Abstract
Zinc oxide nanoparticles-(ZnONPs)modified carbon paste enzyme electrodes (ZnONPsMCPE) were developed for determination of glucose. The determination of glucose was carried out by oxidation of H2O2 at +0.4 V. ZnONPsMCPE provided biocompatible microenvironment for GOx and necessary pathway of electron transfer between GOx and electrode. The response of GOx/ZnONPsMCPE was proportional to glucose concentration and detection limit was 9.1 × 10–3 mM. Km and Imax, were calculated as 0.124 mM and 2.033 μA. The developed biosensor exhibits high analytical performance with wide linear range (9.1 × 10–3–14.5 mM), selectivity and reproducibility. Serum glucose results allow us to ascertain practical utility of GOx/ZnONPsMCPE biosensor.
Introduction
The measurement of glucose is very important in many fields such as biological analysis, environment, food industries, and clinical biochemistry especially for diagnosis of Diabetes Mellitus (DM) (Mizutani and Yabuki Citation1997, Wang Citation1999, Yoshimura and Hozumi Citation1996). DM, chronic and persistent carbohydrate, protein, and lipid metabolism disorder characterized with hyperglycemia may occur in childhood or in adults. The disease is associated with long-term damage, dysfunction, and failure of several organs especially eyes, kidneys, nerves, brain, and heart (Ramachandran et al. Citation2008). Thus, early diagnosis of diabetes can provide better management of the disease. For this reason, blood glucose levels should be determined by using very sensitive and easier methods. Many methods have been developed for determination of glucose such as chemiluminescence (Zhu et al. Citation2002), colorimetric (Wang et al. Citation2009a), electrochemical (Ye et al. Citation2004, Zhao et al. Citation2007, Comba et al. Citation2010), and other methods (Shafer-Peltier et al. Citation2002). In all these methods, electrochemical biosensors especially enzyme-based amperometric glucose biosensors are more commonly investigated due to their simplicity, high accuracy, fast response, storage stability, miniaturization, and low cost (Wang Citation2007, Sadik et al. Citation2009).
In amperometric glucose biosensors, glucose oxidase (GOx) catalyzes the direct electron transfer from2 glucose to oxygen to produce glucanolactone and hydrogen peroxide. An enzyme electrode for glucose determination is based on the detection of enzymatically generated hydrogen peroxide during the enzymatic reaction (Wang et al. Citation2007, Deyhimi and Salamat-Ahangari Citation2003).
The glucose can be determined by monitoring the oxidation current of liberated hydrogen peroxide at constant potential (Moreno-Bondi et al. Citation1990).
The oxidation of hydrogen peroxide can be achieved at high potentials. These high potentials can cause the oxidation of other electroactive compounds such as uric acid and ascorbic acid present in real samples. For this reason, redox mediators have been used in order to decrease the overpotential. In addition to different electron transfer mediators (Erden et al. Citation2012), nanostructured materials including carbon nanotubes (Rakhi et al. Citation2009), polymers (Sirkar et al. Citation2000), electrospun nanofibers (Manesh et al. Citation2008), silanes (Luong et al. Citation2004) and metals such as gold (Liu and Ju Citation2003, Anik et al. Citation2012), platinum (Zhou et al. Citation2005), nickel (Salimi et al. Citation2007), and copper (Zhao et al. Citation2006) have been used to construct biosensors. Besides metal nanostructures, metal oxides such as iron oxide (Kaushik et al. Citation2008), NiFe2O4 (Luo et al. Citation2010), zirconium oxide (Zhao et al. Citation2005), and tin oxide-chitosan nanobiocomposite (Ansari et al. Citation2009) have been widely used to modify electrodes and facilitate the electron transfer between the electrode surface and enzyme's active sites (Zhang et al. Citation2004) in the last few years. They could keep the enzyme activity due to the covetable microenvironment (Wang et al. Citation2009b). These nanostructures have been widely used in optics, optoelectronics, electrics, and biosensors owing to its unique properties such as high specific surface area and nontoxicity (Wang Citation2005, Pumera et al. 2007).
Over the last decade, ZnONPs have been applied in oxidase-based amperometric biosensors due to excellent properties such as biocompatibility, chemical stability, and high electrocatalytic activity (Tian et al. Citation2002, Zang et al. Citation2007, Sun et al. Citation2008). In addition, ZnONPs provide friendly microenvironment for physical adsorption with high isoelectric point (pI 9.5) especially for acidic protein molecules such as glucose oxidase with low pI (4.2–4.5) (Saha et al. Citation2009).
Up to now, many studies on different ZnO nanostructures for determination of hydrogen peroxide (Gu et al. Citation2009), cholesterol (Umar et al. Citation2009), phenolic compounds (Zhao et al. Citation2009), hemoglobin (Ma and Tian Citation2010), and glucose (Ren et al. Citation2009, Xiao et al. Citation2009) have been reported. Recently, several glucose biosensor based on ZnO nanorods (Liu et al. Citation2009), Co-doped ZnO nanoclusters (Zhao et al. Citation2007), tetragonal pyramid-shaped porous ZnO (Dai et al. Citation2009), ZnO nanotubes (Kong et al. Citation2009), ZnO nanoflake (Fulati et al. Citation2010), ZnO nanowires (Usman Ali et al. Citation2010), ZnO hollow nanospheres (Meng et al. Citation2011), and ZnO nanoparticles (Ren et al. Citation2009) have been developed.
Carbon paste electrode (CPE), a mixture of graphite powder and linker (e.g paraffin/mineral oil), has been used as a matrice since 1958 by Adams for various analytical applications. Due to its wide anodic potential range, chemical stability, ease of modification, and low cost, preparation of modified carbon paste electrodes with new materials is becoming popular in electrochemical researches (Gilmartin and Hart Citation1995, Švancara et al. Citation2009, Miscoria et al. Citation2005, Koyuncu et al. Citation2007).
In this study, a simple and cost effective glucose biosensor was developed at low potential to eliminate effects of interferences. The parameters affecting the sensitivity of enzyme electrode were studied. Glucose analysis in serum samples was also performed using developed enzyme electrode. The utility of ZnONPs with carbon paste electrodes for amperometric detection was performed for the first time. In this method, the electrocatalytic properties of ZnONPs towards the oxidation of H2O2 via cyclic voltammetry technique were examined.
Experimental
Materials
Glucose oxidase (GOx) (from Aspergillus niger, EC 1.1.3.4 39680 Units/g solid) and zinc oxide (ZnO) nanoparticles (< 100 nm) were from Sigma. Uric acid (UA), ascorbic acid, creatinine, urea, glucose, glutaraldeyde, and bovine serum albumine were also purchased from Sigma. Graphite powder and parafin oil were obtained from Fluka. Hydrogen peroxide (35%, v/v aqueous solution), di-sodium monohydrogen phosphate heptahydrate, and sodium dihydrogen phosphate dihydrate were acquired from Riedel de Haën. Ultrapure water from Purelab Elga system was used for preparing all solutions. Glucose stock solutions were allowed to mutarotate at room temperature overnight before use. Measurements were carried out in a phosphate buffer (0.1 M, pH 7.0) supporting electrolyte. All other chemicals were of analytical grade.
Apparatus and measurements
All electrochemical studies were performed on IVIUMSTAT electrochemical analyzer (Ivium Techonologies, Netherlands) using a three-electrode cell stand (Bioanalytical Systems, Inc., USA). Bare and modified carbon paste electrodes were used as working electrodes. The counter electrode and reference electrode were a platinum wire (BAS MW 1034) and Ag/AgCl, 3 M KCl (BAS MF 2052), respectively. All potential values given below refer to Ag/AgCl. The pH values of the buffer solutions were measured by using ORION Model 1906 D. 720A pH/ion meter (Thermo Scientific USA). Temperature control was achieved with Grant LTD GG thermostat (Grant Instruments, UK). Amperometric measurements were carried out in a phosphate buffer (0.1 M pH 7.0, 5 ml) under stirred condition. The response current was obtained with the difference between the steady-state current and background current. All measurements were carried out at room temperature (23 ± 2°C).
Preparation of carbon paste electrodes
For bare carbon paste electrode (BCPE) construction, carbon paste was prepared by mechanically hand mixing graphite powder (70.0% w/w) and paraffin oil (30.0% w/w) until homogeneous paste was obtained. ZnONPs modified CPEs were prepared by mixing ZnONPs with graphite powder in a ratio of 3:1 (w/w), followed by the incorporation of paraffin oil, and mixed regularly. In the case of the enzyme electrode, enzyme solution containing various units of GOx, 10 μL of glutaraldehyde (1.25% (v/v)) and 1.5 mg BSA was added into graphite powder-ZnONPs mixture. Finally, carbon paste was obtained by mixing with 15 μL paraffin oil for 20 min. A portion of the given pastes was placed firmly into a cavity of polyetheretherketone (PEEK) rod electrode bodies (with 3 mm internal diameter) and the surfaces of obtained electrodes were smoothed on a weighing paper before starting new experiment. Amperometric determination of glucose was performed by the oxidation of enzymatically produced hydrogen peroxide at +0.4 V versus Ag/AgCl reference electrode. To find the optimum composition, calibration graphs were plotted for each electrode and slopes of the calibration graphs (sensitivity) were compared. The obtained electrodes were stored in the refrigerator at +4°C when not in use.
Results and discussion
Electrochemical characterization of bare and ZnONPs-modified electrodes
The electrocatalytic activities of bare and ZnONPs-modified carbon paste electrodes (ZnONPsMCPE) for hydrogen peroxide oxidation were studied in the presence and absence of 10 mM hydrogen peroxide by cyclic voltammetry. The determination of H2O2, a product of oxidase-based enzymatic reactions, is very important due to its easy oxidation or reduction. Cyclic voltammograms of BCPE and ZnONPsMCPEs were shown in in the absence H2O2 (a, c) and in the presence H2O2 (b, d) in the potential range between − 0.3 V–+ 1.0 V at scan rate 50 mV s− 1. As can be seen from CVs, the current response increment is observed due to hydrogen peroxide oxidation at ZnONPsMCPE. In addition, the ZnONPsMCPE show significant oxidation of hydrogen peroxide starting around +0.4 V (vs. Ag/AgCl). In contrast, the oxidation of H2O2 at bare carbon paste electrode begins after + 0.7 V. The ZnONPsMCPE provide an important decrease in working potential for determination of H2O2. Thus, the determination of glucose in serum can be achieved without any interference effects at low potential.
Optimization of the biosensor
The sensitivities of the enzyme electrodes were determined at different GOx concentrations (4–12 U). The enzyme loading dependency on biosensor sensitivity was investigated by measuring the different GOx concentrations (4–12 U) loaded biosensors responses to sequential glucose increments from 0.02 to 0.20 mM. Other parameters were kept constant at + 0.4 V. As expected from the increased biocatalytic activity of the electrode, sensitivity was enhanced with the increasing of the amount of enzyme in the paste. Although the maximum sensitivity was obtained at 12 U glucose oxidase loading, 10 U was selected due to negligible increment ().
Figure 2. Effect of GOx unit on sensitivity of ZnONPs modified carbon paste enzyme electrode (0.1 M pH 7.0 PBS at + 0.4 V vs. Ag/AgCl).
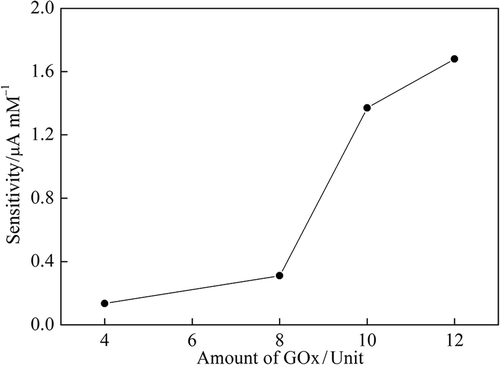
The glucose enzyme electrode sensitivity variation due to pH was obtained by measuring the electrode responses to sequential glucose increments from 0.02 to 0.20 mM at the desired pH in a stirred buffer (6.0–9.0). The maximum sensitivity was obtained at pH 7.0 (). The same pH result was also found in related studies (Tian et al. Citation2002, Saha et al. Citation2009, Dai et al. Citation2009). Different pH values for glucose biosensors were also reported (Usman Ali et al. Citation2010, Wei et al. Citation2010, Lei et al. Citation2011). This was attributed to the amount of ZnONPs, enzyme loading, and electrode preparation procedures.
Figure 3. Effect of pH value on sensitivity of ZnONPs modified carbon paste enzyme electrode (0.1 M pH 7.0 PBS at + 0.4 V vs. Ag/AgCl).
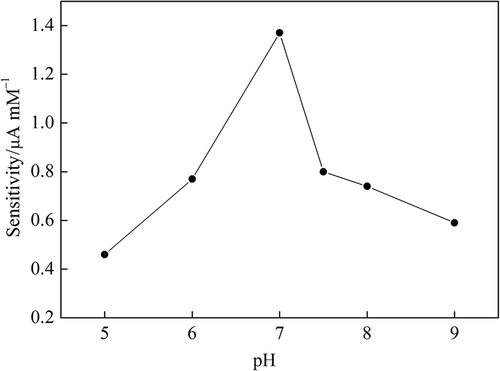
Temperature dependence of glucose enzyme electrode sensitivity was obtained by measuring the electrode responses to sequential glucose increments from 0.02 to 0.20 mM maintained at the desired temperature (10–50°C). As illustrated in , the sensitivity of the biosensor increased with increasing temperature until 25°C, and then declined slowly. The increment in the response of sensors could be affected by temperature increases due to enhanced enzymatic activity, increased membrane permeability of various participating species, and decrease in dissolved O2 concentration (Vaddiraju et al. Citation2011). The decline in the response of sensors could be due to denaturation of GOx. Further experiments were performed at 25°C.
The effect of glucose concentration
In order to determine the linear working range of the glucose biosensor, the amperometric response recorded as a function of glucose concentration. As presented in (inset A and B) two linear working ranges were obtained. The inset in shows a good linear response in the range of 9.1 × 10–3 mM–0.16 mM glucose with a correlation coefficient of 0.9800. The regression equation is I(μA) = 1.73Cglucose + 1.29. Also good linear response in the range of 1.0 mM–14.5 mM glucose with a correlation coefficient of 0.9853 was obtained (). The regression equation is I(μA) = 0.026Cglucose + 1.72. Relatively low detection limit (9.1 × 10–3 mM) was obtained. The linear range covers the normal serum glucose level (4.4–6.6 mM) (Wang Citation2007).
Figure 5. Effect of glucose concentration on amperometric response of ZnONPs modified carbon paste enzyme electrode, inset: linear working ranges plots (0.1 M pH 7.0 PBS at + 0.4 V vs. Ag/AgCl).
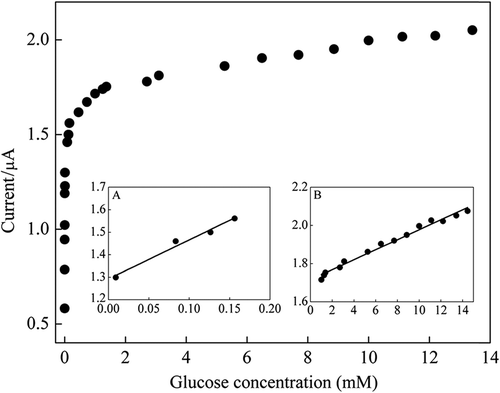
Km gives an indication of the enzyme–substrate kinetics for the glucose biosensor. Kinetic parameters, such as Km and Imax, are usually calculated from the well-known Lineweaver–Burk plot by plotting “1/current response vs. 1/substrate concentration”. Km and Imax for developed biosensor were determined using Hanes–Woolf plot due to not linearity of Lineweaver–Burk plot. Kinetic parameters were obtained from the Hanes–Woolf plot by plotting “substrate concentration/current response vs. substrate concentration” (). Km and Imax were calculated as 0.124 mM and 2.033 μA, respectively, using following equation (Garrett and Grisham Citation2009).
Figure 6. Substrate concentration/current response vs. substrate concentration plot (Hanes-Woolf plot).
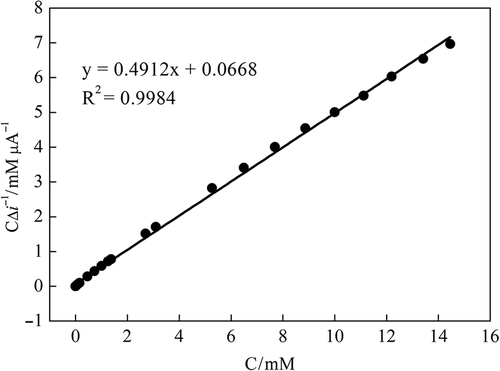
The Km in the present work is lower than GOx immobilized on Co-doped ZnO nanoclusters (21 mM) (Zhao et al. Citation2007), ZnO nanotubes (19 mM) (Kong et al. Citation2009), and ZnO nanorod via citric acid-assisted annealing route (1.95 mM) (Yang et al. Citation2010).
Repeatability, reproducibility, and response time
The sensitivities of same glucose enzyme electrode were obtained five times consecutively at + 0.4 V (vs. Ag/AgCl) to evaluate of the repeatability of the enzyme electrode. The relative standard deviation of the obtained sensitivities was found as 3.307%. This result was the indication of good stability. The reproducibility of enzyme electrode was also investigated by measuring the biosensor responses to sequential glucose increments from 0.02 to 0.20 mM in the same day. Four different enzyme electrodes, constructed independently, had a relative standard deviation of 4.273% (). These results indicate that the repeatability and reproducibility of the enzyme electrode were highly satisfactory and obtained electrode can be used in many analyses. The response time is important for the biosensor applications. The response time of ZnONPs modified carbon paste enzyme electrode which defines as the time it takes for the electrode to reach 95% of the steady-state current. The response time was found as 20 s.
Table I. Reusability and reproducibility for ZnONPs modified carbon paste enzyme electrode.
Storage stability
The storage stability of the enzyme electrode was investigated by measuring the biosensor responses to sequential glucose increments from 0.02 to 0.20 mM in a period of 150 days (). The optimized biosensor was retained about 85%, 60%, 45%, 40% of its response after 1 week, 3 weeks, 3 months, and 5 months, respectively. Good long-term stability can be attributed to the mild immobilization procedure and a beneficial environment for preventing enzyme leakage. Immobilization of GOx into carbon paste provides a biocompatible microenvironment around the enzyme, maintaining its biological activity.
Effects of interferences
The real sample contains other common species that could potentially interfere with the glucose detection. In the light of low concentrations of these ascorbic acid, creatinine, urea, uric acid in the blood, the interference needs to be seriously considered because they could be easily oxidized at a relatively high over potential, and a lower working potential helps to avoid the electrochemical reaction of the interferences.
The selectivity of developed biosensor was evaluated in the presence of common interfering species such as ascorbic acid (0.1 mM), creatinine (0.1 mM), urea (0.8 mM), and uric acid (0.05 mM) in oxygenated PBS. The percentages of interference were determined in terms of the difference in percentage of response between the buffer solution containing glucose and buffer solution containing interferences and glucose. The biosensor shows quick response for each addition of 0.1 mM glucose. However, as can be seen from no noteworthy response was observed for the addition of creatinine, urea, and uric acid into buffer solution containing 0.1 mM glucose at + 0.4 V (vs. Ag/AgCl). Although the ascorbic acid caused a small increase in the current, these results can be overseen for the determination of glucose in serum samples due to maximum concentration of L-ascorbic acid in human body is about 0.1 mM (Xiao et al. Citation2000). The results of interference study were shown in . Thus, the developed biosensor is highly selective for glucose and can be applied for glucose determination in human serum samples under physiological conditions.
Table II. Effects of interferences on glucose response.
Determination of glucose in serum
Human serum samples were assayed in order to show the practical utility of this glucose biosensor. Serum samples were provided by a local hospital, which were firstly analyzed with Beckmann Coulter Olympus AU2700 analyzer which is based on hexokinase method. Then serum samples of 10 μL were successively added into 5 mL phosphate buffered (0.1 M pH 7.0), and the response of the developed biosensor was obtained at 0.4 V. The accuracy of the developed biosensor was checked by t-test. The t value was found as 0.408 at 95% confidence level, for tcritic 4.30. As shown in , good agreement between the two methods results allows us to ascertain the practical utility of the developed biosensor at a confidence level of 95%.
Table III. The determination of glucose levels in human serum samples.
The developed biosensor have demonstrated better performance in terms of working range, detection limit and Km compared to other studies based on various synthesized ZnO nanostructures. ZnO nanostructures created a biocompatible environment for GOx, the obtained biosensor has higher storage stability compare to other modified electrodes. Moreover, real sample application for the determination of glucose couldn't find in most of developed biosensors (Saha et al. Citation2009, Ren et al. Citation2009, Kong et al. Citation2009, Yang et al. Citation2010).
Conclusion
The development of highly sensitive electrochemical glucose oxidase biosensor for the determination of glucose was performed by immobilization of GOx within a ZnONPsMCPE. The ZnONPs modified carbon paste provided a biocompatible microenvironment for GOx and a necessary pathway of electron transfer between GOx and the electrode. ZnO nanoparticles tend to act like nanoscale electrodes. The response of the GOx biosensor was proportional to glucose concentration and the detection limit was 9.1 × 10–3 mM. Kinetic parameters, Km and Imax, were calculated as 0.124 mM and 2.033 μA, respectively. The developed biosensor exhibits high analytical performance with a wide linear range (from 9.1 × 10–3 to 14.5 mM), high sensitivity, selectivity, reproducibility, reusability, and storage stability. The serum glucose results allow us to ascertain the practical utility of the ZnONPs-modified GOx biosensor at a confidence level of 95%.
Acknowledgement
Financial support of this study from The Scientific and Technological Research Council of Turkey is gratefully acknowledged.
Declaration of interest
The authors report no conflicts of interest. The authors alone are responsible for the content and writing of the paper.
References
- Anik U, Cubukcu M, Yavuz Y. 2012. Nanomaterial-based composite biosensor for glucose detection in alcoholic beverages. Artif Cells Blood Substit Immobil Biotechnol.Epub ahead of print.
- Ansari AA, Kaushik A, Solanki PR, Malhotra BD. 2009. Electrochemical cholesterol sensor based on tin oxide-chitosan nanobiocomposite film. Electroanalysis.21:965–972.
- Comba FN, Rubianes MD, Cabrera L, Gutiérrez S, Herrasti P, Rivas GA. 2010. Highly sensitive and selective glucose biosensing at carbon paste electrodes modified with electrogenerated magnetite nanoparticles and glucose oxidase. Electroanalysis.22:1566–1572.
- Dai Z, Shao G, Hong J, Bao J, Shen J. 2009. Immobilization and direct electrochemistry of glucose oxidase on a tetragonal pyramid-shaped porous ZnO nanostructure for a glucose biosensor. Biosens Bioelectron.24:1286–1291.
- Deyhimi F, Salamat-Ahangari R. 2003. An initial-rate potentiometric method for the determination of uric acid using a fluoride ion-selective electrode. Talanta.61:493–499.
- Erden PE, Zeybek B, Pekyardimci S, Kilic E. 2012. Amperometric carbon paste enzyme electrodes with Fe(3)O(4) nanoparticles and 1,4-Benzoquinone for glucose determination. Artif Cells Blood Substit Immobil Biotechnol.Epub ahead of print.
- Fulati A, Ali SMU, Asif MH, Alvi NUH, Willander M, Brännmark C, et al. 2010. An intracellular glucose biosensor based on nanoflake ZnO. Sensor Actuator B-Chem.150:673–680.
- Garrett RH, Grisham CM. 2009. Biochemistry. Boston: Brooks/Cole Cengage Learning.
- Gilmartin MAT, Hart JP. 1995. Sensing with chemically and biologically modified carbon electrodes. A review. Analyst.120:1029–1045.
- Gu BX, Xu CX, Zhu GP, Liu SQ, Chen LY, Wang ML, Zhu JJ. 2009. Layer by layer immobilized horseradish peroxidase on zinc oxide nanorods for biosensing. J Physical Chem B.113:6553–6557.
- Kaushik A, Khan R, Solanki PR, Pandey P, Alam J, Ahmad S, Malhotra BD. 2008. Iron oxide nanoparticles–chitosan composite based glucose biosensor. Biosens Bioelectron.24:676–683.
- Kong T, Chen Y, Ye Y, Zhang K, Wang Z, Wang X. 2009. An amperometric glucose biosensor based on the immobilization of glucose oxidase on the ZnO nanotubes. Sensor Actuator B-Chem.138:344–350.
- Koyuncu D, Erden PE, Pekyardimc Ş, Kılıç E. 2007. A new amperometric carbon paste enzyme electrode for ethanol determination. Anal Lett.40:1904–1922.
- Lei Y, Yan X, Zhao J, Liu X, Song Y, Luo N, Zhang Y. 2011. Improved glucose electrochemical biosensor by appropriate immobilization of nano-ZnO. Colloids Surf B Biointerfaces.82:168–172.
- Liu S, Ju H. 2003. Reagentless glucose biosensor based on direct electron transfer of glucose oxidase immobilized on colloidal gold modified carbon paste electrode. Biosens Bioelectron.19:177–183.
- Liu X, Hu Q, Wu Q, Zhang W, Fang Z, Xie Q. 2009. Aligned ZnO nanorods: a useful film to fabricate amperometric glucose biosensor. Colloids Surf B Biointerfaces.74:154–158.
- Luo L, Li Q, Xu Y, Ding Y, Wang X, Deng D, Xu Y. 2010. Amperometric glucose biosensor based on NiFe2O4 nanoparticles and chitosan. Sensor Actuator B-Chem.145:293–298.
- Luong JHT, Hrapovic S, Wang D, Bensebaa F, Simard B. 2004. Solubilization of multiwall carbon nanotubes by 3-aminopropyltriethoxysilane towards the fabrication of electrochemical biosensors with promoted electron transfer. Electroanalysis.16:132–139.
- Ma W, Tian D. 2010. Direct electron transfer and electrocatalysis of hemoglobin in ZnO coated multiwalled carbon nanotubes and nafion composite matrix. Bioelectrochemistry.78:106–112.
- Manesh KM, Kim HT, Santhosh P, Gopalan AI, Lee K-P. 2008. A novel glucose biosensor based on immobilization of glucose oxidase into multiwall carbon nanotubes–polyelectrolyte-loaded electrospun nanofibrous membrane. Biosens Bioelectron.23:771–779.
- Meng F, Yin J, Duan Y-Q, Yuan Z-H, Bie L-J. 2011. Co-precipitation synthesis and gas-sensing properties of ZnO hollow sphere with porous shell. Sensor Actuator B-Chem.156:703–708.
- Miscoria SA, Barrera GD, Rivas GA. 2005. Enzymatic biosensor based on carbon paste electrodes modified with gold nanoparticles and polyphenol oxidase. Electroanalysis.17:1578–1582.
- Mizutani, F, Yabuki S. 1997. Rapid determination of glucose and sucrose by an amperometric glucose-sensing electrode combined with an invertase/mutarotase-attached measuring cell. Biosens Bioelectron.12:1013–1020.
- Moreno-Bondi MC, Wolfbeis OS, Leiner MJP, Schaffar BPH. 1990. Oxygen optrode for use in a fiber-optic glucose biosensor. Anal Chem.62:2377–2380.
- Pumera M, Sánchez S, Ichinose I, Tang J. 2007. Electrochemical nanobiosensors. Sensor Actuator B-Chem.123:1195–1205.
- Rakhi RB, Sethupathi K, Ramaprabhu S. 2009. A glucose biosensor based on deposition of glucose oxidase onto crystalline gold nanoparticle modified carbon nanotube electrode. J Phys Chemi B.113:3190–3194.
- Ramachandran A, Mary S, Yamuna A, Murugesan N, Snehalatha C. 2008. High prevalence of diabetes and cardiovascular risk factors associated with urbanization in India. Diabetes Care.31:893–898.
- Ren X, Chen D, Meng X, Tang F, Hou X, Han D, Zhang L. 2009. Zinc oxide nanoparticles/glucose oxidase photoelectrochemical system for the fabrication of biosensor. J Colloid Interface Sci.334: 183–187.
- Sadik OA, Aluoch AO, Zhou A. 2009. Status of biomolecular recognition using electrochemical techniques. Biosens Bioelectron.24:2749–2765.
- Saha S, Arya SK, Singh SP, Sreenivas K, Malhotra BD, Gupta V. 2009. Zinc oxide–potassium ferricyanide composite thin film matrix for biosensing applications. Anal Chim Acta.653:212–216.
- Salimi A, Roushani M, Soltanian S, Hallaj R. 2007. Picomolar detection of insulin at renewable nickel powder-doped carbon composite electrode. Anal Chem.79:7431–7438.
- Shafer-Peltier KE, Haynes CL, Glucksberg MR, Van Duyne RP. 2002. Toward a glucose biosensor based on surface-enhanced raman scattering. J Am Chem Soc.125:588–593.
- Sirkar K, Revzin A, Pishko MV. 2000. Glucose and lactate biosensors based on redox polymer/oxidoreductase nanocomposite thin films. Anal Chem.72:2930–2936.
- Sun XW, Wang JX, Wei A. 2008. Zinc oxide nanostructured biosensor for glucose detection. J Mat Sci Technol.24:649–656.
- Švancara I, Vytřas K, Kalcher K, Walcarius A, Wang J. 2009. Carbon paste electrodes in facts, numbers, and notes: a review on the occasion of the 50-years jubilee of carbon paste in electrochemistry and electroanalysis. Electroanalysis.21:7–28.
- Tian ZR, Voigt JA, Liu J, Mckenzie B, Mcdermott MJ. 2002. Biomimetic arrays of oriented helical ZnO nanorods and columns. J Am Chemi Soc.124:12954–12955.
- Umar A, Rahman MM, Vaseem M, Hahn Y-B. 2009. Ultra-sensitive cholesterol biosensor based on low-temperature grown ZnO nanoparticles. Electrochem Commun.11:118–121.
- Usman Ali SM, Nur O, Willander M, Danielsson B. 2010. A fast and sensitive potentiometric glucose microsensor based on glucose oxidase coated ZnO nanowires grown on a thin silver wire. Sensor Actuator B-Chem.145;869–874.
- Vaddiraju S, Legassey A, Wang Y, Qiang L, Burgess DJ, Jain F, Papadimitrakopoulos F. 2011. Design and fabrication of a high-performance electrochemical glucose sensor. J Diabetes Sci Technol.5:1044–51.
- Wang J. 1999. Amperometric biosensors for clinical and therapeutic drug monitoring: a review. J Pharm Biomed Anal.19:47–53.
- Wang J. 2005. Nanomaterial-based electrochemical biosensors. Analyst.130:421–426.
- Wang J. 2007. Electrochemical glucose biosensors. Chem Rev.108: 814–825.
- Wang X-D, Chen H-X, Zhou T-Y, Lin Z-J, Zeng J-B, Xie Z-X, et al. 2009a. Optical colorimetric sensor strip for direct readout glucose measurement. Biosens Bioelectron.24:3702–3705.
- Wang X, Hagiwara T, Uchiyama S. 2007. Immobilization of uricase within polystyrene using polymaleimidostyrene as a stabilizer and its application to uric acid sensor. Anal Chim Acta.587:41–46.
- Wang Y-T, Yu L, Zhu Z-Q, Zhang J, Zhu J-Z, Fan C-H. 2009b. Improved enzyme immobilization for enhanced bioelectrocatalytic activity of glucose sensor. Sensor Actuator B-Chemical.136:332–337.
- Wei Y, Li Y, Liu X, Xian Y, Shi G, Jin L. 2010. ZnO nanorods/Au hybrid nanocomposites for glucose biosensor. Biosens Bioelectron.26: 275–278.
- Xiao F, Zhao F, Mei D, Mo Z, Zeng B. 2009. Nonenzymatic glucose sensor based on ultrasonic-electrodeposition of bimetallic PtM (M = Ru, Pd and Au) nanoparticles on carbon nanotubes–ionic liquid composite film. Biosens Bioelectron, 24:3481–3486.
- Xiao L, Chen J, Cha C-S. 2000. Elimination of the interference of ascorbic acid in the amperometric detection of biomolecules in body fluid samples and the simple detection of uric acid in human serum and urine by using the powder microelectrode technique. J Electroanalytical Chem.495:27–35.
- Yang Z, Ye Z, Zhao B, Zong X, Wang P. 2010. A rapid response time and highly sensitive amperometric glucose biosensor based on ZnO nanorod via citric acid-assisted annealing route. Physica E: Low Dimens Syst Nanostruct.42:1830–1833.
- Ye J-S, Wen Y, De Zhang W, Ming Gan L, Xu GQ, Sheu F-S. 2004. Nonenzymatic glucose detection using multi-walled carbon nanotube electrodes. Electrochem Commun.6:66–70.
- Yoshimura K, Hozumi K. 1996. Response characteristics of a glucose electrode with a sensing membrane prepared by plasma polymerization. Microchem J.53:404–412.
- Zang J, Li CM, Cui X, Wang J, Sun X, Dong H, Sun CQ. 2007. Tailoring zinc oxide nanowires for high performance amperometric glucose sensor. Electroanaly.19:1008–1014.
- Zhang S, Yang W, Niu Y, Sun C. 2004. Multilayered construction of glucose oxidase on gold electrodes based on layer-by-layer covalent attachment. Anal Chim Acta.523:209–217.
- Zhao G, Feng J-J, Xu J-J, Chen H-Y. 2005. Direct electrochemistry and electrocatalysis of heme proteins immobilized on self-assembled ZrO2 film. Electrochem Commun.7:724–729.
- Zhao J, Wang F, Yu J, Hu S. 2006. Electro-oxidation of glucose at self-assembled monolayers incorporated by copper particles. Talanta.70:449–454.
- Zhao J, Wu D, Zhi J. 2009. A novel tyrosinase biosensor based on biofunctional ZnO nanorod microarrays on the nanocrystalline diamond electrode for detection of phenolic compounds. Bioelectrochem.75:44–49.
- Zhao ZW, Chen XJ, Tay BK, Chen JS, Han ZJ, Khor KA. 2007. A novel amperometric biosensor based on ZnO:Co nanoclusters for biosensing glucose. Biosens Bioelectron.23:135–139.
- Zhou H, Chen H, Luo S, Chen J, Wei W, Kuang Y. 2005. Glucose biosensor based on platinum microparticles dispersed in nano-fibrous polyaniline. Biosens Bioelectron.20:1305–1311.
- Zhu L, Li Y, Tian F, Xu B, Zhu G. 2002. Electrochemiluminescent determination of glucose with a sol–gel derived ceramic–carbon composite electrode as a renewable optical fiber biosensor. Sensor Actuator B-Chem.84:265–270.