Abstract
Multiple inter-connected signaling pathways, involving kinases and phosphatases, form a framework that controls sperm motility, function, and fertilizing ability. Methods that give a broad view of the proteomic landscape may prove valuable in uncovering new crosstalk connections, as well as in discovering new proteins within this regulatory framework. A multi-immunoblotting strategy was utilized to evaluate this concept on human and porcine spermatozoa samples. In human and porcine spermatozoa, a diversity of kinases were identified including protein kinase A (PKA), protein kinase B (PKB), isoforms of protein kinase C (PKC), calmodulin-dependent kinases (CAMK), casein kinase (CK), and isoforms of glycogen synthase kinase (GSK3). Several phosphatases, such as protein phosphatase (PP)-1, PP2A, PP2C, and mitogen activated protein kinase (MAPK) phosphatase (MKP-1), were identified in human spermatozoa. The phosphorylation epitopes recognized belonged to members of the MAPK family, in addition to α and β isoforms of GSK3 and cAMP response element binding protein (CREB). Proteomic approaches that allow a broad view may aid in understanding the crosstalk between signaling systems in spermatozoal physiology.
Introduction
Signal transduction pathways involving multiple kinases and phosphatases form a framework that controls sperm motility, function, and fertilizing ability. The effects of calcium and cAMP-dependent proteins on spermatozoal activity are well established [Brokaw Citation1987; De Jonge Citation1996; Doherty et al. Citation1995; Talbot et al. Citation1976]. Protein kinase B (PKB/Akt) has been more recently recognized as another important component in the signaling cascades that direct sperm physiology [Aquila et al. Citation2004; Gallardo Bolanos et al. Citation2014; Jungnickel et al. Citation2007; Nauc et al. Citation2004; Vadnais et al. Citation2013]. Evidence that Akt may affect sperm behavior stemmed from work with wortmannin, a phosphoinositide-3 kinase (PI3K) inhibitor [Fisher et al. Citation1998]. This protein, PI3K, was known to act as an upstream regulator of Akt. Additional studies uncovered further clues for Akt involvement by identifying a downstream target, glycogen synthase kinase 3 (GSK3), present in spermatozoa [Vijayaraghavan et al. Citation1996; Vijayaraghavan et al. Citation2000]. The acquisition of sperm motility was associated with decreased GSK3 activity [Vijayaraghavan et al. Citation1996]. Thus, two components in the PI3K-Akt-GSK3 pathway were known to be present in sperm, with Akt only later being identified [Aquila et al. Citation2004; Nauc et al. Citation2004]. In addition to protein kinases, the phosphatases that modulate these kinases have a vital role in sperm physiology [Rotfeld et al. Citation2014; Signorelli et al. Citation2012]. A relationship was noted between protein phosphatase-1 (PP-1) and GSK3 [Vijayaraghavan et al. Citation1996]. Future studies would reveal further connections and help to solve this physiological puzzle, with PP-1 acting as a negative regulator by dephosphorylating Akt, a negative regulator of GSK3 [Thayyullathil et al. Citation2011]. Mathematically, one would expect an increase to result from the removal of a negative (subtracting a negative). Experiments on sperm from mice lacking Akt corroborated these deductions of elevated GSK3 with impaired motility and fertility [Kim et al. Citation2012]. Building on the work of these studies, the importance of this pathway and its ability to crosstalk with other signaling cascades are better understood [Aparicio et al. Citation2007; Bragado et al. Citation2010; Somanath et al. Citation2004].
Methods that give a broad view of the proteomic landscape may prove valuable in filling in these missing pieces by uncovering new connections as well as discovering new proteins. Previous research in our laboratory considering similarities in growth factors and signaling proteins between humans and farm animals lead to the investigation of boar and human spermatozoa in a multi-immunoblotting strategy offered by Kinexus [Henricks et al. Citation1998]. Both boar and human spermatozoa were analyzed for kinases and phosphorylated proteins. Human sperm samples were also processed for phosphatase identification. This strategy can also be adapted to aid in elucidating the activities of compounds and proteins that affect fertility and reproduction in a variety of species.
Results and Discussion
Kinases in porcine spermatozoa
Immunoblot scans of the 28 proteins identified by the Kinetworks™ Protein Kinase Screen (KPKS) in porcine are displayed in . lists the location of the kinases shown in , as well as their molecular weight and relative lane abundance. Protein kinase B (PKB/Akt) was identified in this screen as well as its downstream targets GSK3α and GSK3β. Other proteins activated by phosphoinositides include PKCλ/ι and PKCζ. Calcium and cAMP regulated proteins including calmodulin-dependent kinases (CAMK) and protein kinase A (PKA) were identified, respectively. Additionally, multiple members of the casein kinase 2 (CK2) and ribosomal S6-kinase (RSK) families were present.
Figure 1. Kinetworks™ Protein Kinase Screen (KPKS) of porcine spermatozoa. Known proteins are marked with an arrow and labeled with a ‘p’ for porcine and a corresponding number which can be used with the key for identification. A: proteins p1-p18 and B: proteins p19-p28.
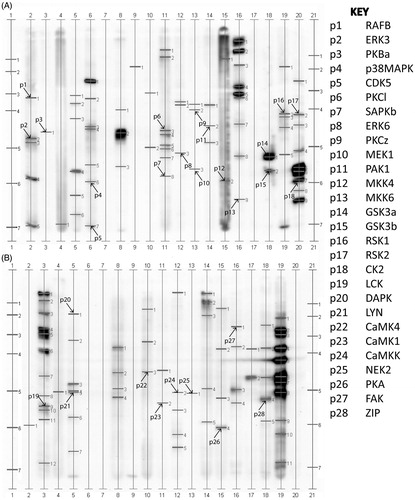
Table 1. Kinases in porcine spermatozoa.
Proteins in human spermatozoa
Immunoblot scans of the 34 proteins identified by the KPKS screen in human sperm are displayed in . lists the location of the kinases in , as well as their molecular weight and relative lane abundance. As in porcine sperm, Akt and GSK3α and β were identified. In addition the upstream Akt regulator phosphoinositide-dependent kinase (PDK1) was identified. Multiple members of PKC, ERK, MAPK, CAMK, and CK2 families were identified in human sperm. , is a blot of Akt identified in human sperm by two different antibodies.
Figure 2. Kinetworks™ Protein Kinase Screen (KPKS) of human spermatozoa. Known proteins are marked with an arrow and labeled with an ‘h’ for human and a corresponding number which can be used with the key for identification. A: proteins h1-23 and B: proteins h24-34. Results of Western blot: Lanes 1 and 2 were incubated with Ab1 and Lanes 3 and 4 were incubated with Ab2. Lanes 1 and 3 are extracts of HCT-8 cells used as positive controls. Lanes 2 and 4 are sperm extracts.
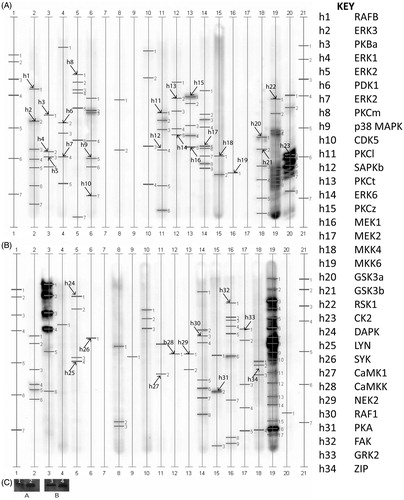
Table 2. Kinases in human spermatozoa.
Phosphatases and phosphorylation sites
lists the identities of the phosphatases and their location shown in . Multiple phosphatases from human sperm were identified including protein phosphatase (PP-1), PP2A, PP2C, and MAP kinase phosphatase (MKP-1). Phosphorylation epitopes recognized in are listed in and include several members of the MAPK family, in addition to α and β isoforms of GSK3 and the multi-functional cAMP response element binding protein (CREB).
Figure 3. Kinetworks™ Protein Phosphatase Screen (KPPS) phosphatase and Kinetworks™ Phosphosite Screen (KPSS) phosphorylation site screens of human spermatozoa. Known proteins are marked with an arrow and labeled with a ‘s’ for phosphatase or a ‘k’ for phosphorylation and a corresponding number which can be used with the Key for identification. A: phosphatases along with B: phosphorylated proteins are indicated.
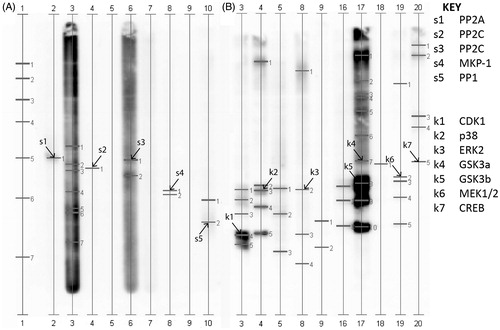
Table 3. Results from phosphatase and phosphorylation site screens.
Protein expression patterns in human and porcine sperm
presents a qualitative comparison of protein expression in porcine and human sperm (Supplemental Table 1 cross-references mouse proteins). Proteins with the greatest band intensity were normalized to 100 and other proteins were expressed as a percentage to establish relative abundance. Because of possible differences in antibody and species sensitivities strict quantitative analyses is not advisable without controlling for these variables. A recent review explores the role of these proteins in kinase cascades in several species [Lackey and Gray Citation2015].
Table 4. Comparison of protein expression patterns in human and porcine sperm.
The multi-immunoblotting strategy used in Kinexus screens offers time and cost savings when trying to visualize the proteomic landscape. In the screen for kinases in boar and human sperm samples, components of multiple signaling cascades were identified that are known to be regulators of sperm physiology. Proteins identified in the kinase screen included members of PKA, PKC, and PKB/Akt pathways and regulators such as CAMK, ERKs, and MAPKs [Almog et al. Citation2008; Bernabò et al. Citation2010; Bragado et al. Citation2010; Gallardo Bolanos et al. Citation2014; González-Fernández et al. Citation2013; Mannowetz et al. Citation2010]. Ribosomal S6 kinases were identified in porcine sperm and human sperm [Sagare-Patil and Modi Citation2013]. Cyclin-dependent kinase 5, CK2, and RAFB were also identified for the first time in porcine spermatozoa. In addition to the qualitative results, semi-quantitative data could be acquired in the future by calibration to different quantities of protein extract loaded per lane. Future research could ascertain if the CK2 abundance is associated with fertility. In addition, specific preparative methods could be used to enrich cellular components such as the mitochondrial fractions or membranes to complement the immunocytochemistry and better understand the activities of these proteins in affecting mitochondrial and sperm function [Gallardo Bolanos et al. Citation2014]. Comparisons of protein expression from total sperm with swim-up selected and low-motility populations could also be screened to better understand differences between high-fertility and lower-fertility samples. Other approaches can also give views of the proteomic landscape such as a phosphoproteomic UPLC-MS method that identified multiple proteins altered in asthenozoospermia [Parte et al. Citation2012]. Among these proteins with altered expression were PKA and Akt [Parte et al. Citation2012]. The phosphatase screen identified multiple phosphatases that regulate the activity of these protein kinases, including serine-threonine phosphatases, such as PP-1, PP2A, and PP2C as well as MKP-1, a dual specificity phosphatase (DUSP) that targets threonine and tyrosine residues. In the screen of phosphorylation sites, members of cAMP, MAPK, and AKT-GSK3 pathways were identified. The expression patterns for both GSK3 isoforms in human and porcine sperm appears to follow that of bovine sperm with the α isoform having higher expression [Somanath et al. Citation2004]. Screening of this type may yield interesting data when comparing samples of high and low fertility. Furthermore, several unidentified phosphorylated proteins were detected after progesterone and IGF-stimulation. Subsequent isolation of these unidentified proteins combined with mass-spectrometric sequencing could be utilized to identify new proteins and reveal new interactions. The multiple signaling pathways in human and porcine spermatozoa identified using the approach described may help in understanding the complex relationships between these systems in male reproductive physiology.
Materials and Methods
Spermatozoa
Discarded frozen samples provided by the ART Laboratory at Greenville Health System came from anonymized donors ranging in age from 25 to 40 y who were free of HIV and hepatitis C. Because no patient data were involved, this study did not require Internal Review Board approval. Samples used had no extender other than glycerol and were thawed in a water bath at 40 °C for 5 min. Three samples of good motility (>75%) were pooled and extracted. Sperm concentration and motion parameters were assessed with the IVOS semen analyzer (Hamilton Thorne Research, Beverly, MA, USA).
Boar spermatozoa were obtained from high-fertility Duroc breed animals through Lean Value Sires (New Carlisle, OH, USA). Semen was shipped overnight to the laboratory and packaged so the temperature was maintained at approximately 17 °C. Methods for preparing the spermatozoa utilized non-toxic plastics [Johnson et al. Citation1996; Mortimer Citation1994]. The semen from several boars was pooled and centrifuged at 400 × g for 5 min to remove the extender. Spermatozoa samples were washed twice with 0.2 mM NaHCO3 Tyrode's Albumin-Lactate-Pyruvate Media (TALP) and resuspended in 0.5 mL media [Gray et al. Citation2015].
Processing
Motile human and boar spermatozoa were each selectively separated from the pellet using the swim-up method for immunoblotting analysis to enrich low-abundance proteins [Lackey et al. Citation1998; Mortimer Citation1994]. The supernatants containing the motile spermatozoa were carefully pipetted, pooled, and processed [Gray et al. Citation2015; Henkel and Schill Citation2003; Lalancette et al. Citation2006].
Progesterone and IGF-1 reagents were purchased from Sigma (St. Louis, MO, USA). Sperm were incubated for 30 min at 37 °C with 100 ng/mL IGF-1 and 1 µg/mL progesterone added to TALP for induction and analysis in the Kinetworks™ Phosphosite Screen (KPSS) phosphorylation site screen [Blackmore et al. Citation1990; Kaya et al. Citation2012; Oberlender et al. Citation2013]. Induced and non-induced swim-up sperm samples were prepared for Western blotting or Kinexus analysis after being lysed in 500 µL of Cytobuster reagent per mL of sperm pellet (EMD Millipore, Billerica, MA, USA) [Martins-Branco et al. Citation2012]. In addition, protease and phosphatase inhibitors were added to the lysis mixture. Phosphatase I and II inhibitors were from Sigma and the Halt protease inhibitor was from Thermo-Fisher (Rockford, IL, USA), both were used according to manufacturer’s instructions.
Protein identification
Secondary antibodies, transfer membranes, reagents, and film used for detection were purchased from Thermo-Fisher. Human lysates were quantified by the Bradford protein method and after boiling for 5 min, 30 μg of protein was separated under denaturing SDS-PAGE on 10% polyacrylamide Tris-glycine gels (Bio-Rad, Hercules, CA, USA), and then electroblotted to PVDF membranes [Aquila et al. Citation2004; Gallardo Bolanos et al. Citation2014; Vijayaraghavan et al. Citation2000]. Non-specific sites were blocked with 5% non-fat dry milk in 0.2% Tween-20 in Tris-buffered saline (TBST) for 1 h at room temperature. Primary antibodies were incubated overnight at 4 °C. A primary mouse anti-Akt antibody (Ab1) against the pleckstrin homology domain corresponding to residues 1-149 of human Akt1 was purchased from EMD Millipore (Billerica, MA, USA) and used at a dilution of 1:500. A primary rabbit anti-Akt antibody against amino acids 345-480 of Akt1/2 of human origin (Ab2) was acquired from Santa Cruz Biotechnology (Dallas, TX, USA) and used at dilution of 1:200. Protein extracts from the HCT-8 cell line were used as a positive control. Membranes were incubated with their respective goat-anti-rabbit or goat-anti-mouse HRP-labeled secondary antibodies at room temperature for 1 hr. Secondary antibodies were detected with SuperSignal™ West Femto Maximum Sensitivity Substrate according to the manufacturer's instructions. Each membrane was exposed CL-XPosure films for 2 min [Rago et al. Citation2014].
Human and porcine sperm samples were analyzed in KPSS and KPKS; human samples were also subjected to KPPS (see http://www.kinexus.ca for more information). A total of 600 µg lysate was prepared for KPKS and KPPS and 350 µg for KPSS [Pelech Citation2004]. Briefly, a multi-immunoblot system is applied using screened antibodies for protein identification and detection [Pelech et al. Citation2003; Pelech Citation2004]. After chemiluminescent detection the protein signals are quantified by an optical scanner and results provided in image and table formats [Pelech et al. Citation2003; Pelech Citation2004].
Supplementary material available online
IAAN_1089335_Supplemental_File.zip
Download Zip (64.7 KB)Acknowledgments
The authors wish to acknowledge Dr. William Boone for assistance and access to samples and Dr. Donald M. Henricks for guidance and expertise.
Declaration of interest
The authors report no conflicts of interest. The authors alone are responsible for the content and writing of the paper.
Author contribution
Conceived and designed the experiments: BL, SG; Performed the experiments: BL, SG; Analyzed the data: BL,SG; Contributed reagents and materials: SG; Both authors wrote the manuscript and approved the revised paper.
References
- Almog, T., Lazar, S., Reiss, N., Etkovitz, N., Milch, E., Rahamim, N., et al. (2008) Identification of extracellular signal-regulated kinase 1/2 and p38 MAPK as regulators of human sperm motility and acrosome reaction and as predictors of poor spermatozoan quality. J Biol Chem 283: 14479–14489
- Aparicio, I.M., Bragado, M.J., Gil, M.C., Garcia-Herreros, M., Gonzalez-Fernandez, L., Tapia, J.A., et al. (2007) Porcine sperm motility is regulated by serine phosphorylation of the glycogen synthase kinase-3alpha. Reproduction 134: 435–444
- Aquila, S., Sisci, D., Gentile, M., Middea, E., Catalano, S., Carpino, A., et al. (2004) Estrogen receptor (ER)alpha and ER beta are both expressed in human ejaculated spermatozoa: evidence of their direct interaction with phosphatidylinositol-3-OH kinase/Akt pathway. J Clin Endocrinol Metab 89: 1443–1451
- Bernabò, N., Mattioli, M. and Barboni, B. (2010) The spermatozoa caught in the net: the biological networks to study the male gametes post-ejaculatory life. BMC Syst Biol 4: 87
- Blackmore, P.F., Beebe, S.J., Danforth, D.R. and Alexander, N. (1990) Progesterone and 17 alpha-hydroxyprogesterone. Novel stimulators of calcium influx in human sperm. J Biol Chem 265: 1376–1380
- Bragado, M.J., Aparicio, I.M., Gil, M.C. and Garcia-Marin, L.J. (2010) Protein kinases A and C and phosphatidylinositol 3 kinase regulate glycogen synthase kinase-3A serine 21 phosphorylation in boar spermatozoa. J Cell Biochem 109: 65–73
- Brokaw, C.J. (1987) Regulation of sperm flagellar motility by calcium and cAMP-dependent phosphorylation. J Cell Biochem 35: 175–184
- De Jonge, C. (1996) The cAMP-dependent kinase pathway and human sperm acrosomal exocytosis. Front Biosci 1: d234–d240
- Doherty, C.M., Tarchala, S.M., Radwanska, E. and De Jonge, C.J. (1995) Characterization of two second messenger pathways and their interactions in eliciting the human sperm acrosome reaction. J Androl 16: 36–46
- Fisher, H.M., Brewis, I.A., Barratt, C.L., Cooke, I.D. and Moore, H.D. (1998) Phosphoinositide 3-kinase is involved in the induction of the human sperm acrosome reaction downstream of tyrosine phosphorylation. Mol Hum Reprod 4: 849–855
- Gallardo Bolanos, J.M., Balao da Silva, C.M., Martin Munoz, P., Morillo Rodriguez, A., Plaza Davila, M., Rodriguez-Martinez, H., et al. (2014) Phosphorylated AKT preserves stallion sperm viability and motility by inhibiting caspases 3 and 7. Reproduction 148: 221–235
- González-Fernández, L., Macías-García, B., Loux, S.C., Varner, D.D. and Hinrichs, K. (2013) Focal adhesion kinases and calcium/calmodulin-dependent protein kinases regulate protein tyrosine phosphorylation in stallion sperm. Biol Reprod 88: 138
- Gray, S.L., Lackey, B.R. and Boone, W.R. (2015) Impact of Kudzu and Puerarin on Sperm Function. Reprod Toxicol 53:54–62
- Henkel, R. and Schill, W.-B. (2003) Sperm preparation for ART. Reprod Biol Endocrinol 1: 108
- Henricks, D.M., Kouba, A.J., Lackey, B.R., Boone, W.R. and Gray, S.L. (1998) Identification of insulin-like growth factor I in bovine seminal plasma and its receptor on spermatozoa: influence on sperm motility. Biol Reprod 59: 330–337
- Johnson, J.E., Boone, W.R. and Blackhurst, D.W. (1996) Manual versus computer-automated semen analyses. Part II. Determination of the working range of a computer-automated semen analyzer. Fertil Steril 65: 156–159
- Jungnickel, M.K., Sutton, K.A., Wang, Y. and Florman, H.M. (2007) Phosphoinositide-dependent pathways in mouse sperm are regulated by egg ZP3 and drive the acrosome reaction. Dev Biol 304: 116–126
- Kaya, A., Atabekoglu, C.S., Kahraman, K., Taskin, S., Ozmen, B., Berker, B., et al. (2012) Follicular fluid concentrations of IGF-I, IGF-II, IGFBP-3, VEGF, AMH, and inhibin-B in women undergoing controlled ovarian hyperstimulation using GnRH agonist or GnRH antagonist. Eur J Obstet Gynecol Reprod Biol 164: 167–171
- Kim, S.T., Omurtag, K. and Moley, K.H. (2012) Decreased spermatogenesis, fertility, and altered Slc2A expression in Akt1-/- and Akt2-/- testes and sperm. Reprod Sci 19: 31–42
- Lackey, B.R., Boone, W.R., Gray, S.L. and Henricks, D.M. (1998) Computer-assisted sperm motion analysis of bovine sperm treated with insulin-like growth factor I and II: implications as motility regulators and chemokinetic factors. Arch Androl 41: 115–125
- Lackey, B.R. and Gray, S.L. (2015) Second messengers, steroids and signaling cascades: Crosstalk in sperm development and function. Gen Comp Endocrinol S0016–6480(15)00199-9. doi: 10.1016/j.ygcen.2015.07.005. [Epub ahead of print] Review. PMID: 26188217
- Lalancette, C., Faure, R.L. and Leclerc, P. (2006) Identification of the proteins present in the bull sperm cytosolic fraction enriched in tyrosine kinase activity: a proteomic approach. Proteomics 6: 4523–4540
- Mannowetz, N., Kartarius, S., Wennemuth, G. and Montenarh, M. (2010) Protein kinase CK2 and new binding partners during spermatogenesis. Cell Mol Life Sci 67: 3905–3913
- Martins-Branco, D., Esteves, A.R., Santos, D., Arduino, D.M., Swerdlow, R.H., Oliveira, C.R., et al. (2012) Ubiquitin proteasome system in Parkinson’s disease: A keeper or a witness? Exp Neurol 238: 89–99
- Mortimer, D. (1994) Practical Laboratory Andrology. Oxford University Press, London
- Nauc, V., De Lamirande, E., Leclerc, P. and Gagnon, C. (2004) Inhibitors of phosphoinositide 3-kinase, LY294002 and wortmannin, affect sperm capacitation and associated phosphorylation of proteins differently: Ca2+-dependent divergences. J Androl 25: 573–585
- Oberlender, G., Murgas, L.D.S., Zangeronimo, M.G., Silva, A.C. da, Menezes, T. de A., Pontelo, T.P., et al. (2013) Role of insulin-like growth factor-I and follicular fluid from ovarian follicles with different diameters on porcine oocyte maturation and fertilization in vitro. Theriogenology 80: 319–327
- Parte, P.P., Rao, P., Redij, S., Lobo, V., D’Souza, S.J., Gajbhiye, R., et al. (2012) Sperm phosphoproteome profiling by ultra performance liquid chromatography followed by data independent analysis (LC-MS(E)) reveals altered proteomic signatures in asthenozoospermia. J Proteomics 75: 5861–5871
- Pelech, S. (2004) Tracking cell signaling protein expression and phosphorylation by innovative proteomic solutions. Curr Pharm Biotechnol 5: 69–77
- Pelech, S., Sutter, C. and Zhang, H. (2003) Kinetworks protein kinase multiblot analysis. Methods Mol Biol 218: 99–111
- Rago, V., Giordano, F., Brunelli, E., Zito, D., Aquila, S. and Carpino, A. (2014) Identification of G protein-coupled estrogen receptor in human and pig spermatozoa. J Anat 224: 732–736
- Rotfeld, H., Hillman, P., Ickowicz, D. and Breitbart, H. (2014) PKA and CaMKII mediate PI3K activation in bovine sperm by inhibition of the PKC/PP1 cascade. Reproduction 147: 347–356
- Sagare-Patil, V. and Modi, D. (2013) Progesterone activates Janus Kinase 1/2 and activators of transcription 1 (JAK1-2/STAT1) pathway in human spermatozoa. Andrologia 45: 178–186
- Signorelli, J., Diaz, E.S. and Morales, P. (2012) Kinases, phosphatases and proteases during sperm capacitation. Cell Tissue Res 349: 765–782
- Somanath, P.R., Jack, S.L. and Vijayaraghavan, S. (2004) Changes in sperm glycogen synthase kinase-3 serine phosphorylation and activity accompany motility initiation and stimulation. J Androl 25: 605–617
- Talbot, P., Summers, R.G., Hylander, B.L., Keough, E.M. and Franklin, L.E. (1976) The role of calcium in the acrosome reaction: an analysis using ionophore A23187. J Exp Zool 198: 383–392
- Thayyullathil, F., Chathoth, S., Shahin, A., Kizhakkayil, J., Hago, A., Patel, M., et al. (2011) Protein phosphatase 1-dependent dephosphorylation of Akt is the prime signaling event in sphingosine-induced apoptosis in Jurkat cells. J Cell Biochem 112: 1138–1153
- Vadnais, M.L., Aghajanian, H.K., Lin, A. and Gerton, G.L. (2013) Signaling in sperm: toward a molecular understanding of the acquisition of sperm motility in the mouse epididymis. Biol Reprod 89: 127
- Vijayaraghavan, S., Mohan, J., Gray, H., Khatra, B. and Carr, D.W. (2000) A role for phosphorylation of glycogen synthase kinase-3alpha in bovine sperm motility regulation. Biol Reprod 62: 1647–1654
- Vijayaraghavan, S., Stephens, D.T., Trautman, K., Smith, G.D., Khatra, B., Cruz e Silva, E.F. da, et al. (1996) Sperm motility development in the epididymis is associated with decreased glycogen synthase kinase-3 and protein phosphatase 1 activity. Biol Reprod 54: 709–718