Abstract
The pure motor peripheral nerves animal model, obtained by ablating the dorsal root ganglions (DRGs), was used to investigate the accuracy of peripheral nerve selective regeneration using small gap sleeve bridging compared to epineurium neurorrhaphy. The results showed that the number of improperly regenerated motor axons in the distal stump and the misrouting ratio were significantly lower when the nerve transection was treated by small gap sleeve bridging. This suggests that more accurate reinnervation may be achieved by small gap sleeve bridging than by traditional epineurium neurorrhaphy.
Introduction
Peripheral nerve injury is a serious health problem in today's society that can lead to lifelong disability and permanent disfigurement (Ichihara et al. Citation2008, Biazar et al. Citation2010). Peripheral nerves generally consist of both motor and sensory fibers. One important factor in postoperative functional recovery is the correct routing of the proximal sensory and motor tracts to their corresponding tracts in the distal stumps during regeneration. Unfortunately, nerve injury typically results in the mistargeting of regenerating axons from the proximal to distal nerve stumps. Functional recovery greatly depends on the correct projection and appropriate reestablishment of nerve–target connections and many studies focus on improving the accuracy of peripheral nerve regeneration. To identify sensory and motor fascicles, various types of techniques have been developed, including intraoperative electro-stimulation (Jiang et al. Citation2006, Hakstian Citation1968), single fascicular recordings (Williams and Terzis Citation1976), and various types of staining techniques (Gruber and Zenker Citation1973). These intraoperative diagnostic tools have been introduced for the management of peripheral nerve lesions but have limited practical use for most reconstructive nerve surgeons. Biological and molecular mechanisms regulate individual regeneration of axons in fascicles and further study of these mechanisms will lead to improved treatment of peripheral nerve injury (Lundborg Citation2000).
Many studies have investigated the nerve selective regeneration theory (Yu et al. Citation2009, Al-Majed et al. Citation2000). For over 20 years, our lab has investigated the application of small gap sleeve bridging fields. Tubulation in the peripheral nerve lesion site was initially used as an alternative to nerve grafts to avoid downsides including donor site morbidity induced by the graft harvest and the limited length of the available graft material. Although the gap is longer than 5 mm, the gold standard for bridging the proximal and distal stumps is still the nerve autograft (Brooks et al. Citation2012). We have attempted to improve the nerve regenerative effect by determining the optimal conditions for axonal regeneration over a surgically induced short, empty space between the proximal and distal nerve ends. Previous experiments confirm the benefit of using a conduit small gap sleeve bridging to substitute traditional epineurium neurorrhaphy. This study was designed to determine if small gap sleeve bridging improves the accuracy of peripheral nerve selective regeneration.
Material and methods
Experimental design
The experiments were performed with the adult rat femoral nerve that consists of intermingled sensory and motor fibers. A total of 12 male Sprague-Dawley rats (200–250 g) were randomly divided into Group I and Group II, and both underwent surgically induced peripheral nerve injury. The rats in Group I were treated with epineurium neurorrhaphy and the rats in Group II were treated with small gap sleeve bridging. After two months, pure motor peripheral nerves were collected from the two groups by ablating the dorsal root ganglions (DRGs). These rats were sacrificed one month later, and the nerve tissues were collected for morphological evaluation.
Animal preparation
The animals were housed under specific pathogen-free laboratory conditions with controlled lighting and were given a rodent diet and water ad libitum. Every effort was made to minimize animal suffering and to reduce the number of animals used. All of the surgical procedures, experimental manipulations and peri-operative care measures were performed in strict accordance with the Chinese Guidelines for the Care and Use of Laboratory Animals and were approved by the People's Hospital of Beijing University Medical Ethics Committee. The animals were returned to the animal facility following the surgical procedures and were monitored for infection and other disabilities.
Surgical techniques
The first surgery (femoral nerve injury and repair animal model)
General anesthesia (sodium pentobarbital, 30 mg/kg) was administered by intraperitoneal injection. After the rats had been anesthetized, their right inguinal regions were shaved, and the surgeries were performed under aseptic conditions. The surgical procedures were performed under a binocular surgical microscope using standard microsurgical techniques. The femoral nerve was exposed using an inguinal approach and was separated from its ensheathing fascial layer and surrounding vasculature by gentle dissection to minimize tension on the subsequent repair site. The parent nerve was transected with microscissors about 7–8 mm proximal to the bifurcation point, a region where axons to the two terminal nerve branches are randomly distributed (Brushart Citation1988). For the rats in Group I, the transected femoral nerve was repaired by an epineurium neurorrhaphy with 10–0 nylon suture (see ). For the rats in Group II, 6 mm biodegradable chitin conduits were placed at the repair site, a 2 mm gap was maintained between the proximal and distal nerve segments (see ) and the nerve was anchored with a 10–0 nylon suture to the channel for stability (details regarding this surgical procedure have been presented by Jiang Baoguo, 2006). Biodegradable chitin conduits (which have been patented by our laboratory and authorized by the State Intellectual Property Office of the People's Republic of China No. ZL01 136314.2) are artificial nerve grafts consisting of a polysaccharide shell that demonstrates satisfactory biocompatibility and degradation characteristics. They are now being used in a preclinical study. The biodegradable chitin conduits used were 0.1 mm thick and had a 1.5 mm inner diameter. Finally, the surgical site was closed in layers with 4–0 nylon sutures and the rats were allowed to recover.
Figure 1. Operating microscope images (A, C, E, 5.5×) displaying the gross anatomy of the surgical procedures and the corresponding schematic drawings (B, D, F). A and B demonstrate the surgical procedures of repairing the femoral nerve by epineurium neurorrhaphy. C and D demonstrate the surgical procedures for repairing the femoral nerve by small gap sleeve bridging with a biodegradable chitin conduit. The 6 mm biodegradable chitin conduits were placed at the repair site and a 2 mm gap was maintained between the proximal and distal nerve segments. E and F demonstrate the removal of the sensory axons by ablating the right L2–4 DRGs and the arrows in photo E indicate the right L2–4 DRGs.
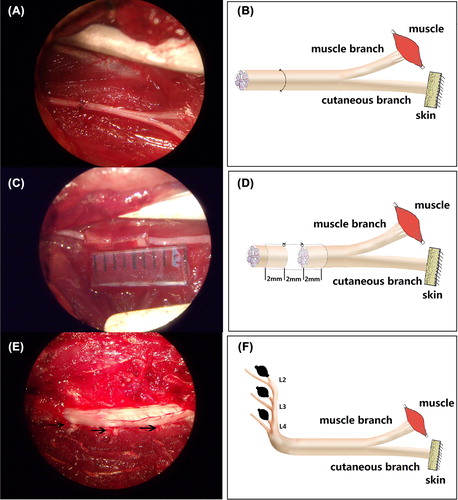
The second surgery (pure motor peripheral nerve)
Two months after the first surgical operation, general anesthesia was administered as previously described. The anesthetized animals were shaved and prepared, and the surgeries were performed under aseptic conditions. A posterior midline incision in the pars lumbalis was made to strip the erector spinae, and the lumbar spinous processes and laminae were exposed. The dural sac was carefully opened with a pair of microsurgical scissors. The right L2–4 DRGs were selectively ablated in the two groups (see ). In addition, the muscles, subcutaneous tissues, and skin were closed and the animals were allowed to survive for one month to provide them with sufficient time for Wallerian degeneration of the sensory axons in the femoral nerve to occur.
Histological study
One month after the second surgery, the animals were sacrificed with an overdose of sodium pentobarbital and perfused via the aorta with 100 mL of saline followed by 500 mL of ice-cold 4% paraformaldehyde (pH 7.4). After perfusion, the right femoral nerve and the saphenous nerve, including the proximal and distal segments, were removed from each rat. The tissues were fixed in 4% paraformaldehyde in 0.1 M phosphate buffer for 12 h at 4°C. After this, the nerves were rinsed twice in phosphate buffer, and three tissue blocks (approx. 5–6 mm long) were cut; one proximal and one distal to the suture site, along with the saphenous nerve. Then each sample was fixed in 1% osmium tetroxide for 12 h, dehydrated through a graded series of ethanol and embedded in paraffin. Specimen transections (5 μm) were then taken for osmium acid staining and the images were collected using an Olympus DP50 camera.
Statistical analysis
The number of parent myelinated axons in the proximal stump and regenerating myelinated axons in the distal stump and the saphenous nerve stump were counted using the counting tool in the Photoshop CS4 software. The misrouting ratio was calculated by dividing the number of axons in the saphenous nerve stump by the number of regenerating myelinated axons in the distal stump. The mean number of myelinated axons, the mean misrouting ratio and the standard deviation were then calculated using SPSS 15.0 software for each group. The results are presented as the mean ± deviation. The differences in the number of parent myelinated axons, regenerating myelinated axons and misrouting ratio between the two groups were analyzed using a t-test. A P-value of less than 0.05 was considered statistically significant.
Results
General observation
All of the animals used in this study survived the operations and the desired duration of the experiments. They showed normal eating and drinking behavior, and there were no signs of infection. The right hind limbs and feet of the animals in Group II showed mild swelling. Neither autophagy nor significant denervated muscle atrophy was observed in any animals.
Histomorphometry
Morphological analysis of the mean number of the parent motor axons in the proximal stump revealed no significant difference between the groups, with 425.67 ± 15.07 axons in Group I and 427.33 ± 19.39 axons in Group II. In the proximal stumps, the motor axons were evenly distributed with similar fiber diameter (see ). Although the number of regenerating motor axons in Group I (1249.67 ± 66.32) were slightly higher than in Group II (1186.50 ± 54.62), this difference was not statistically significant (P = 0.05).
Figure 2. Light microscopy images (10 × 100) of the parent motor axons in the proximal stumps (A: Group I; B: Group II), the regenerating axons in the distal stumps (C: Group I; D: Group II), and the misrouted motor axons in the cutaneous stumps (E: Group I; F: Group II). The scale bar is 20 μm.
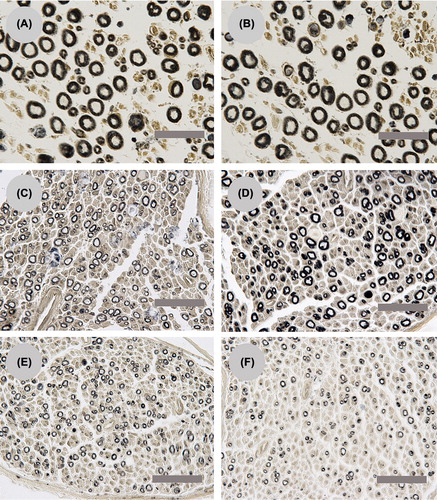
The misrouted axons were found in the regenerating motor axons in the saphenous nerve stump, and the misrouted axons in Group I (568.67 ± 21.36) were significantly more than Group II (378.17 ± 29.86) (P < 0.05). The number of parent motor axons in the proximal stump and regenerating motor axons in the distal and saphenous nerve stump is presented in . The misrouting ratio in Group I (45.56 ± 1.80%) was significantly higher than in Group II (31.93 ± 3.05%) (P < 0.05). For both groups, the regenerating motor axons were evenly distributed and the diameter of the fibers was similar (see ). The misrouted motor axons in the saphenous nerve of Group I were more mature than in Group II determined by the larger diameter of the nerve fiber and increased thickness of the myelin sheath in Group I (see ).
Figure 3. A comparison of the different numbers of parent motor axons in the proximal stump and regenerating motor axons in the distal stump and saphenous nerve stump for Group I and Group II. NPMA: the number of parent motor axons; NRMA: the number of regenerating motor axons; NMMA: the number of misrouting motor axons. Neither the number of parent motor axons nor the regenerating motor axons exhibited a significant difference between Group I and Group II. The number of misrouting motor axons in the saphenous nerve for Group I is significantly more than Group II.
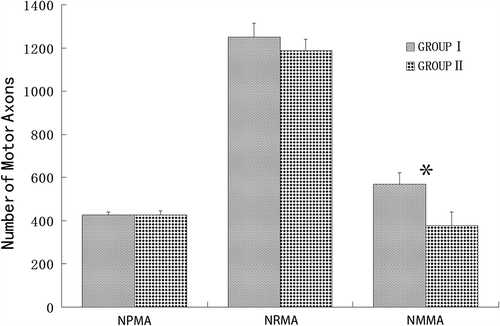
Discussion
The femoral nerve divides distally to the inguinal ligament into one pure cutaneous branch (the saphenous nerve) and a muscle branch to the quadriceps. Schwann cell tubes leading to the terminal cutaneous and quadriceps muscle branches may be treated as relatively equal “targets” (Brushart Citation1993). The rat femoral nerve was introduced as a model system to study the fate of regenerating axons when they are given equal access to the terminal cutaneous and muscle branches (Weiss and Edds Citation1945). In an uninjured femoral nerve, no motor axons project into the cutaneous branch. Thus, reinnervation of the distal cutaneous branch by regenerating motor axons represents a failure of specificity. Previous studies have demonstrated specific regeneration quantified by simple retrograde tracing, but this method is neither direct nor accurate. In this study, pure regenerating motor axons were attained by ablating the L2-L4 DRGs, which send sensory axons to the femoral nerve. Next, the misrouting ratio was calculated by dividing the number of motor axons that mistarget to the cutaneous branch by the total number of regenerating motor axons. These results suggested that, for two different suture methods, the regenerating motor neurons preferentially reinnervated a terminal nerve branch to the muscle as opposed to the skin, which are consistent with previous studies. This process is also known as preferential motor reinnervation (PMR) (Madison et al. Citation2007).
Regenerating motor axons in the muscle branch cannot represent proper reinnervation because 50%–60% of the myelinated axons in the muscle branching to the quadriceps are afferent fibers that innervate proprioceptors in the muscle spindle (Midha et al. Citation2001). The discussion in this study will therefore be limited to the comparison of the misrouting ratio of motor reinnervation for the two different suture methods. In this study, the misrouting ratio of Group I is significantly higher than Group II, which indicated that small gap sleeve bridging may improve the accuracy of peripheral nerve selective regeneration. We have demonstrated that using a conduit small gap sleeve bridging to substitute for the traditional epineurium neurorrhaphy can achieve enhanced recovery of morphology and electrophysiology in rats, rabbits, and rhesus monkeys (Zhang et al. Citation2008, Zhang et al. Citation2009). The lower misrouting ratio that was obtained using small gap sleeve bridging is an important discovery possibly leading to a more functional recovery over epineurium neurorrhaphy, although the regenerating axons for the two suture methods were not significantly different.
The peripheral nervous system is capable of robust regeneration after a nerve lesion. Many studies have demonstrated that one parent axon can regenerate and maintain several collaterals in a regenerative distal stump. This process is often referred to as “multiple regeneration.” In this study, the number of regenerating motor axons is about three times more than the parent motor axons in the proximal stump for both groups. However, the capacity for robust regeneration after peripheral nerve lesions does not provide sufficient functional recovery, which requires that the regenerating axons accurately reinnervate to their original target organs. Misdirected regeneration is a major barrier to functional recovery. During the early stages of regeneration the motor axons produce multiple collateral sprouts that randomly reinnervate a previous sensory or motor Schwann cell tube. Specificity of peripheral nerve regeneration may be generated by the pruning collaterals in foreign pathways and maintaining these collaterals within the appropriate basement membrane tube (Kawamura et al. Citation2010). This mechanism of specificity is commonly referred to as the “pruning hypothesis” but this was not the focus of our experiments so we were unable to determine this.
While the axon segment distal to the injury site degenerates, the Schwann cells that were originally associated with the myelinated axons typically proliferate within the basal lamina and form the “Bands of Bungner” (Chew et al. Citation2008). The basal lamina scaffolds of Schwann cells in the distal stump serve as effective pathways for the elongation, maintenance, and maturation of regenerating axons (Chew et al. Citation2008). The initial misrouting axons not only fail to establish functional contacts, but also exclude appropriate axons from the pathways that they occupy. Thus, an initial correct projection is important for functionary recovery. Robinson and Madison demonstrated that the cutaneous pathway is capable of supporting robust motor axon regeneration (Robinson and Madison Citation2004). The regenerating nerve fibers distal to the nerve repair return to normal levels within 2 years (Mackinnon et al. Citation1991). In this study, the misrouting ratio is 45.56 ± 1.80% for Group I and 31.93 ± 3.05% for Group II providing evidence that initial reinnervation is not random and only a partial regeneration of the axons was sent to the foreign location. Thus the pruning mechanism is responsible for ameliorating the regenerative accuracy.
The mechanism of lowering the misrouting ratio by using small gap sleeve bridging is not completely understood from the data presented here. The chitin conduits provide an effective nerve regenerating chamber, which can form a microenvironment that is suitable for the diffusion of target-derived neurotrophic factors. The activity of the fluid collected from the regeneration chambers was tested in previous studies, neurotrophic factors such as nerve growth factor (NGF), brain-derived nerve growth factor (BDNF), glial-cell-line-derived neurotrophic factor (GDNF), and insulin-like growth factors (IGFs) were detected (Fox et al. Citation2007). Lundborg et al. and Mackinnon et al. have demonstrated that target-derived neurotrophic factors contribute to tissue specificity of regenerating axons and they will grow toward the distal nerve stump rather than the tendon, muscle, or granulation tissue. This phenomenon is referred to as neurotropism (chemotropism) (Lundborg et al. Citation1986). However, it is not clear if target- derived neurotrophic factors generate the initial motor–sensory specificity. Several previous assessments of reinnervation accuracy in the adult femoral nerve model have demonstrated the effects of denervated end-organs on the guidance choices of motor neurons at the nerve repair site (Robinson and Madison Citation2006). These findings suggested that the relative trophic support provided to the neurons and/or proximal stumps by the denervated end-organs are an important determinant of regeneration accuracy (Uschold et al. Citation2007). Another probable explanation for motor–sensory pathway recognition is the presence of recognition molecules present in the motor Schwann cell tubes as opposed to the sensory Schwann cell tubes. Brushart (Citation1993) has demonstrated that PMR would function regardless of whether the axons had eventually contacted the muscle or skin, suggesting that the nerve pathway itself is sufficiently competent to generate the PMR. The L2 carbohydrate is rarely found on sensory axons, but it is selectively expressed on the Schwann cells and the Schwann cell basement membranes of motor axons (Hall et al. Citation1993). Specific recognition contributes to pathway selection, (du Lac et al. Citation1986) thus epineurium neurorrhaphy is limited because it does not provide an appropriate cavity for the regenerating axons to find their counterpart in the distal nerve stumps. For an enhanced functional recovery, a specific distance is needed for the nerve to complete its appropriate selection.
Conclusion
Satisfactory functional recovery after nerve injury depends on regenerating axons accurately reaching and reinnervating the appropriate target end-organs. Small gap sleeve bridging with biodegradable chitin conduits can create optimal conditions for axonal regeneration and improve regeneration accuracy. The emphasis of the entubulation has been placed on the intrinsic healing capacities of the nerve rather than on the surgeon's technical skill. In future studies, because of the enhanced initial specificity, a peripheral nerve injury may be repaired by small gap sleeve bridging as an alternative to conventional repair techniques.
Acknowledgments
We sincerely thank the Department of Orthopedics, Peking Union Medical College Hospital, Beijing, P. R. China, and the Department of Trauma Orthopedics, Peking University People’s Hospital, Beijing, P. R. China for their help with animal feeding and data collection.
Declaration of interest
The authors report no declarations of interest. The authors alone are responsible for the content and writing of the paper.
This research project was funded by the Chinese National Natural Science Fund for Outstanding Youth (30625036); Chinese 973 Project Planning (2005CB522604); Chinese National Natural Science Youth Fund (30801169); Beijing City Science & Technology New Star Classification A-2008-10; Chinese National Natural Science Fund (31171150, 81171146, 30971526, and 31040043); and Chinese Educational Ministry New Century Excellent Talent Support Project (2011).
References
- Al-Majed AA, Neumann CM, Brushart TM, Gordon T. 2000. Brief electrical stimulation promotes the speed and accuracy of motor axonal regeneration. J Neurosci. 20:2602–2608.
- Biazar E, Khorasani MT, Montazeri N, Pourshamsian K, Daliri M, Rezaei M, et al. 2010. Types of neural guides and using nanotechnology for peripheral nerve reconstruction. Int J Nanomedicine. 5:839–852.
- Brooks DN, Weber RV, Chao JD, Rinker BD, Zoldos J, Robichaux MR, et al. 2012. Processed nerve allografts for peripheral nerve reconstruction: a multicenter study of utilization and outcomes in sensory, mixed, and motor nerve reconstructions. Microsurgery. 32:1–14.
- Brushart TM. 1988. Preferential reinnervation of motor nerves by regenerating motor axons. J Neurosci. 8:1026–1031.
- Brushart TM. 1993. Motor axons preferentially reinnervate motor pathways. J Neurosci. 13:2730–2738.
- Chew SY, Mi R, Hoke A, Leong KW. 2008. The effect of the alignment of electrospun fibrous scaffolds on Schwann cell maturation. Biomaterials. 29:653–661.
- du Lac S, Bastiani MJ, Goodman CS. 1986. Guidance of neuronal growth cones in the grasshopper embryo. II. Recognition of a specific axonal pathway by the aCC neuron. J Neurosci. 6:3532–3541.
- Fox MA, Sanes JR, Borza DB, Eswarakumar VP, Fassler R, Hudson BG, et al. 2007. Distinct target-derived signals organize formation, maturation, and maintenance of motor nerve terminals. Cell. 129:179–193.
- Gruber H, Zenker W. 1973. Acetylcholinesterase: histochemical differentiation between motor and sensory nerve fibres. Brain Res. 51:207–214.
- Hakstian RW. 1968. Funicular orientation by direct stimulation. An aid to peripheral nerve repair. J Bone Joint Surg Am. 50:1178–1186.
- Hall H, Liu L, Schachner M, Schmitz B. 1993. The L2/HNK-1 carbohydrate mediates adhesion of neural cells to laminin. Eur J Neurosci. 5:34–42.
- Ichihara S, Inada Y, Nakamura T. 2008. Artificial nerve tubes and their application for repair of peripheral nerve injury: an update of current concepts. Injury. 39:29–39.
- Jiang B, Zhang P, Zhang D, Fu Z, Yin X, Zhang H. 2006. Study on small gap sleeve bridging peripheral nerve injury. Artif Cells Blood Substit Immobil Biotechnol. 34:55–74.
- Kawamura DH, Johnson PJ, Moore AM, Magill CK, Hunter DA, Ray WZ, et al. 2010. Matching of motor-sensory modality in the rodent femoral nerve model shows no enhanced effect on peripheral nerve regeneration. Exp Neurol. 223:496–504.
- Lundborg G, Dahlin LB, Danielsen N, Nachemson AK. 1986. Tissue specificity in nerve regeneration. Scand J Plast Reconstr Surg. 20:279–283.
- Lundborg G. 2000. A 25-year perspective of peripheral nerve surgery: evolving neuroscientific concepts and clinical significance. J Hand Surg Am. 25:391–414.
- Mackinnon SE, Dellon AL, O’Brien JP. 1991. Changes in nerve fiber numbers distal to a nerve repair in the rat sciatic nerve model. Muscle Nerve. 14:1116–1122.
- Madison RD, Robinson GA, Chadaram SR. 2007. The specificity of motor neurone regeneration (preferential reinnervation). Acta Physiol (Oxf). 189:201–206.
- Midha R, Nag S, Munro CA, Ang LC. 2001. Differential response of sensory and motor axons in nerve allografts after withdrawal of immunosuppressive therapy. J Neurosurg. 94:102–110.
- Robinson GA, Madison RD. 2004. Motor neurons can preferentially reinnervate cutaneous pathways. Exp Neurol. 190:407–413.
- Robinson GA, Madison RD. 2006. Developmentally regulated changes in femoral nerve regeneration in the mouse and rat. Exp Neurol. 197:341–346.
- Uschold T, Robinson GA, Madison RD. 2007. Motor neuron regeneration accuracy: balancing trophic influences between pathways and end-organs. Exp Neurol. 205:250–256.
- Weiss P, Edds MV. 1945. Sensory-motor nerve crosses in the rat. J Neurophysiol. 30:173–193.
- Williams HB, Terzis JK. 1976. Single fascicular recordings: an intraoperative diagnostic tool for the management of peripheral nerve lesions. Plast Reconstr Surg. 57:562–569.
- Yu K, Zhang C, Wang Y, Zhang P, Zhang D, Zhang H, Jiang B. 2009. The protective effects of small gap sleeve in bridging peripheral nerve mutilation. Artif Cells Blood Substit Immobil Biotechnol. 37:257–264.
- Zhang P, Yin X, Kou Y, Wang Y, Zhang H, Jiang B. 2008. The electrophysiology analysis of biological conduit sleeve bridging rhesus monkey median nerve injury with small gap. Artif Cells Blood Substit Immobil Biotechnol. 36:457–463.
- Zhang P, Zhang C, Kou Y, Yin X, Zhang H, Jiang B. 2009. The histological analysis of biological conduit sleeve bridging rhesus monkey median nerve injury with small gap. Artif Cells Blood Substit Immobil Biotechnol. 37:101–104.