Abstract
In this work, doxorubicin (DOX)-loaded magnetic albumin nanospheres were prepared using desolvation method in order to develop magnetically responsive nanocarrier system. Nanoparticles were characterized with zetasizer, SEM, AFM, XRD and magnetometer. In vitro DOX release was also investigated. It was found that nanoparticles had spherical shape with narrow size distributions and had magnetic responsiveness. In addition, slower drug release was observed with nanoparticulate system compared with free DOX. Moreover, the release rate should accelerate at target sites (cancer cells or tissue) where proteolytic enzymes are more abundant as known; therefore, magnetic albumin nanospheres can be effective potential DOX carrier with targeting ability.
Introduction
Albumin is the most abundant plasma protein and is synthesized in the liver where it is produced at a rate of approximately 0.7 mg/h for every gram of liver (Kratz 2008). Over the past decades, albumin has emerged as a versatile carrier for therapeutic and diagnostic agents, rheumatoid arthritis and infectious diseases (Elsadek and Kratz 2012). Nanoparticles made of albumin offer several specific advantages: they are non-toxic, non-antigenic, biodegradable, easy to prepare and reproducible. Also, they are expected to be well tolerated, which is supported by clinical studies with registered HSA-based particle formulations such as AlbunexTM and AbraxaneTM (Elzoghby et al. 2012).
Cancer is on the increase in the developing world as well as in the industrial world. The latest world cancer statistics report that the number of new cancer cases will increase to more than 15 million by 2020 (Kairemo et al. 2008). According to the Cancer Statistics 2012, it is estimated that more than 1500 people per day will die by this year due to cancer in the United States (Siegel et al. 2012). Chemotherapy is a major therapeutic approach for the treatment of localized and metastasized cancers. Most of the conventional chemotherapeutics agents have poor pharmacokinetics profiles and are distributed non- specifically in the body leading to systemic toxicity (Danhier et al. 2010). Doxorubicin (DOX, anthracyclinic antibiotic) is one of the most potent and widely used anticancer drugs. Its mode of action is complex and not completely understood, but it is believed to interact with cell DNA by intercalation and subsequent inhibition of biosynthesis. DOX has a number of undesirable side effects such as nausea, vomiting, hair loss, cardiotoxicity and myelosupression which lead to a very narrow therapeutic index (Brannon-Peppas and Blanchette 2004, Shen et al. 2011a). Novel delivery systems have permitted cancer treatments that have significantly reduced side effects, and have enabled new and better chemotherapeutic regimens using existing pharmaceuticals (Moses et al. 2003). There are FDA-approved liposomal DOX encapsulations (Doxil®/Caelyx® and MyocetTM) (Wagner et al. 2010), and researchers have studied various DOX carrier systems for targeted delivery and improved therapeutic index up to now (Reddy et al. 2004, Qu et al. 2010, Jeong et al. 2010, Min et al. 2011).
The specific tumour targeting of nanocarriers leads to better profiles of pharmacokinetics and pharmacodynamics, controlled and sustained release of drugs, an improved specificity, an increased internalization and intracellular delivery and, more importantly, a lower systemic toxicity. The tumour targeting consists in passive and active targeting (Danhier et al. 2010). Active targeting is based on the over- or exclusive expressionof different epitopes or receptors in tumoral cells and on specific physical characteristics. Thus, vectors that are sensitive to physical stimuli (e.g. temperature, pH, electric charge, light, sound and magnetism) have been developed (Arruebo et al. 2007). Magnetic nanoparticles are being of great interest due to their unique purposes and have been actively investigated as the next generation of targeted drug delivery for more than thirty years (Chomoucka et al. 2010). In summary, for magnetic targeting, magnetic nanoparticle introduced first in the body and then under the influence of external magnets, it is possible to guide nanoparticles to a particular targeted site (Arruebo et al. 2007, Danhier et al. 2010).
The aim of this work is to synthesize DOX-carrying nanoparticulate formulation that can be controlled by the external magnetic field in order to address tumour region and can release the drug especially at this region resulting in improved therapeutic index. Widder et al. have evaluated biologically active magnetic albumin microspheres containing DOX (1980), but DOX-incorporated magnetic albumin nanospheres have not been reported yet. In this study, we prepared nanoparticles with a simple and cheap method using bovine serum albumin (BSA) as a biopolymer, and in vitro drug release was investigated.
Materials and methods
Materials
BSA was purchased from Sigma, glutaraldehyde (GA) was obtained from Merck, and DOX was supplied commercially. Fe3O4 nanoparticles (magnetite) were synthesized as previously reported (Dung et al. 2009). All the other reagents were of analytical grade. The water used in experiments was purified using Milli-Q Advantage A10.
Synthesis of albumin nanoparticles by desolvation method
BSA nanoparticles (BSA-NPs) were synthesized with the minor modification of the method described by Langer et al. (2003). Briefly, 25–100 mg of BSA was dissolved in 1 ml of water and 4 ml of ethanol (the desolvation agent) was added dropwise by a tubing pump under shaking (480 rpm) at room temperature. Then, 0.588 μl GA (8%)/mg BSA was added and left to shake for 24 h. At the end of the time period, nanoparticles were purified and washed with water by centrifugation to remove non-desolvated protein and excess of the cross-linking agent. Non-desolvated protein in supernatants was defined via Bradford protein measurement assay (Bradford 1976), and desolvation efficiency was calculated using the following formulae.
All these and the following analysis in that report were repeated three times, and standard deviation values were calculated.
DOX loading into albumin nanoparticles
Incorporation of DOX into BSA-NPs was achieved according to Dreis et al. with a minor modification (2007). Twenty-five milligrams of albumin and 0.5–5 mg of DOX were dissolved in 1 ml of water (pH 6.5), and then the method for nanoparticle synthesis was applied as mentioned earlier. The amount of DOX in the collected supernatants was measured using UV–vis spectrophotometer (Perkin Elmer Lambda 35) at 480 nm using calibration curve (y = 18.613x) constructed with DOX solutions (10–50 μg/ml) in water to determine DOX content in DOX-incorporated BSA nanoparticles (DOX-BSA-NPs). Drug incorporating content (DIC) and drug incorporating efficiency (DIE) were evaluated using the following equations:
Preparation of Fe3O4 and DOX-loaded BSA nanoparticles
To DOX–albumin solution, 1.5–7.5 mg magnetite was added, and then, standard procedure was carried out. After 24 h and purification studies, DOX-loaded magnetic BSA nanoparticles (M-DOX-BSA-NPs) were formed. DOX incorporating content and DOX incorporating efficiency were also calculated, and all the calculations are shown in .
Table I. DIC and DIE calculations, mean particle size and PDI of nanoparticles.
Characterization of nanoparticles with zetasizer, SEM and AFM
Size analysis of nanoparticles diluted with water was carried out at room temperature using zeta sizer (NanoSZ, Malvern). Mean particle diameter and polydispersity index (PDI) of nanoparticles are demonstrated in . Scanning electron microscope (SEM, Philips XL-30S FEG and FEI Quanta250 FEG) imaging analysis of freeze-dried nanoparticles was performed in Izmir Institute of Technology, and atomic force microscopy (AFM, NT-MDT Ntegra Solaris, non-contact mode) was used for imaging of M-DOX-BSA-NPs in Selçuk University. Nanoparticles were observed using mica surface.
Identification of phase composition and magnetization
BSA nanoparticles and magnetic BSA nanoparticles were analysed for phase composition using X-ray powder diffractometer (XRD, Philips X-Pert Pro) over the 2q range from 0 to 80° at a rate of 2.5°/min, using Cu-Kα radiation.
Magnetism of magnetite and M-DOX-BSA-NPs was determined using magnetometer (Lake Shore Vibrometric Sample Magnetometer). Five hundred Gauss (G) of magnetic field was applied to nanoparticles at room temperature, and their magnetic properties were identified. Both the analyses were performed in Izmir Institute of Technology.
In vitro drug release studies
In vitro release studies were performed in three steps. First, various GA amounts (25–100% of the calculated amount necessary for the quantitative cross-linking of the amino groups of lysine in albumin molecules, Langer et al. 2003) which were used during nanoparticle preparation were evaluated based on the release amounts of DOX to detect optimum value of GA for ideal cross-linking. The nanoparticles were dispersed in water (pH adjusted to 5) and sealed in dialysis tubing (12.000 MWCO). The dialysis tubings were placed in PBS at pH 5.0 and then set in a water bath at 37°C with constant shaking. Samples were taken at predetermined time intervals and replaced with pre-warmed fresh media. The amount of DOX released in collected sample was analysed spectrophotometrically. The cumulative fraction of released DOX versus time was expressed by the following equation as described by Gaihre et al. (2009):
Second, release studies in 10 mM phosphate-buffered saline (PBS) solution at pH 5.0, 6.0 and 7.4 were carried out using M-DOX-BSA-NPs synthesized with chosen optimum GA amount. DOX release assay from free form as control group was also evaluated at the same conditions, and the results were compared with each other. Finally, the release of DOX from M-DOX-BSA-NPs in the presence of chymotrypsin (110.9 U/per dialysis tubing) was evaluated in the same conditions. Two milligrams of the enzyme that has the specific activity of 55.45 U/mg was added to the dialysis tubing, and DOX release studies were performed.
Results and discussion
Synthesis and characterization of nanoparticles
In the present study, we synthesized BSA nanoparticles using desolvation method since this method is simpler, and resulting particles can be more easily cleaned. Moreover, obtained particles are in range of nanometer size compared to those synthesized using the emulsion techniques (Kumar 2006). BSA has been used in many studies as a drug carrier (Merodio et al. 2001, Li et al. 2008, 2009, Qu et al. 2010, Zhao et al. 2010). In case the size and size distribution of nanoparticles can alter their fate of metabolism in the body, hydrodynamic diameter and PDI of nanoparticles were measured in each step.Moreover, the size of particles that were distributed into the bloodstream must be significantly smaller than 5 μm, without forming aggregates, to ensure that the particles do not cause an embolism (Singh and Lillard 2009). Initially, BSA concentration effect on nanoparticle formation was evaluated for the preparation of empty nanoparticles. Protein content of the nanoparticles was calculated using BSA standard curve. Desolvation efficiency was above 80% for each nanoparticle, and also, they had a monomodal size distribution. There was a slight rise in desolvation efficiency and in the size of nanospheres with increased BSA concentration (seen in –A), therefore 25 mg/ml was chosen as an ideal albumin amount. Observations are in accordance with those of the earlier work, but we obtained smaller nanoparticles compared to the nanoparticles reported in the study by Langer et al. (2003). Nanoparticles synthesized with 25 mg/ml albumin concentration had 94.18 ± 8.20 nm of mean hydrodynamic diameter.
Figure 1. Particle size and (A) desolvation efficiency of BSA-NPs at different BSA concentrations; (B) DOX incorporation efficiency of DOX-BSA-NPs at different DOX concentrations; and (C) DOX incorporation efficiency of M-DOX-BSA-NPs at different magnetite concentrations.
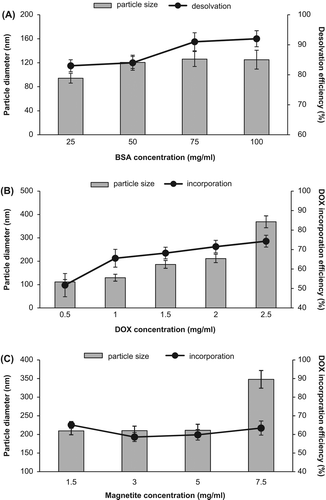
DOX can entrap in albumin nanospheres via the combination of hydrophobic and ionic interactions (between –NH3+ of DOX and –COO− of albumin) and the chemical attachment through glutaraldehyde (Shen et al. 2011a). DOX concentration influenced the size and polydispersity of albumin nanoparticles because DOX hydrochloride, which was used in experiments, dissociates in water into the protonized anthracycline and negatively charged chloride ions, and ionic compounds were previously shown to influence particle formation (Dreis et al. 2007). In this work, mean particle diameter increased with the rise in DOX concentration () as mentioned in the previous report (Dreis et al. 2007). An increase in DOX incorporation content was determined with the rise in DOX concentration, but nevertheless, PDI also dramatically increased (). Shen et al. (2011b) described that during the process of nanoparticle preparation, some amino groups of DOX were inevitably coupled to the amino groups of albumin molecules by GA, which was introduced to couple the amino groups of albumin molecules to enhance the stability of nanoparticles. Therefore, DOX-loaded nanoparticles are more hydrophobic than DOX-unloaded nanoparticles due to some hydrophobic DOX on the surface. The more hydrophobic surface may cause aggregation to some extent and render higher PDI values. The aggregation of DOX-loaded nanoparticles is not a problem for future applications when the PDI value is lower than 0.2 (Shen et al. 2011b). Thus, 1 mg/ml of DOX concentration was chosen for further synthesis. Size of nanospheres synthesized with 1 mg/ml of DOX was about 129.50 ± 14.80 nm, incorporation efficiency was 65.52 ± 4.6%, and PDI was 0.162 ± 0.010.
We investigated the effect of 1.5–7.5 mg/ml of magnetic powder concentration on nanoparticle size and incorporation content of DOX. While DOX incorporation efficiency was nearly the same (59–65%) at each Fe3O4 concentration, nanoparticle size increased slightly for 1.5–5 mg/ml. At that concentration range, hydrodynamic diameter of nanoparticles was about 210 nm; however, it increased to 348.0 ± 23.8 nm with 7.5 mg/ml concentration of Fe3O4 (seen in and ). A frequent problem with magnetic iron oxide nanoparticles is that they tend to aggregate forming strong magnetic dipole attractions between particles (Easo and Mohanan 2013). Thus, 7.5 mg/ml concentration was found as excess due to the aggregation leading to the formation of larger particles. Particles of > 200 nm are generally cleared via the reticuloendothelial system (Easo and Mohanan 2013). Depending on the reports, the ideal size requirements for nanoparticles developed for cancer treatment are between 70 and 200 nm (Gaumet et al. 2008). Therefore, we selected 5 mg/ml concentration as appropriate. In the final formulation, DOX/Fe3O4/BSA ratio is 1:5:25 (w/w/w). In addition, the ratio of the amount of our magnetic agent to the amount of albumin is almost similar to that used in an earlier study by Chen et al. (2010).
The SEM photographs of the empty, DOX-loaded and DOX-unloaded magnetic nanoparticles are shown in . Images revealed the formation of spherical nanoparticles with a narrow size distribution verified by zeta size measurements. Drug-loaded BSA nanoparticles that were spherical in shape had also been demonstrated by Merodio et al. (2001), Li et al. (2008) and Zhao et al. (2010). Naturally, on increasing the amount of iron oxide nanoparticles per latex, the chances of two magnetic latexes coming under the influence of magnetic field of each other increase (Gaihre et al. 2008). The final formulation of nanoparticles has a magnetic core which means that the magnetic field leads to the nanoparticles’ interaction with each other and to tolerable aggregation. Atomic force images of the DOX-loaded magnetic albumin nanoparticles revealed the spherical shape, smooth surfaces and the nearly uniform size distributions (). These results were in agreement with SEM pictures.
Figure 2. SEM images of (A) empty, (B) DOX-loaded and (C) DOX-unloaded magnetic albumin nanoparticles.
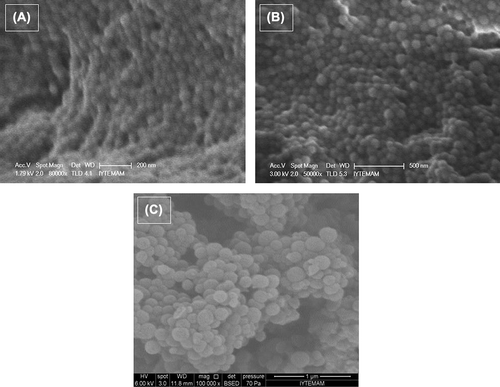
The XRD spectrum of nanoparticles is shown in . The spinel diffraction pattern was confirmed from the h k l indices detected. The XRD spectrum exhibited peaks corresponding to magnetic BSA nanoparticles marked with their indices (220), (311), (400), (511) and (440), which are similar to those reported previously for Fe3O4 (magnetite) nanoparticles (Jiang et al. 2004, Sun et al. 2006, Dung et al. 2009). The peaks at 30.168, 35.467, 43.167, 57.168 and 62.634 are the characteristic peaks of the Fe3O4 nanoparticles. Almost all of the typical crystal peaks of Fe3O4 were found in the sample of magnetic BSA nanoparticles. However, no typical crystal diffraction peaks of Fe3O4 were detected in the sample of BSA nanoparticles. Therefore, XRD pattern revealed that the prepared magnetic nanoparticles were pure Fe3O4.
The effective use of magnetic nanoparticles for biomedical applications such as targeted drug delivery depends on a number of factors related to the size and magnetism of the biocompatible nanoparticles. In addition, increasing the magnetization is advantageous to facilitate manipulation in drug delivery schemes(Chomoucka et al. 2010). Thus, besides the morphological characterization with SEM and AFM, magnetization test was carried out to determine its magnetic response to external magnetic field. The magnetic responsiveness of M-DOX-BSA nanoparticles was determined with a magnetometer. While magnetite nanoparticles had a magnetization value of 92 emu/g,the magnetization value of M-DOX-BSA nanoparticles was found experimentally to be 2.5 emu/g at room temperature when 500 G of magnetic field was applied. Magnetization value was calculated from magnetization curve of M-DOX-BSA nanoparticles which is shown in . It is known that when magnetic capsules are encapsulated with polymers, there is generally a marginal decrease in the value of magnetization (Zhang and Misra 2007). Therefore, it can be suggested that magnetite agent was coated with albumin and DOX-loaded magnetic albumin nanoparticles are responsive to magnetic field; moreover, these nanoparticles can be addressed to tumoral region with the aid of external magnetic field resulting in magnetic targeting.
In vitro drug release profiles
In order to evaluate the effect of GA amount on the release profile of the drug, three different (25, 75 and 100%) GA ratios were used after desolvation procedure. There was a decrease in the drug release with an increase in GA amount and with the ratio of 100% cross-linking DOX release was too slow(data not shown). As seen in , DOX-loaded nanospheres cross-linked with the ratio of 25% were allowed more desirable release rate than those cross-linked with the ratio of 75%. A similar result, in which increased GA amount led to a decrease in the release of drug because of the formation of denser polymer cross-links, in turn, leading to an increase in the diffusional path length that the drug molecules have to traverse, was also observed in an earlier report described by Thakkar et al. (2005). In addition, it could be suggested that GA forms amide bond between the amino group of DOX and the amino groups of lysine residues in BSA molecules. Dsouza and Topp (2004) explained that the hydrolysis rate constants of linkages between drugs and polymers depend on the chemical nature of the linkage, the structure of the polymer and the surrounding conditions. Amide bonds are very stable to aqueous hydrolysis at physiological pH.
Figure 6. In vitro release profile of DOX from (A) M-DOX-BSA nanoparticles cross-linked different GA ratio and (B) M-DOX-BSA nanoparticles in PBS medium with the absence or presence of proteolytic enzyme at pH 5, 6 and 7.4.
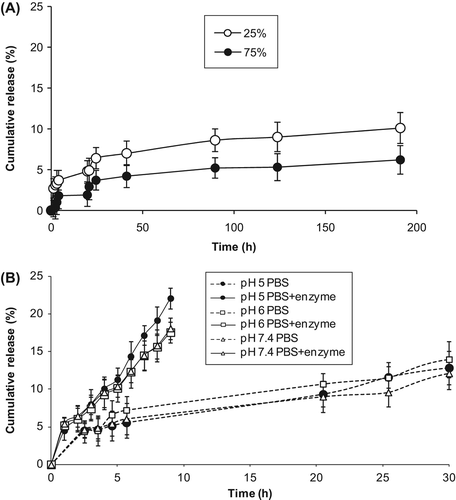
Similar and slow drug release rate of DOX from M-DOX-BSA nanoparticles was observed in pH 5, 6 and 7.4 medium; 5%–7% of DOX was released from M-DOX-BSA nanoparticles at the end of 5 h (), and 16%–18% of DOX was released at the end of 50 h (data not shown). The weak interactions of DOX with the nanoparticle form may be responsible for the initial DOX release. The latter release should be due to DOX fractions weakly interacted and covalently linked to the protein matrix via GA. It was clearly observed that DOX release from free form was faster than that of the nanoparticulate form, and about 60% of DOX was released in each PBS medium within 4 h of dialysis. Fast free DOX release profile was also determined in the literature (Yang et al. 2008, Gaihre et al. 2009). DOX release characteristics from M-DOX-BSA nanoparticles indicated that magnetic nanoparticle form of DOX could minimize its harmful side effects because of slower release rate of drug while reaching tumour tissue. Shen et al. (2008) explained that there are more enzymes in target sites (cancer cells or cancer tissues). So we used a proteolytic enzyme (chymotrypsin) which is able to degrade albumin, during release studies. Drug release results in the presence of enzyme are shown in . The addition of chymotrypsin to the release medium allowed the release of higher amount of DOX. Extra 5% of DOX release was observed in the presence of chymotrypsin compared with that in the absence of enzyme within 5 h of dialysis. On the other hand, about 10% of DOX was released in 20 h in the absence of enzyme but equal percentage was released in 5 h in the presence of enzyme. Increased drug release was determined using proteolytic enzyme in the release medium as described in the early works (Merodio et al. 2001, Shen et al. 2008, Gaihre et al. 2009, Shen et al. 2011a).
Conclusion
In this paper, anti-cancer agent-loaded magnetic albumin nanospheres were developed using a simple and cheap method. DOX was chosen as an anti-cancer drug because it has wide activity against a number of human neoplasms. Formulation was characterized for size, size distribution, morphology and magnetic responsiveness. According to the data, M-BSA-DOX nanoparticles have about 200 nm of hydrodynamic diameter and spherical shape. In addition, nanoparticles can be controlled by external magnetic field. Drug release characteristics suggest that DOX-loaded magnetic nanoparticles should have a potential for drug delivery with reduced damaging side effects, so these nanoparticles can be used as a vehicle for magnetically targeted cancer therapy with minimized required dosage of DOX. Future works include investigation of cytotoxicity of drug formulation against cancer cell lines and afterwards evaluation of targeting capability and effectiveness of these magnetic nanospheres under magnetic field in animal models.
Declaration of interest
The authors report no declarations of interest. The authors alone are responsible for the content and writing of the paper.
References
- Arruebo M, Fernandez-Pacheco R, Ibarra MR, Santamaria J. 2007. Magnetic nanoparticles for drug delivery. Nanotoday. 2:22–32.
- Bradford MM. 1976. A rapid and sensitive method for the quantitation of microgram quantities of protein utilizing the principle of protein-dye binding. Anal Biochem. 72:248–254.
- Brannon-Peppas L, Blanchette JO. 2004. Nanoparticle and targeted systems for cancer therapy. Adv Drug Deliver Rev. 56:1649–1659.
- Chen D, Tang Q, Xue W, Xiang J, Zhang L, Wang X. 2010. The preparation and characterization of folate-conjugated human serum albumin magnetic cisplatin nanoparticles. J Biomed Res. 24:26–32.
- Chomoucka J, Drbohlavova J, Huska D, Adam V, Kizek R, Hubalek J. 2010. Magnetic nanoparticles and targeted drug delivering. Pharmacol Res. 62:144–149.
- Danhier F, Feron O, Preat V. 2010. To exploit the tumor microenvironment: passive and active tumor targeting of nanocarriers for anti-cancer drug delivery. J Control Release. 148:135–146.
- Dreis S, Rothweiler F, Michaelis M, Cinatl J Jr, Kreuter J, Langer K. 2007. Preparation, characterization and maintenance of drug efficacy of doxorubicin-loaded human serum albumin (HSA) nanoparticles. Int J Pharmaceut. 341:207–214.
- Dsouza AJM, Topp EM. 2004. Release from polymeric prodrugs: linkages and their degradation. J Pharm SCI-US. 93:1962–1979.
- Dung DKT, Hai TH, Phuc LH, Long DB, Vinh LK, Truc PN. 2009. Preparation and characterization of magnetic nanoparticles with chitosan coating. J Phys Conf Ser. 187:1–5.
- Easo SL, Mohanan PV. 2013. Dextran stabilized iron oxide nanoparticles: synthesis, characterization and in vitro studies. Carbohydr Polym. 92:726–732.
- Elsadek B, Kratz F. 2012. Impact of albumin on drug delivery – New applications on the horizon. J Control Release. 157:4–28.
- Elzoghby AO, Samy WM, Elgindy NA. 2012. Albumin-based nanoparticles as potential controlled release drug delivery systems. J Control Release. 157:168–182.
- Gaihre B, Aryal S, Khil MS, Kim HY. 2008. Encapsulation of Fe3O4 in gelatin nanoparticles: effect of different parameters on size and stability of the colloidal dispersion. J Microencapsul. 25:21–30.
- Gaihre B, Khil MS, Lee DR, Kim HY. 2009. Gelatin coated magnetic iron oxide nanoparticles as carrier system: Drug loading and in vitro drug release study. Int J Pharmaceut. 365:180–189.
- Gaumet M, Vargas A, Gurny R, Delie F. 2008. Nanoparticles for drug delivery: the need for precision in reporting particle size parameters. Eur J Pharm Biopharm. 69:1–9.
- Jeong Y, Jin SG, Kim IY, Pei J, Wen M, Jung TY, et al. 2010. Doxorubicin-incorporated nanoparticles composed of poly(ethylene glycol)-grafted carboxymethyl chitosan and antitumor activity against glioma cells in vitro. Colloids Surf B Biointerfaces. 79:149–155.
- Jiang W, Yang HC, Yang SY, Horng HE, Hung JC, Chen YC, Hong CY. 2004. Preparation and properties of superparamagnetic nanoparticles with narrow size distribution and biocompatible. J Magn Magn Mater. 283:210–214.
- Kairemo K, Erba P, Bergström K, Pauwels EKJ. 2008. Nanoparticles in cancer. Curr Radiopharma. 1:30–36.
- Kratz F. 2008. Albumin as a drug carrier: Design of prodrugs, drug conjugates and nanoparticles. J Control Release. 132:171–183.
- Kumar C. 2006. Nanotechnologies for the life sciences: biological and pharmaceutical nanomaterials. In: Langer K, Ed. Peptide Nanoparticles, vol. 2. Weinheim: Wiley, pp. 145–184.
- Langer K, Balthasar S, Vogel V, Dinauer N, von Briesen H, Schubert D. 2003. Optimization of the preparation for human serum albumin (HSA) nanoparticles. Int J Pharmaceut. 257:169–180.
- Li FQ, Su H, Wang J, Liu JY, Zhu QG, Fei YB, et al. 2008. Preparation and characterization of sodium ferulate entrapped bovine serum albumin nanoparticles for liver targeting. Int J Pharmaceut. 349: 274–282.
- Li JM, Chen W, Wang H, Jin C, Yu XJ, Cui L, et al. 2009. Preparation of albumin nanospheres loaded with gemcitabine and their cytotoxicity against BXPC-3 cells in vitro. Acta Pharmacol Sin. 30: 1337–1343.
- Merodio M, Arnedo A, Renedo MJ, Irache JM. 2001. Ganciclovir-loaded albumin nanoparticles: characterization and in vitro release properties. Eur J Pharm Sci. 12:251–259.
- Min K, Jo H, Song K, Cho M, Chun YS, Jon S, et al. 2011. Dual-aptamer based delivery vehicle of doxorubicin to both PSMA(+) and PSMA(-) prostate cancers. Biomaterials. 32:2124–2132.
- Moses MA, Brem H, Langer R. 2003. Advancing the field of drug delivery: taking aim at cancer. Cancer Cell. 4:337–341.
- Qu X, Yang C, Zhang J, Ding N, Lu Y, Huang L, Xiang G. 2010. In vitro evaluation of a Folate-bovine serum albumin- doxorubicin conjugate. J Drug Target. 18:351–361.
- Reddy LH, Sharma RK, Murthy RSR. 2004. Enhanced tumour uptake of doxorubicin loaded poly(butyl cyanoacrylate nanoparticles in mice bearing dalton's lymphoma tumour. J Drug Target. 12:443–451.
- Shen Z, Wei W, Zhao Y, Ma G, Dobashi T, Maki Y, et al. 2008. Thermosensitive polymer-conjugated albumin nanospheres as thermal targeting anti-cancer drug carrier. Eur J Pharmaceut Sci. 35:271–282.
- Shen Z, Li Y, Kohama K, Oneill B, Bi J. 2011a. Improved drug targeting of cancer cells by utilizing actively targetable folic acid-conjugated albumin nanospheres. Pharmacol Res. 63:51–58.
- Shen Z, Wei W, Tanaka H, Kohama K, Ma G, Dobashi T, et al. 2011b. A galactosamine-mediated drug delivery carrier for targeted liver cancer therapy. Pharmacol Res. 64:410–419.
- Siegel R, Naishadham D, Jemal A. 2012. Cancer statistics. CA Cancer J Clin. 62:10–29.
- Singh R, Lillard JW. 2009. Nanoparticle based targeted drug delivery. Exp Mol Pathol. 86:215–223.
- Sun J, Zhou S, Hou P, Yang Y, Weng J, Li X, Li M. 2006. Synthesis and characterization of biocompatible Fe3O4 nanoparticles. J Biomed Mater Res A. 80A:333–341.
- Thakkar H, Sharma RK, Mishra AK, Chuttani K, Murthy RR. 2005. Albumin microspheres as carriers for the antiarthritic drug celecoxib. AAPS PharmSciTech. 6:65–73.
- Wagner S, Rothweiler F, Anhorn MG, Sauer D, Riemann I, Weiss EC, et al. 2010. Enhanced drug targeting by attachment of an anti αv integrin antibody to doxorubicin loaded human serum albumin nanoparticles. Biomaterials. 31:2388–2398.
- Widder KJ, Senyei AE, Ranney DF. 1980. In vitro release of biologically active adriamycin by magnetically responsive albumin microspheres. Cancer Res. 40:3512–3517.
- Yang X, Chen Y, Yuan R, Chen G, Blanco E, Gao J, Shuai X. 2008. Folate-encoded and Fe3O4-loaded polymeric micelles for dual targeting of cancer cells. Polymer. 49:3477–3485.
- Zhang J, Misra RDK. 2007. Magnetic drug-targeting carrier encapsulated with thermosensitive smart polymer: Core–shell nanoparticle carrier and drug release response. Acta Biomaterialia. 3:838–850.
- Zhao D, Zhao X, Zu Y, Li J, Zhang Y, Jiang R, Zhang Z. 2010. Preparation, characterization, and in vitro targeted delivery of folate-decorated paclitaxel-loaded bovine serum albumin nanoparticles. Int J Nanomed. 5:669–677.