by radioimmunoassay. All samples were run in duplicate. The minimum level of detection was 0.08 ng/mL. All samples were analyzed in the same assay and the intra-assay coefficient of variation was 8%.
Statistical analysis
Data concerning testosterone plasma levels were analyzed using 1-way analysis of variance (ANOVA), followed by post hoc Fisher’s least significant difference test and p ≤ 0.01 was considered statistically significant.
Abbreviations | ||
CP | = | calprotectin |
FLB- Zn2+ | = | free and loosely bound Zn2+ |
LC | = | Leydig cells |
LPS | = | endotoxin lipopolysaccharide |
MMPs | = | matrix metalloproteinases |
RAGE | = | receptor for advanced glycation end products |
ROS | = | reactive oxygen species |
TLR | = | toll-like receptors |
TM | = | testicular macrophages |
Declaration of interest
Grant support was received from the Ministry of University and Research, Italy. The authors report no conflicts of interest. The authors alone are responsible for the content and writing of the paper.
Author contributions
Conceived and designed the experiments: FG; Performed the experiments: MCS, MAG; Analyzed the data: FG, MCS, MAG, FF, GM, RR; Contributed reagents/materials/analysis tools: FG; Wrote the manuscript: FG, MCS, MAG, FF, RR.
References
- Ahmed, S.B. and Russell, R.M. (1982) The effect of ethanol feeding on zinc balance and tissue zinc levels in rats maintained on zinc-deficient diets. J Lab Clin Med 100:211–217
- Allen, J.A., Diemer, T., Janus, P., Hales, K.H. and Hales, D.B. (2004) Bacterial endotoxin lipopolysaccharide and reactive oxygen species inhibit Leydig cell steroidogenesis via perturbation of mitochondria. Endocrine 25:265–275
- Bedwal, R.S. and Bahuguna, A. (1994) Zinc, copper and selenium in reproduction. Experientia 50:626–640
- Bierhaus, A., Humpert, P.M., Morcos, M., Wendt, T., Chavakis, T., Arnold, B., et al. (2005) Understanding RAGE, the receptor for advanced glycation end products. J Mol Med 83:876–886
- Boyd, J.H., Kan, B., Roberts, H., Wang, Y. and Walley, K.R. (2008) S100A8 and S100A9 mediate endotoxin-induced cardiomyocyte dysfunction via the receptor for advanced glycation end products. Circ Res 102:1239–1246
- Cicero, T.J., Bell, R.D., Meyer, E.R. and Badger, T.M. (1980) Ethanol and acetaldehyde directly inhibit testicular steroidogenesis. J Pharmacol Exp Ther 213:228–233
- Clynes, R., Moser, B., Yan, S.F., Ramasamy, R., Herold, K. and Schmidt, A.M. (2007) Receptor for AGE (RAGE): weaving tangled webs within the inflammatory response. Curr Mol Med 7:743–751
- Condorelli, R.A., Calogero, A.E., Vicari, E. and La Vignera, S. (2014) Chronic consumption of alcohol and sperm parameters: our experience and the main evidences. Andrologia Apr 27. doi: 10.1111/and.12284. [Epub ahead of print] PubMed PMID: 24766499
- Ehlermann, P., Eggers, H., Bierhaus, A., Most, P., Weichenhan, D., Greten, J., et al. (2006) Increased proinflammatory endothelial response to S100A8/A9 after preactivation through advanced glication end products. Cardiovasc Diabetol 5:6
- Ehrchen, J.M., Sunderkötter, C., Foell, D., Vogl, T. and Roth, J. (2009) The endogenous Toll-like receptor 4 agonist S100A8/S100A9 (calprotectin) as innate amplifier of infection, autoimmunity, and cancer. J Leukoc Biol 86:557–566
- Gavaler, J.S., Perez, H.A., Estes, L. and Van Thiel, D.H. (1983) Morphologic alterations of rat Leydig cells induced by ethanol. Pharmacol Biochem Behav 18:341–347
- Gebhardt, C., Németh, J., Angel, P. and Hess, J. (2006) S100A8 and S100A9 in inflammation and cancer. Biochem Pharmacol 72:1622–1631
- Gebhardt, C., Riehl, A., Durchdewald, M., Németh J., Fürstenberger G., Müller- Decker, M., et al. (2008) RAGE signaling sustains inflammation and promotes tumor development. J Exp Med 205:275–285
- Ghavami, S., Rashedi, I., Dattilo, B.M., Eshraghi, M., Chazin, W.J., Hashemi, M., et al. (2008) S100A8/A9 at low concentration promotes tumor cell growth via RAGE ligation and MAP kinase-dependent pathway. J Leukoc Biol 83:1484–1492
- Giannessi, F., Giambelluca, M.A., Grasso, L., Scavuzzo, M.C. and Ruffoli, R. (2008) Curcumin protects Leydig cells of mice from damage induced by chronic alcohol administration. Med Sci Monit 14:237–242
- Hales, D.B. (2002) Testicular macrophage modulation of Leydig cell steroidogenesis. J Reprod Immunol 57:3–18
- Hamdi, S.A., Nassif, O.I. and Ardawi, M.S. (1997) Effect of marginal or severe dietary zinc deficiency on testicular development and functions of the rat. Arch Androl 38:243–253
- Henke, M.O., Renner, A., Rubin, B.K., Gyves, J.I., Lorenz, E. and Koo, J.S. (2006) Up-regulation of S100A8 and S100A9 protein in bronchial epithelial cells by lipopolysaccharide. Exp Lung Res 32:331–347
- Ichikawa, M., Williams, R., Wang, L., Vogl, T. and Srikrishna, G. (2011) S100A8/A9 activate key genes and pathways in colon tumor progression. Mol Cancer Res 9:133–148
- Isaksen, B. and Fagerhol, M.K. (2001) Calprotectin inhibits matrix metalloproteinases by sequestration of zinc. Mol Pathol 54:289–292
- Karimi, J., Goodarzi, M.T., Tavilani, H., Khodadadi, I. and Amiri, I. (2012) Increased receptor for advanced glycation end products in spermatozoa of diabetic men and its association with sperm nuclear DNA fragmentation. Andrologia 44:280–286
- Kawai, T. and Akira, S. (2006) TLR signaling. Cell Death Differ 13:816–825
- Kido, J., Kido, R., Suryono, Kataoka, M., Fagerhol, M.K. and Nagata, T. (2004) Induction of calprotectin release by porphyromonas gingivalis lipopolysaccharide in human neutrophils. Oral Microbiol Immunol 19:182–187
- Kido, J., Hayashi, N., Kataoka, M. and Nagata, T. (2005) Calprotectin expression in human monocytes: induction by porphyromonas gingivalis lipopolysaccharide, tumor necrosis factor-alpha, and interleukin-1beta. J Periodontol 76:437–442
- Leclerc, E., Fritz, G., Vetter, S.W. and Heizmann, C.W. (2009) Binding of S100 proteins to RAGE: An update. Biochim Biophys Acta 1793:993–1007
- Mallidis, C., Agbaje, I., Rogers, D., Glenn, J., McCullough, S., Atkinson, A.B., et al. (2007) Distribution of the receptor for advanced glycation end products in the human male reproductive tract: prevalence in men with diabetes mellitus. Hum Reprod 22:2169–2177
- Miller, S.C., Bowman, B.M. and Rowland, H.G. (1983) Structure, cytochemistry, endocytic activity, and immunoglobulin (Fc) receptors of rat testicular interstitial–tissue macrophages. Am J Anat 168:1–13
- Oteiza, P.I., Olin, K.L., Fraga, C.G. and Keen, C.L. (1995) Zinc deficiency causes oxidative damage to proteins, lipids and DNA in rat testes. J Nutr 125:823–829
- Quintans, L.N., Castro, G.D. and Castro, J. (2005) Oxidation of ethanol to acetaldehyde and free radicals by rat testicular microsomes. Arch Toxicol 79:25–30
- Ramasamy, R., Yan, S.F. and Schmidt, A.M. (2009) RAGE: therapeutic target and biomarker of the inflammatory response–the evidence mounts. J Leukoc Biol 86:505–512
- Ramasamy, R., Yan, S.F. and Schmid, A.M. (2011) Receptor for AGE (RAGE): signaling mechanisms in the pathogenesis of diabetes and its complications. Ann NY Acad Sci 1243:88–102
- Rao, R.K., Seth, A. and Sheth, P. (2004) Recent Advances in Alcoholic Liver Disease I. Role of intestinal permeability and endotoxemia in alcoholic liver disease. Am J Physiol Gastrointest Liver Physiol 286:881–884
- Reddy, M.M., Mahipal, S.V., Subhashini, J., Reddy, M.C., Roy, K.R., Reddy, G.V., et al. (2006) Bacterial lipopolysaccharide-induced oxidative stress in the impairment of steroidogenesis and spermatogenesis in rats. Reprod Toxicol 22:493–500
- Schmidt, A.M., Sahagan, B., Nelson, R.B., Selmer, J., Rothlein, R. and Bell, J.M. (2009) The role of RAGE in amyloid-beta peptide-mediated pathology in Alzheimer's disease. Curr Opin Investig Drugs 10:672–680
- Shang, T., Zhang, X., Wang, T., Sun, B., Deng, T. and Han, D. (2011) Toll-like receptor-initiated testicular innate immune responses in mouse Leydig cells. Endocrinology 152:2827–2836
- Shi, Q., Hales, D.B., Emanuele, N.V. and Emanuele, M.A. (1998) Interaction of ethanol and nitric oxide in the hypothalamic-pituitary-gonadal axis in the male rat. Alcohol Clin Exp Res 22:1754–1762
- Siu, M.K. and Cheng, C.Y. (2004) Dynamic cross-talk between cells and the extracellular matrix in the testis. Bioessays 26:978–992
- Stríz, I. and Trebichavský, I. (2004) Calprotectin- a pleiotropic molecule in acute and chronic inflammation. Physiol Res 53:245–253
- Tamai, H., Horie, Y., Kato, S., Yokoyama, H. and Ishii, H. (2002) Long-term ethanol feeding enhances susceptibility of the liver to orally administered lipopolysaccharides in rats. Alcohol Clin Exp Res 26:75S–80S
- Uesugi, T., Froh, M., Arteel, G.E., Bradford, B.U., Wheeler, M.D., Gabele, E., et al. (2002) Role of lipopolysaccharide-binding protein in early alcohol-induced liver injury in mice. J Immunol 168:2963–2969
- Vogl, T., Tenbrock, K., Ludwig, S., Leukert, N., Ehrhardt, C., van Zoelen, M.A., et al. (2007) Mrp8 and Mrp14 are endogenous activators of Toll-like receptor 4, promoting lethal, endotoxin-induced shock. Nat Med 13:1042–1049
- Volz, H.C., Laohachewin, D., Seidel, C., Lasitschka, F., Keilbach, K., Wienbrandt, A.R., et al. (2012) S100A8/A9 aggravates post-ischemic heart failure through activation of RAGE-dependent NF-κB signaling. Basic Res Cardiol 107:250
- Yan, S.F., Yan, S.D., Ramasamy, R., Schmidt, A.M. (2009) Tempering the wrath of RAGE: an emerging therapeutic strategy against diabetic complications, neurodegeneration and inflammation. Ann Med 41:408–422
- Zhu, Q., Meisinger, J., Emanuele, NV., Emanuele, M.A., LaPaglia, N. and Van Thiel, D.H. (2000) Ethanol exposure enhances apoptosis within the testes. Alcohol Clin Exp Res 24:1550–1556