Abstract
The composition of sperm proteins influences the fertilizing ability of sperm and hence the present study was conducted (i) to profile sperm proteins expression patterns in bulls of differing fertility index and (ii) to identify and relate the abundant sperm proteins with bull fertility. The semen samples were collected from Holstein-Friesian bulls (n = 12) varying in conception rate (CR) (high/low). The frozen semen straws (three ejaculates, from each bull) were used to study (a) sperm kinetic parameters, (b) plasmalemma integrity, (c) mitochondrial membrane potential, and (d) chromatin distribution. Three bulls were randomly selected from each group (n = 3) and the neat sperm pellets were subjected to percoll purification, followed by protein isolation using 0.1% Triton X100. The sperm kinetic parameters, plasmalemma integrity, mitochondrial membrane potential, and the chromatin distribution did not differ significantly between groups. The number of acidic (pI; 3.1–5.6, 37%) and basic (pI; 7.9–10.0, 27%) proteins and their pattern of expression varied significantly (p < 0.05) between high and low fertile bulls. The abundant sperm protein spots in 2D-gel electrophoresis (2DE) were identified as seminal plasma protein PDC-109 (i.e., protein with N-terminus aspartic acid, D and carboxy terminus cystine, having 109 amino acids) and its isoform and spermadhesin-1 (SPADH1). The western blot analysis confirmed the presence of PDC-109 isoform proteins at 15.4 kDa (pI 5.3 and 5.5). The seminal plasma protein PDC-109 was abundant in the low fertile when compared to the high fertile group (p < 0.05). This study suggests that the imbalance in acidic and basic sperm proteins may influence sperm fertility and sperm PDC-109 levels above a certain threshold affects bull fertility.
Introduction
Bull fertility assessment using in vitro sperm functional attributes is not sufficient in predicting the conception rate (CR) with artificial insemination (AI) [Park et al. Citation2013] and hence functional genomics and metabolomic approaches have been focused to assess bull fertility [Kovac et al. Citation2013; Liu et al. Citation2014]. The sperm associated as well as the seminal plasma proteins regulate the events integrated with sperm maturation, fertilization, and early embryonic development [Arangasamy et al. Citation2005; Manjunath and Therien Citation2002; O'Leary et al. Citation2004]. The alterations in sperm proteins involved in chromatin assembly and metabolism might result in inaccurate epigenetic signatures leading to failed embryonic development [Azpiazu et al. Citation2014].
During semen cryopreservation, the seminal plasma is diluted approximately 10–20 fold and hence the influence of seminal plasma proteins on fertility may be limited. Thus, the proteins present in the sperm, i.e., sperm specific proteins along with proteins acquired from accessory sex glands, are critical for the sperm to achieve fertilization competence [Rego et al. Citation2014]. In bull sperm, the proteomic analysis revealed a significant quantitative difference in eight proteins between high and low fertile bulls and suggested that the imbalance in sperm proteins might affect sperm fertilizing ability [D'Amours et al. Citation2010; Soggiu et al. Citation2013]. The expression profile of acid seminal fluid protein (aSFP) was 8.5 times greater in high fertility bulls and binder of sperm protein (BSP-A3) was 2.5 times greater in the lower fertility bulls [Roncoletta et al. Citation2006]. Recently, two-dimensional difference gel electrophoresis (2D-DIGE) based proteomic profiling of spermatogenic and sertoli cells were used to differentiate prepubertal bulls with altered fertility status [Tripathi et al. Citation2014]. The comparison of proteomic status of bulls varying in fertility by 2D-gel electrophoresis (2DE) and the identification of proteins by mass spectrometry could be a potential approach for fertility assessment [Liu, et al. Citation2014; Park et al. Citation2012; Peddinti et al. Citation2008; Pixton et al. Citation2004]. The complete proteome analysis of fertile individuals is obligatory to develop the fertility markers using bioinformatic approaches [Amaral et al. Citation2014; Oliva et al. Citation2010].
The selection of a prime bull for AI is critical and economically important, as it can influence the fertility and productivity of approximately 300,000 cows through AI. Under field conditions, the conception rate varies from 30–45% and to improve this, it is important to develop a marker for predicting bull fertility. So far, no protein has been identified as a fertility prediction marker [Rahman et al. Citation2013]. Since a single protein/molecule alone may not influence the events associated with fertilization, the objectives of this study were (i) to profile sperm proteins using 2DE to analyze protein expression patterns in the bulls differing in fertility index and (ii) to identify and relate the abundant proteins of sperm with bull fertility.
Results
Sample strategy
The percentage of pregnancy rate for each Holstein-Friesian bull in a batch of contemporaries (n = 20; maintained at Nandini sperm station, Bengaluru, India) was calculated from approximately 1,500 AI/bull. Then the bulls were selected based on the percentage point deviation (high fertile, n = 6, average bull fertility Index, +2.0 and low fertile n = 6, average bull fertility Index, −2.0) of the pregnancy rate from the average fertility of their contemporary bulls (). The semen samples (three ejaculates/bull) were collected and an aliquot of neat semen sample (1 mL) was subjected to centrifugation at 7,000x g at 4 °C for 10 min to separate the seminal plasma and sperm. The sperm pellet was snap frozen in liquid nitrogen and stored at −80 °C until protein isolation. The remaining semen samples were diluted in tris-egg yolk extender and filled in 250 µL straws and then cryopreserved using a fast programmable freezer. The frozen semen straws were used for the assessment of sperm attributes.
Figure 1. Grouping of bulls was carried out based on conception rate. In (A), the conception rate for each bull in the semen bank was calculated from approximately 1,500 inseminations per bull. Bulls were grouped as high fertile (n = 6) and low fertile (n = 6) based on bull fertility index calculated using the average conception rate of their contemporary bulls (n = 20) in the semen bank. In (B), the conception rate significantly (p < 0.001) differed between high and low fertile groups.
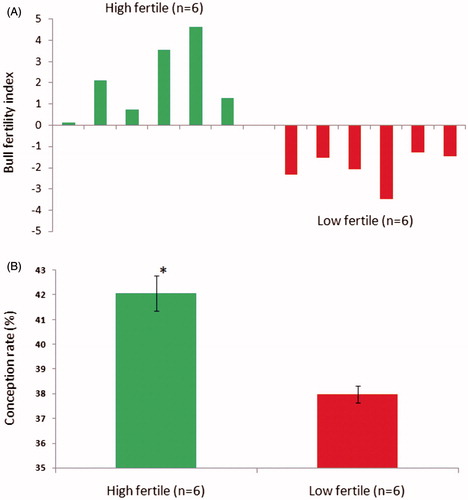
Assessment of sperm attributes
The sperm kinetic parameters, plasmalemma integrity, mitochondrial membrane potential, and chromatin distribution did not differ significantly between the high and low fertile groups (). The sperm attributes did not have a significant correlation with CR (Supplementary Table 1).
Table 1. Sperm kinetic parameters, plasmalemma integrity, mitochondria membrane potential, and chromatin distribution in high and low fertile bull frozen thawed semen.
Sperm 2DE-protein mapping
Sperm protein profiles and the patterns of protein expression between high and low fertile bulls were compared and protein spot abundance was analyzed by matched spot plot analysis (). The abundance of proteins in relation to iso-electric pH was considered for generating the iso-electric point based 2DE-protein map between fertility groups. The 2DE-protein profile revealed a differential expression of proteins towards acidic pH (pI; 3.0 to 5.6, 37% distribution in sperm) and basic pH (pI; 7.9 to 10.0, 27% distribution in sperm) regions. The protein profile pattern was considered to be similar at pI 5.7 to 7.8 ().
Figure 2. Sperm protein profile between high and low fertile bulls was analyzed in Dymension 2 software (Syngene, UK). In Panel A, the protein profile matching protocol was followed as per the recommended guidelines. In Panel B, the combined image was generated to analyze the protein spots matching between fertility groups and sample alignment was confirmed with 3D profile matching. The target protein spot abundance was analyzed by matched spot plot to obtain its protein abundance in high and low fertile groups.
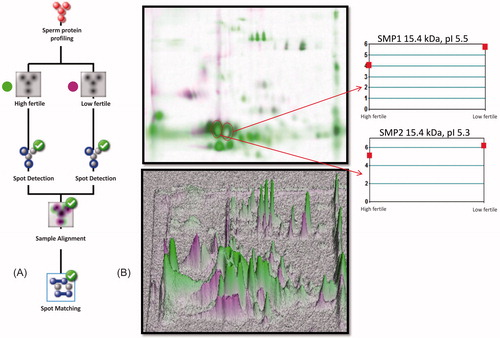
Figure 3. 2DE-protein mapping of spermatozoa showing differential expression profile between high and low fertile groups. (A) Different pH gradients were analyzed (pH 3.1–5.6, 5.7–7.8 and 7.9–10.0), proteins areas under pH 5.7–7.8 was less varied compared to other pH gradients. (B) Spermatozoa proteomic distribution as per pI nature of proteins.
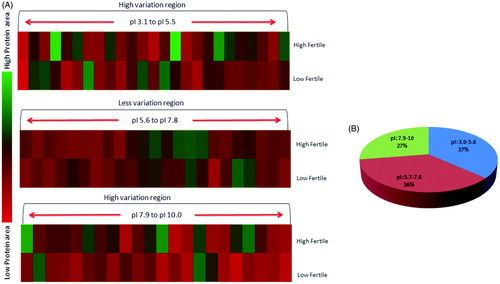
Identification of proteins and expression analysis
The abundant sperm protein spots () in 2D-gels were excised and peptide fingerprints were obtained using MALDI-TOF in order to identify the proteins. The spots, SMP1 () and SMP2 () were identified as seminal plasma protein PDC-109 (15.4 kDa; pI 5.3 and pI 5.5) and the spot SMP3 () was identified as spermadhesin-1 (SPADH1) 15.0 kDa; pI 5.3 (). The abundance of PDC-109 isoforms was significantly (p < 0.05) greater in bulls with a low conception rate (). The protein abundance of SPADH1 did not significantly differ between high and low fertile bulls. Further, the western blot analysis confirmed the presence of PDC-109 at 15.4 kDa (pI 5.3 and 5.5) (). The relative expression of normalized raw volume of PDC-109 was significantly higher in the low fertile group as compared to the high fertile group (p < 0.05) ().
Figure 4. Identification of 2DE protein spot by MALDI-TOF and corresponding spectra of proteins. In (A), protein spots SMP1, SMP2, and SMP3 were identified as major spots and excised from the gel for MALDI-TOF experiment. In (B-D), respective MALDI spectra and inserted bar graph indicating the peptide mass fingerprints of SMP1, SMP2, and SMP3 obtained in Mascot database search.
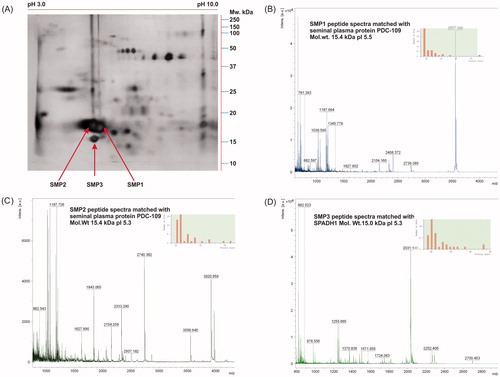
Figure 5. In (A) and (B), 2DE analysis of SMP1 and SMP2 (15.4 kDa) protein spots with pI 5.5 and 5.3. The expression levels were significantly higher in low fertile group when compared to high fertile group. (C) An immunocomplex formation of PDC-109 (BSPH1) antibodies with SMP1 and SMP2 was confirmed by 2DE followed by western blot analysis. (D) Relative expression by western blot analysis of PDC-109 differed significantly (p < 0.05) between fertility groups.
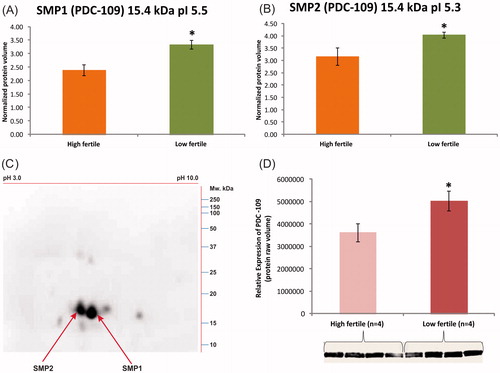
Table 2. Identification of the most abundant spermatozoa proteins by MALDI-TOF and the Mascot score, sequence coverage, and unique peptide reads obtained using Mascot online search engine (Swiss-Prot database) using peptide mass finger print online tool.
The PDC-109 protein interactions and its gene ontology
The PDC-109 was identified as a core for 8 major proteins (NPC2, ACR, PH-20, ZPBP, SPINK6, Apo-AI, CatSper3, and PB1) by STRING functional protein association network (Version 9.1) analysis (). The gene ontology (PANTHER classification system, Version 10.0) of PDC-109 interacting proteins under molecular functions exhibited its annotations with catalytic activity, binding, transporter activity, enzyme regulator activity, and receptor activity at different levels (). In biological process, the major annotations identified were metabolic process, cellular process, localization, and biological regulation (). The protein class, major annotations found were enzyme hydrolase, modulator, protease, receptor, and transporter proteins (). The major functions of interacting proteins of PDC-109 in relation to the Bos taurus genome were identified as cell recognition, cholesterol efflux, capacitation, acrosome reaction, and fertilization in functional annotation of genes using DAVID Bioinformatics Resources 6.7: Online Bioinformatic Database ().
Figure 6. (A) PDC-109 interactions were analyzed in STRING online software using organism, Bos taurus. The PDC-109 is identified as a core for eight major proteins (NPC2, ACR, PH-20, ZPBP, SPINK6, Apo-AI, CatSper3, and PB1) involved in different molecular functions (B), biological process (C), and protein class (D) analyzed in PANTHER classification system. (STRING Access Date: 06.07.2014, Time: 2.21 pm; PANTHER Access Data: 20.06.2015, Time: 11.00 am).
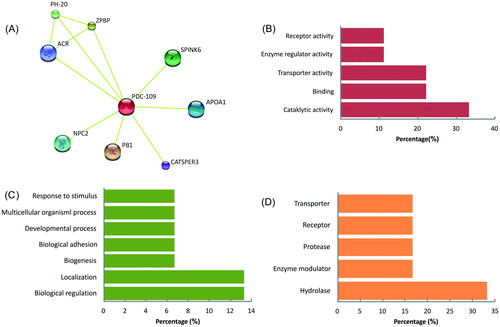
Table 3. The gene annotations of PDC-109 and its interacting proteins studied using DAVID Bioinformatics Resources 6.7 (online bioinformatic database) in specific to Bos taurus genome.
Discussion
The present study suggests that the functional attributes of spermatozoa are insufficient to assess semen quality and hence molecular evaluation of semen might be essential to assess bull fertility. It has been established that the majority of sperm membrane anchoring proteins are secreted from the accessory sex glands [Rodriguez-Martinez et al. Citation2011]. The proteins binding with sperm during epididymal transit and ejaculation are essential for successful fertilization and healthy embryonic development [Bromfield et al. Citation2014] since the seminal plasma proteins are diluted approximately 10–20 times with tris-egg yolk extender during the preparation of semen straws.
As a prerequisite, sperm attributes were compared between high and low fertile groups and it was observed that the sperm functional parameters did not differ between groups and also no correlation was observed between sperm attributes and CR. This may be due to reduced accuracy of in vitro sperm functional parameters in predicting the critical differences in fertilization between high and low fertile groups [Boryshpolets et al. Citation2013; Vasan Citation2011]. Hence predicting bull fertility status based on sperm attributes might not be an accurate bull fertility screening parameter [Oehninger et al. Citation2000] and should perhaps only be used for preliminary screening to assess semen quality [Rodriguez-Martinez Citation2013].
The sperm 2DE protein profile varied between groups at different pH gradients (). This finding suggested that the imbalance in proteomic distribution in acidic and basic pH regions could be one of the reasons for altered CR between high and low fertile groups. It has been established that the acidic class of proteins such as spermadhesins and binder of sperm proteins binds to spermatozoa during epidydimal transit before ejaculation which regulates sperm capacitation events in bovine [Dostalova et al. Citation1994; Hung and Suarez Citation2012]. Further, two-dimensional polyacrylamide gel electrophoresis of bovine seminal plasma proteins revealed the influence of abundant acidic proteins on semen freezability [Jobim et al. Citation2004] and the basic proteins might favor biological activities such as capacitation and acrosomal reaction of spermatozoa during fertilization [De Canio et al. Citation2014].
In the current study, the abundant sperm protein spots (SMP1, SMP2, and SMP3) were identified as seminal plasma protein PDC-109/BSP1 (SMP1 and SMP2) and SPADH1 (SMP3) by MALDI-TOF analysis coupled with peptide mass finger printing. The expression levels of PDC-109 and its isoforms (15.4 kDa; pI 5.3 and 5.5) were found to be significantly higher in the low fertile when compared to the high fertile group. However, the abundance of SPADH1 did not differ between groups. The 2DE and western blot analysis confirmed the presence of PDC-109 homologous proteins with the molecular weight of 15.4 kDa with pI 5.3 and 5.5 by immunocomplex formation with SMP1 and SMP2 protein spots. The relative expression of sperm PDC-109 was significantly higher in low fertile bulls when compared to high fertile bulls.
In bull semen, PDC-109 (BSP-A1/A2), BSP-A3, and BSP-30 kDa are the major secretory proteins from seminal vesicles [Manjunath et al. Citation1994]. The PDC-109 protein is a polypeptide of 109 amino acids with two tandem repeating fibronectin type-II (Fn-II) domains, proceeding to N-terminal domain, composed of 23 amino acid residues. Binding of PDC-109 to the plasma membrane of spermatozoa causes conformational changes of sperm membrane proteins and enhances progressive motility and promotes efflux of cholesterol and phospholipids leading to capacitation during fertilization [Therien et al. Citation1999; Thomas et al. Citation2003; Wah et al. Citation2002]. The PDC-109 (BSP-A1/A2 or BSP1) was localized in equatorial and post equatorial regions of the acrosomal membrane of spermatozoa [Kim et al. Citation2010; Souza et al. Citation2008]. It also enables the binding of sperm to oviductal epithelium and promotes the formation of bovine oviductal sperm reservoir to facilitate sperm capacitation and fertilization [Fiol de Cuneo et al. Citation2004; Gwathmey et al. Citation2003]. Very recently, a technique called sequestration of PDC-109 proteins in crossbreed bull semen resulted in significant improvements in semen freezability and sperm attributes [Srivastava et al. Citation2012]. In contradiction, the studies in relevance to PDC-109 indicate its pivotal importance in regulation of spermatozoa function.
The PDC-109 protein interaction network revealed its direct association with NCP2, ACR, PH-20, ZPBP, SPINK6, APOA1, CatSper3, and PB1 proteins. PDC-109 in association with other proteins that regulate sperm motility (catsper3, APOA1) [Jin et al. Citation2007; Kwon et al. Citation2014; Singh and Rajender Citation2014], acrosomal integrity (ACR), cholesterol transport (NCP2, PB1, APOA1) [Infante et al. Citation2008; Liou et al. Citation2006], acrosomal reaction (NCP2, PB1) [Lusignan et al. Citation2007], cumulus cell penetration (PH20, ACR) [Martin-DeLeon Citation2006], and zona binding (PH-20, ACR, ZPBP) [Zhou et al. Citation2012] functions during fertilization. PDC-109 and its interacting proteins are involved in sperm cholesterol efflux during capacitation/acrosome reaction (APOA1, PB1, SPINK6, NCP2, ACR, PH-20), sperm hyperactivation (CatSper3), and sperm egg interaction (ZPBP, APOA1, PH-20) during fertilization ().
PDC-109 could be considered as a potential modulator of fertility because of its mixed/diverse functions during fertilization. In the present study, a higher concentration of PDC-109 was associated with low fertility bulls. The possible explanation could be that the higher levels of PDC-109 binding to sperm membrane with phosphatidylcholines could induce premature capacitation by triggering the cholesterol efflux shunt in sperm through its interacting proteins. The higher concentration of seminal PDC-109 also increased susceptibility of sperm to cryoinjury in cattle [Srivastava et al. Citation2013] and buffalo [Singh et al. Citation2014]. The higher level of sperm PDC-109 might also induce cell damage either by increasing the sperm susceptibility to reactive oxygen species or cryo-capacitating the spermatozoa during cryopreservation (). Although positive influences of seminal plasma PDC-109 on male fertility have been reported, the present study revealed negative association of sperm PDC-109 with bull fertility. The higher levels of sperm PDC-109 might affect sperm fertilizing ability by inducing cryo-capacitation during cryopreservation.
Figure 7. Predictive pathway of PDC-109 induced premature sperm capacitation and cryo-capacitation by triggering sperm capacitation cascade.

The 2DE analysis of sperm proteins in the present study demonstrated that an imbalance in acidic and basic proteins reflects bull fertility. The abundant sperm proteins were identified as seminal plasma protein PDC-109, 15.4 kDa with isoforms of pI, 5.5 and 5.3, and spermadhesin-1, 15.0 kDa pI 5.3. The relative expression of sperm PDC-109 in bulls differing in their fertility index suggested that above a certain threshold level, PDC-109 might affect bull fertility.
Methodology
Assessment of sperm kinetic parameters
The sperm kinetic parameters of frozen thawed semen were analyzed using computer assisted semen analyzer (CASA - Sperm Class Analyzer, Microptic, Barcelona, Spain). The conditions set for motility and velocity parameters were: static, <10; slow, >10 to <25; medium, >25 to <50, and rapid, >50 µm/sec; to obtain different sperm kinetic parameters. The semen samples were diluted in PBS (5–8 × 106cells/mL) and 5 µL was placed onto a plain glass slide (Himedia, Mumbai, India) and covered with a cover slip (18x18 mm). Twenty-five consecutive frames were captured under 100× magnification from different homogenous fields and analyzed for sperm kinetic parameters.
Plasmalemma integrity
The plasmalemma integrity in frozen thawed semen was assessed using eosin (5%) - nigrosin (10%) stain. The semen samples were mixed equal parts with stain (1:1) and mixed gently. After 30 s, a drop of stained sample was smeared onto the glass slide. The slides were air dried and observed under 1,000x magnification. The unstained sperm were counted as plasmalemma intact (live sperm) whereas partially or completely stained cells were considered as cells with damaged plasma membrane (dead sperm). A minimum of 200 cells were counted in different fields to obtain the percentage of plasmalemma intact sperm.
Mitochondrial membrane potential
The sperm were stained with JC-1 (5,5′,6,6′-tetrachloro-1,1′,3,3′-tetraethylbenzimidazolocarbocyanine iodide) to analyze the mitochondrial membrane potential as described by [Selvaraju et al. Citation2008]. In brief, 20 µL of semen was added to JC-1 stain (1.53 mM JC-1 in 50 µL PBS) and incubated in dark at 37 °C for 30 min. A drop of semen sample was smeared on a glass slide and observed under a G-2A filter (excitation filter, 450–490 nm; dichroic mirror, 505 nm; barrier filter, 520 nm) in an epifluorescence microscope (Nikon Eclipse 80i, Nikon, Japan). A minimum of 200 sperm were counted at 1,000× magnification. The mitochondria with yellow to orange fluorescence are indicative of the formation of J-aggregates and considered positive for mitochondrial membrane potential. The mitochondria with green fluorescence were considered negative.
Chromatin distribution
The frozen thawed semen (20 µL) was smeared onto a clean glass slide and fixed in neutral buffered formalin for 30 min. The sperm were subjected to mild acid hydrolysis by incubating in 5N HCl for 30 min to expose free aldehyde group in the deoxyribose sugar followed by incubation in Schiff’s reagent for 30 min in darkness. Then the slides were washed thrice in sulfite water (180 mL distilled water plus 10 mL of 10 % sodium metabisulfite and 10 mL of 1N HCl) at room temperature for 2 min each. A minimum of 200 cells were evaluated to assess normal chromatin distribution under 1,000 x magnification. The unevenly stained sperm and sperm with vacuoles and folded head were considered to have abnormal chromatin distribution [Selvaraju et al. Citation2011].
Isolation of sperm protein
The sperm pellets were thawed on ice and subjected to percoll purification (45% percoll in phosphate buffer saline pH-7.4) as described by D'Amours et al. [Citation2010]. Briefly, the sperm samples were overlaid onto the 1 mL of 45% percoll in PBS in 1.5 mL microcentrifuge tubes and centrifuged at 1,250 g for 5 min. Supernatant was discarded and sperm pellet was washed thrice with 1 mL tris calcium chloride buffer [Miller et al. Citation1990] (TC buffer: 39.9 mM tris, 2.6 mM CaCl2 and 1.5 mM NaN3, pH-7.3) by centrifugation at 7,000 g at 4 °C for 5 min. The sperm pellet was resuspended in 1 mL of TC buffer with 0.1 M phenyl methyl sulfonyl fluoride (PMSF) and 0.1% Triton X100. The samples were homogenized by passing through a 20 G needle attached to a 5 mL syringe for 3–4 times. Then the tubes were incubated at 4 °C for 1 h and vortexed for 5 min at every 10 min interval. After incubation, the tubes were centrifuged at 12,000 g at 4 °C for 30 min. After centrifugation, supernatant was retained and protein concentration was estimated by Bradford macro method (MERCK GeNei, Germany). The sperm protein samples were added with 10 µL of protease inhibitor cocktail (Sigma-Aldrich, USA) and stored at −80 °C until proteome analysis. For 2DE analysis, the sperm protein samples were randomly selected (n = 3) from each group and protein profiling was carried out in triplicate.
2DE and western blot analysis
The sperm protein samples from the randomly selected high (n = 3) and low (n = 3) fertile bulls were pooled group wise and processed for 2DE. The protein profile from each animal was compared against each other (3X3) and analyzed for differential expression of proteins between high and low fertile bulls.
The 2DE was carried out each time by including high and low fertile sperm protein samples. The sperm protein (50 µg for 2DE profiling and 60 µg for western blot) was added to rehydration buffer (8 M urea, 2% CHAPS, 50 mM dithiothreitol, 0.2% biolyte ampholyte, 2 M thiourea) and the final volume was made to 125 µL per immobilized pH gradient (IPG) strip. The precast IPG strips (pH 3–10, 7 cm, Bio-Rad, USA) were rehydrated with sample for 12 h in active phase at 20 °C at 50 V (Protean IEF cell, Bio-Rad, USA). Later on, the sperm proteins were electro-focused at 250 V for 30 min, 500 V for 1 h, 1,000 V for 1 h, 2,000 V for 1 h, 4,000 V for 1 h, and 8,000 V until it reached 16,000 VH (volt hour). Then the samples were equilibrated with SDS equilibration buffer (6M urea, 57 mM Tris-HCl, pH 8.8, 29.3% glycerol and 2% SDS) containing 20 mg/ml DTT for 20 min followed by further equilibration in equilibration buffer containing 25 mg/ml iodoacetamide for 25 min at room temperature. Then the IPG strips were rinsed in electrode buffer before proceeding to SDS-PAGE. The equilibrated strips were placed in 12% separating gel and sealed with 1.0% agarose containing 0.05% bromophenol blue. The initial separation was done at 50 V for 15 min followed by 100 V until the dye front reached the bottom of the gel. After electrophoretic separation, the gel was stained with silver nitrate to detect protein spots in gel documentation system using Gene Snap software (Syngene, UK, version 7.09.06, G-BOX ichemi XR). The data acquisition and protein spot analysis were carried out using Dymension 2D Gel Analysis software (Syngene, UK). For western blotting, the gel was equilibrated for 20 min in blotting buffer (25 mM tris, 192 mM glycine and 20% methanol) prior to electro-transfer of protein. The wet blot transfer of proteins to polyvinylidene difluoride membrane (PVDF, Millipore, USA) was performed at 20 V, 70 mA at 4 °C for 3 h. After transfer, membrane was washed with tris buffered saline with tween-20 (TBS-T, 19.97 mM Tris HCl, 136.89 mM NaCl, and 0.1% Tween-20) for 2 min and then incubated in blocking buffer containing 5% bovine serum albumin (BSA, Sigma-Aldrich) at 4 °C for 1 h. The membrane was washed with TBS-T and incubated with binder of sperm homolog 1 (BSPH1) primary antibody (goat polyclonal antibody, 30 ng/mL, Santa Cruz Biotechnology, USA) at 4 °C with constant rocking overnight. After incubation, the membrane was washed with TBS-T thrice within 10 min. Then the membrane was incubated in secondary antibody (donkey anti-goat IgG-HRP conjugate, 500 ng/mL, Santa Cruz Biotechnology, USA) at room temperature (28 °C) for 1 h, followed by three washes (3 min each) in TBS-T. The immunocomplex was detected by using ECL-HRP substrate (SuperSignal West Femto, Thermo Scientific, USA). The membrane was incubated with substrate for 5 min in dark and the signal was captured by chemi-documentation system (iChemi XR, Syngene, UK).
2DE protein spot analysis and data acquisition
The 2DE gels were scanned in gel doc to get gene snap image format. The similar image acquisition protocol was followed for each replicate as recommended [Celis and Gromov Citation2000; O'Farrell et al. Citation1977]. The replicate images were loaded to Dymension 2D gel analysis software (Syngene, UK) to compare the sperm protein spots between high and low fertile groups. The region of interest was defined to analyze total number of spots with in the area specified. The gel intensity was kept constant in all replicate analysis and molecular weight calibration was assigned by using standard molecular marker 10–250 kDa (Bio Rad, USA) in order to calculate the molecular weight of protein spots. The pI calibration was assigned by using IPG 3–10 linear range used for isoelectric focusing of proteins in order to calculate the pI of protein spots. The spot area was normalized by generating master gel after loading replicate images (n = 3) of a particular group and spot detection was carried out. The spot matching was performed between high and low fertile groups using matched spot plot in combined profile matching. The protein spot area was normalized using default parameters of the software and the mean intensities were measured in pixels. The replicate normalized volume ratio was generated to measure the protein spot intensities (protein area) and expressed as protein abundance.
In order to quantify the differentially expressed abundant protein spots (SMP1, SMP2, and SMP3) between groups, three technical replicates were analyzed separately for their normalized volume ratio and their protein quantities were compared. Further isoelectric point based 2DE mapping was generated by considering replicate total protein area in order to determine the protein distribution between high and low fertile bulls. Each replicate data was analyzed separately and for isoelectric point based 2DE-protein map, protein spot abundance as per isoelectric point was averaged from triplicate analysis.
Mass spectrometry (MALDI-TOF)
Based on 2DE analysis, the major protein spots (SMP1, SMP2, and SMP3) observed to be abundant and differing between fertility groups were excised and subjected to MALDI-TOF analysis [Shetty et al. Citation2001]. In brief, the protein spots were cut into 1 mm cubes and destained using 50 µL acetonitril (ACN) and 50 µL 50 mM ammonium bicarbonate. Further the reduction of disulfide linkages by 10 mM dithiothreitol (DTT) and alkylation of sulphahydril groups with 50 mM iodoacetamide (IAA) were carried out. Then the peptides in gel pieces were digested using trypsin and extracted from the gel using 0.1% trifluoroacetic acid (TFA) in ACN. The extracted peptides (1 µL) were mixed with 1 µL of α-cyano-4-hydroxycinnamic acid (HCCA) matrix. The total mixture (2 µL) was loaded to a MALDI target plate and allowed to dry. After drying, the plate with sample was subjected to MALDI-TOF (Bruker, USA). After completion of mass to charge ratio analysis, protein spectrum was retrieved from Flexcontrol software (Shrimpex Biotech Service Pvt. Ltd., Chennai. India).
SDS-PAGE and western blot analysis
Sperm protein samples from randomly selected high (n = 4) and low fertile bulls (n = 4) were separated by SDS-PAGE using 6–16% linear gradient gel [Laemmli Citation1970]. The protein samples (5 µg/well) were denatured at 95 °C for 5 min and loaded into the wells along with prestained standard molecular weight marker 10–250 kDa (Bio Rad). After loading the samples, electrophoresis was initially performed for 15 min at 50 V followed by 100 V until the dye front reaches 3/4th of the gel and western blot analysis was carried out as described earlier for 2DE proteins.
Statistical analysis and bioinformatics
The percent mean values for various sperm attributes were arcsine transformed and subjected to Student t-test to assess the differences between groups. Pearson’s correlation was used to assess the correlation between sperm attributes and CR. The values are expressed as mean ± standard error. The protein abundance between groups was analyzed for significance using Student t-test. For all the analysis p < 0.05 was set as significant. The MALDI-TOF peptide mass results were analyzed through Mascot search engine (www.matrixscience.com). Parameters set were: Swissprot as database, carbamidomethylation was allowed as a fixed modification and oxidation of methionine was allowed as a variable modification, maximum peptide tolerance as 100–400 ppm; missed cleavage up to 2. The identified protein network and interaction was studied using STRING 9.1 online software (www.string-db.org) by keeping confidence level at 0.4 (medium confidence) [Jensen et al. Citation2009]. Bioinformatic analysis of proteins, the identified protein predictive pathway information was obtained from DAVID Bioinformatics Resources 6.7 [Huang da et al. Citation2009]. Gene ontology annotations of PDC-109 network proteins were studied using PANTHER classification system version 10.0 [Mi et al. Citation2013].
Abbreviations | ||
2DE | = | 2D-gel electrophoresis |
2D-DIGE | = | two-dimensional difference gel electrophoresis |
ACN | = | acetonitril |
ACR | = | acrosin |
AI | = | artificial insemination |
APOA1 | = | apolipoprotein A-I |
aSFP | = | acid seminal fluid protein |
BSP | = | binder of sperm protein |
CASA | = | computer assisted semen analyzer |
CatSper3 | = | cation channel sperm-associated protein 3 |
CR | = | conception rate |
DTT | = | dithiotheritol |
HCCA | = | alpha cyano 4 hydroxycinnamic acid |
IAA | = | iodoacetamide |
IPG | = | immobilized pH gradient |
MALDI-TOF | = | matrix assisted laser desorption/ionization-time of flight |
NPC2 | = | epididymal secretory protein E1 precursor |
PB1 | = | sperm-binding fibronectin type II-module protein |
PDC-109 | = | protein with N-terminus aspartic acid, D and carboxy terminus cystine, having 109 aminoacids |
PH-20 | = | sperm adhesion molecule 1 |
SDS-PAGE | = | sodium dodecyl sulfate polyacrylamide gel electrophoresis |
SPADH1 | = | spermadhesin-1 |
SPINK6 | = | serine peptidase inhibitor kazal type 6 |
TC | = | tris-calcium chloride |
TFA | = | trifluoroacetic acid |
ZPBP | = | zona pellucida binding protein |
Supplemental material available online
IAAN_1094837_Supplemental_File.zip
Download Zip (16.3 KB)Acknowledgments
We are thankful to Nandini Sperm Station (NSS), Karnataka Milk Federation, Hessarghatta, Bengaluru, India for providing semen samples and conception rate data for the bulls.
Declaration of interest
LS is funded by an Innovation in Science Pursuit for Inspired Research (INSPIRE) fellowship by Department of Science and Technology, Ministry of Science and Technology, Government of India for pursuing Ph.D. programme at the Department of Biochemistry, Jain University, Bengaluru, Karnataka, India. The research work is supported by Indian Council of Agricultural Research-National Institute of Animal Nutrition and Physiology, Adugodi, Bengaluru, India. The authors declare that there is no conflict of interest that could be perceived as prejudicing the impartiality of the research reported. The authors alone are responsible for the content and writing of manuscript.
Author contribution
Conceived and designed the experiments: LS, SS, JPR; Performed the experiments: LS; Analyzed the data: LS, SS, PS; Wrote the manuscript: LS, SS, JPR; Revised the manuscript: LS, SS, PS, JPR. All authors approved the manuscript.
References
- Amaral, A., Castillo, J., Ramalho-Santos, J. and Oliva, R. (2014) The combined human sperm proteome: Cellular pathways and implications for basic and clinical science. Hum Reprod Update 20:40–62
- Arangasamy, A., Singh, L.P., Ahmed, N., Ansari, M.R. and Ram, G.C. (2005) Isolation and characterization of heparin and gelatin binding buffalo seminal plasma proteins and their effect on cauda epididymal spermatozoa. Anim Reprod Sci 90:243–254
- Azpiazu, R., Amaral, A., Castillo, J., Estanyol, J.M., Guimera, M., Ballesca, J.L., et al. (2014) High-throughput sperm differential proteomics suggests that epigenetic alterations contribute to failed assisted reproduction. Hum Reprod 29:1225–1237
- Boryshpolets, S., Kowalski, R.K., Dietrich, G.J., Dzyuba, B. and Ciereszko, A. (2013) Different computer-assisted sperm analysis (CASA) systems highly influence sperm motility parameters. Theriogenology 80:758–765
- Bromfield, J.J., Schjenken, J.E., Chin, P.Y., Care, A.S., Jasper, M.J. and Robertson, S.A. (2014) Maternal tract factors contribute to paternal seminal fluid impact on metabolic phenotype in offspring. Proc Natl Acad Sci USA 111:2200–2205
- Celis, J.E. and Gromov, P. (2000) High-resolution two-dimensional gel electrophoresis and protein identification using western blotting and ECL detection. EXS 88:55–67
- D'Amours, O., Frenette, G., Fortier, M., Leclerc, P. and Sullivan, R. (2010) Proteomic comparison of detergent-extracted sperm proteins from bulls with different fertility indexes. Reproduction 139:545–556
- De Canio, M., Soggiu, A., Piras, C., Bonizzi, L., Galli, A., Urbani, A., et al. (2014) Differential protein profile in sexed bovine semen: Shotgun proteomics investigation. Mol Biosyst 10:1264–1271
- Dostalova, Z., Calvete, J.J., Sanz, L., Hettel, C., Riedel, D., Schoneck, C., et al. (1994) Immunolocalization and quantitation of acidic seminal fluid protein (aSFP) in ejaculated, swim-up, and capacitated bull spermatozoa. Biol Chem Hoppe Seyler 375:457–461
- Fiol de Cuneo, M., Vincenti, L.M., Martini, A.C., Ponce, A.A. and Ruiz, R.D. (2004) Effects of PDC-109 on bovine sperm functional activity in presence or absence of heparin. Theriogenology 62:207–216
- Gwathmey, T.M., Ignotz, G.G. and Suarez, S.S. (2003) PDC-109 (BSP-A1/A2) promotes bull sperm binding to oviductal epithelium in vitro and may be involved in forming the oviductal sperm reservoir. Biol Reprod 69:809–815
- Huang da, W., Sherman, B.T. and Lempicki, R.A. (2009) Systematic and integrative analysis of large gene lists using DAVID bioinformatics resources. Nat Protoc 4:44–57
- Hung, P.H. and Suarez, S.S. (2012) Alterations to the bull sperm surface proteins that bind sperm to oviductal epithelium. Biol Reprod 87:88
- Infante, R.E., Wang, M.L., Radhakrishnan, A., Kwon, H.J., Brown, M.S. and Goldstein, J.L. (2008) NPC2 facilitates bidirectional transfer of cholesterol between NPC1 and lipid bilayers, a step in cholesterol egress from lysosomes. Proc Natl Acad Sci USA 105:15287–15292
- Jensen, L.J., Kuhn, M., Stark, M., Chaffron, S., Creevey, C., Muller, J., et al. (2009) STRING 8–a global view on proteins and their functional interactions in 630 organisms. Nucleic Acids Res 37:D412–416
- Jin, J., Jin, N., Zheng, H., Ro, S., Tafolla, D., Sanders, K.M., et al. (2007) Catsper3 and Catsper4 are essential for sperm hyperactivated motility and male fertility in the mouse. Biol Reprod 77:37–44
- Jobim, M.I., Oberst, E.R., Salbego, C.G., Souza, D.O., Wald, V.B., Tramontina, F., et al. (2004) Two-dimensional polyacrylamide gel electrophoresis of bovine seminal plasma proteins and their relation with semen freezability. Theriogenology 61:255–266
- Kim, H.J., Choi, M.Y., Kim, H.J. and Llinas, M. (2010) Conformational dynamics and ligand binding in the multi-domain protein PDC109. PLoS One 5:e9180
- Kovac, J.R., Pastuszak, A.W. and Lamb, D.J. (2013) The use of genomics, proteomics, and metabolomics in identifying biomarkers of male infertility. Fertil Steril 99:998–1007
- Kwon, W.S., Rahman, M.S., Lee, J.S., Kim, J., Yoon, S.J., Park, Y.J., et al. (2014) A comprehensive proteomic approach to identifying capacitation related proteins in boar spermatozoa. BMC Genomics 15:897
- Laemmli, U.K. (1970) Cleavage of structural proteins during the assembly of the head of bacteriophage T4. Nature 227:680–685
- Liou, H.L., Dixit, S.S., Xu, S., Tint, G.S., Stock, A.M. and Lobel, P. (2006) NPC2, the protein deficient in Niemann-Pick C2 disease, consists of multiple glycoforms that bind a variety of sterols. J Biol Chem 281:36710–36723
- Liu, X., Jin, S.H., Liu, X.X., Wang, W.J. and Liu, F.J. (2014) Proteome profiling of the sperm maturation milieu in the rhesus monkey (Macaca mulatta) epididymis. Reprod Fertil Dev. doi: 10.1071/RD14322
- Lusignan, M.F., Bergeron, A., Crete, M.H., Lazure, C. and Manjunath, P. (2007) Induction of epididymal boar sperm capacitation by pB1 and BSP-A1/-A2 proteins, members of the BSP protein family. Biol Reprod 76:424–432
- Manjunath, P., Chandonnet, L., Leblond, E. and Desnoyers, L. (1994) Major proteins of bovine seminal vesicles bind to spermatozoa. Biol Reprod 50:27–37
- Manjunath, P. and Therien, I. (2002) Role of seminal plasma phospholipid-binding proteins in sperm membrane lipid modification that occurs during capacitation. J Reprod Immunol 53:109–119
- Martin-DeLeon, P.A. (2006) Epididymal SPAM1 and its impact on sperm function. Mol Cell Endocrinol 250:114–121
- Mi, H., Muruganujan, A., Casagrande, J.T. and Thomas, P.D. (2013) Large-scale gene function analysis with the PANTHER classification system. Nat Protoc 8:1551–1566
- Miller, D.J., Winer, M.A. and Ax, R.L. (1990) Heparin-binding proteins from seminal plasma bind to bovine spermatozoa and modulate capacitation by heparin. Biol Reprod 42:899–915
- O'Farrell, P.Z., Goodman, H.M. and O'Farrell, P.H. (1977) High resolution two-dimensional electrophoresis of basic as well as acidic proteins. Cell 12:1133–1141
- O'Leary, S., Jasper, M.J., Warnes, G.M., Armstrong, D.T. and Robertson, S.A. (2004) Seminal plasma regulates endometrial cytokine expression, leukocyte recruitment and embryo development in the pig. Reproduction 128:237–247
- Oehninger, S., Franken, D.R., Sayed, E., Barroso, G. and Kolm, P. (2000) Sperm function assays and their predictive value for fertilization outcome in IVF therapy: A meta-analysis. Hum Reprod Update 6:160–168
- Oliva, R., De Mateo, S., Castillo, J., Azpiazu, R., Oriola, J. and Ballesca, J.L. (2010) Methodological advances in sperm proteomics. Hum Fertil (Camb) 13:263–267
- Park, Y.J., Kim, J., You, Y.A. and Pang, M.G. (2013) Proteomic revolution to improve tools for evaluating male fertility in animals. J Proteome Res 12:4738–4747
- Park, Y.J., Kwon, W.S., Oh, S.A. and Pang, M.G. (2012) Fertility-related proteomic profiling bull spermatozoa separated by percoll. J Proteome Res 11:4162–4168
- Peddinti, D., Nanduri, B., Kaya, A., Feugang, J.M., Burgess, S.C. and Memili, E. (2008) Comprehensive proteomic analysis of bovine spermatozoa of varying fertility rates and identification of biomarkers associated with fertility. BMC Syst Biol 2:19
- Pixton, K.L., Deeks, E.D., Flesch, F.M., Moseley, F.L., Bjorndahl, L., Ashton, P.R., et al. (2004) Sperm proteome mapping of a patient who experienced failed fertilization at IVF reveals altered expression of at least 20 proteins compared with fertile donors: Case report. Hum Reprod 19:1438–1447
- Rahman, M.S., Lee, J.S., Kwon, W.S. and Pang, M.G. (2013) Sperm proteomics: Road to male fertility and contraception. Int J Endocrinol 2013:360986
- Rego, J.P., Crisp, J.M., Moura, A.A., Nouwens, A.S., Li, Y., Venus, B., et al. (2014) Seminal plasma proteome of electroejaculated Bos indicus bulls. Anim Reprod Sci 148:1–17
- Rodriguez-Martinez, H., Kvist, U., Ernerudh, J., Sanz, L. and Calvete, J.J. (2011) Seminal plasma proteins: What role do they play? Am J Reprod Immunol 66(Suppl 1):11–22
- Rodriguez-Martinez, H. (2013) Semen evaluation techniques and their relationship with fertility. Anim Reprod 10:148–159
- Roncoletta, M., Morani Eda, S., Esper, C.R., Barnabe, V.H. and Franceschini, P.H. (2006) Fertility-associated proteins in Nelore bull sperm membranes. Anim Reprod Sci 91:77–87
- Selvaraju, S., Nandi, S., Gupta, P.S. and Ravindra, J.P. (2011) Effects of heavy metals and pesticides on buffalo (Bubalus bubalis) spermatozoa functions in vitro. Reprod Domest Anim 46:807–813
- Selvaraju, S., Ravindra, J.P., Ghosh, J., Gupta, P.S. and Suresh, K.P. (2008) Evaluation of sperm functional attributes in relation to in vitro sperm-zona pellucida binding ability and cleavage rate in assessing frozen thawed buffalo (Bubalus bubalis) semen quality. Anim Reprod Sci 106:311–321
- Shetty, J., Diekman, A.B., Jayes, F.C., Sherman, N.E., Naaby-Hansen, S., Flickinger, C.J., et al. (2001) Differential extraction and enrichment of human sperm surface proteins in a proteome: Identification of immunocontraceptive candidates. Electrophoresis 22:3053–3066
- Singh, A.P. and Rajender, S. (2014) CatSper channel, sperm function and male fertility. Reprod Biomed Online
- Singh, M., Ghosh, S.K., Prasad, J.K., Kumar, A., Tripathi, R.P., Bhure, S.K., et al. (2014) Seminal PDC-109 protein vis-a-vis cholesterol content and freezability of buffalo spermatozoa. Anim Reprod Sci 144:22–29
- Soggiu, A., Piras, C., Hussein, H.A., De Canio, M., Gaviraghi, A., Galli, A., et al. (2013) Unravelling the bull fertility proteome. Mol Biosyst 9:1188–1195
- Souza, C.E., Moura, A.A., Monaco, E. and Killian, G.J. (2008) Binding patterns of bovine seminal plasma proteins A1/A2, 30 kDa and osteopontin on ejaculated sperm before and after incubation with isthmic and ampullary oviductal fluid. Anim Reprod Sci 105:72–89
- Srivastava, N., Jerome, A., Srivastava, S.K., Ghosh, S.K. and Kumar, A. (2013) Bovine seminal PDC-109 protein: An overview of biochemical and functional properties. Anim Reprod Sci 138:1–13
- Srivastava, N., Srivastava, S.K., Ghosh, S.K., Singh, L.P., Prasad, J.K., Kumar, A., et al. (2012) Sequestration of PDC-109 protein improves freezability of crossbred bull spermatozoa. Anim Reprod Sci 131:54–62
- Therien, I., Moreau, R. and Manjunath, P. (1999) Bovine seminal plasma phospholipid-binding proteins stimulate phospholipid efflux from epididymal sperm. Biol Reprod 61:590–598
- Thomas, C.J., Anbazhagan, V., Ramakrishnan, M., Sultan, N., Surolia, I. and Swamy, M.J. (2003) Mechanism of membrane binding by the bovine seminal plasma protein, PDC-109: A surface plasmon resonance study. Biophys J 84:3037–3044
- Tripathi, U.K., Aslam, M.K., Pandey, S., Nayak, S., Chhillar, S., Srinivasan, A., et al. (2014) Differential proteomic profile of spermatogenic and Sertoli cells from peri-pubertal testes of three different bovine breeds. Front Cell Dev Biol 2:24
- Vasan, S.S. (2011) Semen analysis and sperm function tests: How much to test? Indian J Urol 27:41–48
- Wah, D.A., Fernandez-Tornero, C., Sanz, L., Romero, A. and Calvete, J.J. (2002) Sperm coating mechanism from the 1.8 A crystal structure of PDC-109-phosphorylcholine complex. Structure 10:505–514
- Zhou, C., Kang, W. and Baba, T. (2012) Functional characterization of double-knockout mouse sperm lacking SPAM1 and ACR or SPAM1 and PRSS21 in fertilization. J Reprod Dev 58:330–337