Abstract
Outgrowth of collateral sprouts from axons is a natural process that arises during development of and regeneration in the peripheral nervous system. Our previous study showed that if there are enough distal endoneurial tubes into which the proximal regenerative axons can grow, one axon can support three to four collaterals, at most. Here, the proximal half tibial nerve was fixed to the distal stump and served as the donor nerve. The number of myelinated axons was calculated after 4 months. The ratio of distal regenerative myelinated axon number to proximal donor nerve axon number was 1.83 with the tibial function index and the nerve conduction velocities of − 48.6 ± 6.8 and 27.8 ± 5.3 m/s. The regenerated collaterals were isolated and observed to sprout from the node of Ranvier with almost the same features of normal fibers, but with different electrophysiological characteristics. This finding shows the evidence of one-axon trunk several-collateral model in peripheral nerve regeneration and suggests that such multi-collateral regeneration model may be useful in peripheral nerve reconstruction.
Introduction
Outgrowth of collateral sprouts from afferent and motor axons is a natural process that arises during development of and regeneration in the peripheral nervous system (Belzberg and Campbell Citation1998, Kostakoglu Citation1999, Maldonado and Barker Citation2003, McCallister et al. Citation1999, Oberlin et al. Citation1994). When a nerve is injured, the proximal stump of damaged axons sends out numerous sprouts to reach endoneurial tubes in the distal nerve stump. In this process, some sprouts remain viable, while others may go astray, eventually being eliminated. The residual axons in the proximal stump and the endoneurial tubes, where the regenerative axons can grow into the distal nerve stump, may greatly influence the number and quality of regenerative axons that grow into the target tissue, and thus determine the reconstructive effect.
In our previous study, we found that if there are enough distal endoneurial tubes into which the proximal regenerative axons can grow, the regenerative axon will sprout and maintain the most possible collaterals. One axon can support three to four collaterals, at most (Jiang et al. Citation2007, Yin et al. Citation2011a, Citation2011b). However, the location of collateral sprouting and the difference between regenerative axon trunks and new collaterals remain controversial. Answering these questions will greatly help us understand the regeneration of peripheral nerve and may even help us to find new ways (Amr and Moharram Citation2005, Evans et al. Citation1999, Kostakoglu Citation1999, Madihally and Matthew Citation1999, Matsumoto et al. Citation2000a, Citation2000b, McCallister et al. Citation1999, Okajima and Terzis Citation2003, Tung and Mackinnon Citation2001, Vasconcelos and Gay-Escoda Citation2000, Viterbo et al. Citation1994) to reconstruct peripheral nerve and reduce loss of muscle function, impaired sensation, and/or painful neuropathies (Chuang Citation1995, Dahlin and Lundborg Citation2001, Evans et al. Citation1999, Frostick et al. Citation1998, Seckel Citation1990, Sungpet et al. Citation2000, Tung and Mackinnon Citation2001). Thus, in the present study, we focus on the morphological characteristics of the collaterals sprouted through one axon during regeneration of rat peripheral nerve.
Materials and methods
Animals
We used male Sprague-Dawley rats that weighed 200–250 g and that were maintained under specific pathogen-free laboratory conditions. The rats were separated into two groups at random (six animals in each group). Here, every effort was made to minimize animal suffering and reduce the number of animals used, according to the Chinese guidelines for care and use of laboratory animals.
Surgical procedures
Surgical procedures were carried out under a surgical microscope using a microsurgical technique. Rats were anesthetized with sodium pentobarbital (30 mg/kg i.p.). After anesthesia and sterilization, the left sciatic nerve and the common peroneal nerve and the tibial nerve were exposed. Then, to provide a proximal tibial nerve with half numbers of axons, a 3-mm-long nerve segment with about half diameters was removed from the stem of the tibial nerve at a level 8 mm distal to the bifurcation of the sciatic nerve. The proximal end of the excised segments in the tibial nerve residual was ligated with 10-0 nylon sutures. The precise axon number was calculated 4 months later by histological methods. The tibial nerve was then transected and the proximal 1/2 tibial nerve residual served as the donor nerve and was fixed together with the distal stump with 10-0 nylon microsutures. For this, we used biodegradable chitin conduits (patented by our laboratory and authorized by the State Intellectual Property Office of China No. ZL01 136314.2; It was an artificial nerve graft consisting of shell polysaccharide that showed satisfactory biocompatibility and degradation characteristics. This conduit is now in a preclinical study). The gap between the two nerve segments was kept at 1 mm. The biodegradable chitin conduits were 1 cm long, 0.1 mm thick, with an inner diameter of 1.5 mm. Subsequently, the wound was closed using 4-0 nylon sutures (). The control group underwent a sham operation involving full exposure of tibial nerve and subsequent wound closure.
Electrophysiological study
Prior to sacrifice of the animals, the repaired tibial nerve was exposed, and stimulating bipolar electrodes were placed proximal and distal to the repair site. The recording electrode was placed in the gastrocnemius muscle, while the ground electrode went subcutaneously, between the stimulating and recording electrodes. Rectangular pulses (duration 0.1 ms, 0.9 mA, 10 Hz, six continual stimuli) were used to stimulate the repaired tibial nerves. Compound muscle action potential was recorded and nerve conduction velocity (NCV; m/s) was obtained semiautomatically by dividing the distance between the two stimulating sites by the difference in the onset latency. The NCV of the tibial nerve in sham control was recorded similarly.
Isolation of single nerve fiber
Four months after surgery, bifurcated nerve segments at the neurorrhaphy site were harvested and treated according to the method described by Lascelles and Thomas (Lundborg et al. Citation1994). The specimens were fixed with 2.5% glutaraldehyde and 4% paraformaldehyde overnight and then postfixed with 1% osmium tetroxide. After being rinsed thoroughly with distilled water, they were macerated in glycerol solution (glycerol: distilled water 2:1 by volume) for 24 h, then transferred to pure glycerol. Under an operating microscope working at 20× magnification (Carl Zeiss, Germany) the epineurium and perineurium were stripped, and the connective tissue was removed with microsurgical instruments. Thereafter, nerve fibers were teased out and observed under light microscopy (Olympus, Japan).
Histological study
The entire tibial nerve, including the repaired segment, was removed en bloc from each rat. Tissues were then harvested and fixed in 4% paraformaldehyde in 0.1 M phosphate buffer for 12 h at 4°C. After that, the nerves were rinsed twice in phosphate buffer, and three tissue blocks (approx. 5 mm long) were cut, one proximal to the residual segment, one distal to the residual segment, and one from the residual segment. After this step, each sample was postfixed in 1% osmium tetroxide for 6 h, dehydrated through a graded series of ethanols and embedded in paraffin. Specimen sections were then taken for osmium acid staining of the entire nerve perpendicular to the long axis of the nerve fibers. Myelinated axons were quantified according to the unbiased counting rule. Finally, the total number of myelinated axons was estimated by multiplying the axonal density by the total cross-sectional area of the whole nerve in each animal.
Statistical analysis
Student's t-test was employed to compare the number of myelinated nerve fibers and NCV in all groups. p < 0.05 was considered significant for all statistical comparisons. All values are presented as the mean ± SD.
Results
No animal died during the 4 months of follow-up monitoring. After exposure of the tibial nerve, the biodegradable chitin conduits were clearly identifiable under the surgical microscope. The results of quantitative stereological evaluation of three different nerve segments (proximal, mid-segment, and distal) of different groups are summarized in . The single axon count for the sham control group was recorded for comparison. The myelinated fibers of various diameters were observed in the reconstructive group. In some rats wallerian-type degeneration was observed in addition to axonal regeneration in the distal part of the tibial nerve.
Table I. Comparison of myelinated axon numbers for all groups.
Electrophysiological assessment was conducted prior to the sacrifice of the animals. The motor NCV of the tibial nerve in sham control group was 49.5 ± 4.9 m/s. And the NCV of the distal tibial nerve segment in experimental group was 27.8 ± 5.3 m/s ().
Figure 2. Electrophysiological recordings of the compound muscle action potential of gastrocnemius muscles 4 months after operation. The motor NCV of the tibial nerve in the sham control group was 49.5 ± 4.9 m/s (A) while the value of the distal tibial nerve segment in the experimental group was 27.8 ± 5.3 m/s (B).

Histomorphometric evaluation revealed that the number of axons of the proximal donor nerve (mid-segment residual of the tibial nerve) ranged from approximately 1/2.37 to 1/1.74 the number of axons of the whole tibial nerve with an average value of about 1/2. The mean regenerative number of axons in the distal segment was greater than that of the donor axons of the mid segment. The amplifying ratio of the regenerative axons (the ratio of the distal regenerative axon numbers to the proximal donor axon numbers) was 1.83, while the ratio of distal nerve stump endoneurial tubes to proximal donor nerve axon numbers (RDP) was 1.97 (, ).
Figure 3. Histological sections through the reconstructed nerves 4 months after surgery. A: The proximal part of the tibial nerve (normal tibial nerve). B: The distal part of the tibial nerve was repaired with 1/2 number of proximal donor axons (regenerated tibial nerve).
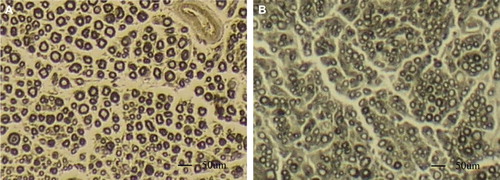
After fixation with osmium tetroxide, nerve specimens appeared dark black. After immersion in glycerol solution for 24 h, the fixed nerves became soft and loose. The epineurium and perineurium were easily stripped off. After removal of the connective tissue, tibial nerve fibers were separated, and single fibers were isolated. Examination under a light microscope revealed that axons sprouted collaterally from the myelinated nerve fiber at the node of Ranvier (). The regenerative collateral fibers in the distal side were a little thinner than the proximal trunk. The node of ranvier where the collaterals sprout was bigger than its normal size. There was no significant difference between the two regenerative collateral fibers in their appearance.
Figure 4. Myelinated nerve fiber isolated from the tibial nerve. A: single fiber isolated from the tibial nerve in sham control group; B: Regenerated fiber at the site of suture with biodegradable chitin conduits. A myelinated axon sprouts collateral branches at the node of Ranvier. (Observed with light microscopy working at 100× magnification).
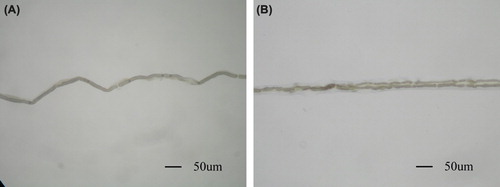
Discussion
In this research, we focused on the morphological characteristics of the collaterals sprouted through one axon during regeneration of rat peripheral nerve and evaluated the recovery of nerve function of the peripheral nerve reconstructed with these collaterals. We found that if there are enough distal endoneurial tubes into which the proximal regenerative axons can grow, the regenerative axon will sprout and maintain the most possible collaterals. The regenerated collaterals sprouted from the node of Ranvier with almost the same features of normal fibers but different electrophysiological characteristics. Such one-axon trunk several-collateral model might be a common mechanism during the regeneration of peripheral nerve.
During development, it has been shown that axons send off many collaterals that grow into the target tissue. Those sprouts extend through their own basal lamina tubes in the proximal segment, traverse the narrow gap of connective tissue between the proximal and distal stumps, and finally enter the distal nerve segment. As described previously, during the regenerating, sprouts which cannot grow into the distal endoneurial tubes gradually degenerate. These sprouts include those blocked by scar tissue, connective tissue and other kinds of obstructions as well as sprouts that grow in the wrong direction. Therefore, when axons establish terminal structures, the number of collaterals is reduced to the usual single trunk. Based on our findings, the number of endoneurial tubes in the distal stump is the most important factor influencing the sprouting of regenerative axons. The amplifying phenomenon of the regenerative axons only arose in nerves in which the number of distal endoneurial tubes was greater than that of the proximal donor axons. Sprouting is the nature of a peripheral nerve. When the integrity of the peripheral nerve is broken, including the interruption of axon continuity, that is the rupture of the endoneurium, perineurium and epineurium, the axons will then sprout and send out collaterals. If there are enough distal endoneurial tubes into which the proximal regenerative axons can grow, the regenerative axon will sprout and maintain the most possible collaterals. However, due to limitations of cell metabolism, there is not an adequate supply of necessary constituents for too many sprouts to grow from a single axon. Thus, there might be a limit to the number of regenerative collaterals from one axon. According to our previous study, one axon can support three to four collaterals, at most (Yin et al. Citation2011a, Sungpet et al. Citation2000, Lundborg et al. Citation1994). Here the number of distal endoneurial tubes into which the regenerative axons can grow is about twice that of the proximal donor axons. The amplifying ratio of the regenerative axons was about 1.83. That means most axons of the donor nerve regenerate and maintain one to two collaterals in the regenerative distal stump. Results here were similar with what we got earlier.
Many studies have been reported to demonstrate the collateral sprouting of the peripheral nerve. However, the exact location of collateral sprouting is unclear. In present study, we found the sprouting appeared to originate at the node of Ranvier of a myelinated nerve fiber. The location of collateral sprouting represents the active region where the donor nerve responds to the change in the nerve reconstruction. Identifying the location of collateral sprouting might help to further understand the mechanism of a neuron to receive a message and send impulses through such one-axon trunk several-collateral model.
Electrophysiological experiments showed the NCV of the distal regenerative nerve was slower than that of the nerves in the control group. This result tallied with the morphological characteristics of the regenerative collaterals. These collateral fibers in the distal side were a little thinner than the proximal trunk. That might be the reason for the smaller conductivity of the neogenic collaterals. At the same time, the action potential waveform of the gastrocnemius muscle changed to irregular after operation. The waveform had one peak in the sham control group, while most had more than one peak after surgery. A single-peak waveform reflects synchrony of gastrocnemius muscle depolarization, while the multipeak waveform reflects asynchrony of depolarization. In other words, after reconstructive surgery, the stimuli exciting the gastrocnemius muscles arrived at the nerve–muscle junction at different times. These results suggest that there is heterogeneity of the electrophysiological properties among the regenerative axons. The regenerative collaterals might have different electrophysiological characteristics due to the regenerative axon trunks. Although the NCV was different, it was seen that the compound muscle action potential was similar to each other between control and experimental animals. How did this happen? We supposed that effective reinnervation was constructed in most muscle fibers. Impulses transferred along the axon trunk and passed to the collaterals through the node of Ranvier at the sprouting site. As the all-or-none law of nerve physiology, collaterals below the node of Ranvier were fired when the impulses depolarized the axon trunk at the sprouting site. Finally, these signals arrived to the neuromuscular junction and triggered muscle contraction. This might be the signal transmission mechanism of such one-axon trunk several-collateral model. The regenerative collaterals had different electrophysiological characteristics to the axon trunk especially in nerve conductivity.
Next, what was the physiological function of these regenerative collaterals? Could these collaterals be used to reconstruct peripheral nerve injury? Here, all animals that underwent reconstructive surgery recovered tibial nerve function in some level. As we reported previously, proximal whole median nerve and even half of it were served as donor nerve to repair the distal donor site and whole ulnar nerve simultaneously (Sungpet et al. Citation2000, Lundborg et al. Citation1994). In both of these experiments, recovery of the reinnervated muscles was observed. In all these studies, the one-axon trunk several-collateral model was participated in the reinnervation mechanism. Meanwhile, partial denervation of an effector may not influence its physiological functioning greatly. Thus, it is practicable to separate partial axons from an intact nerve and still maintain most of the functions of the tissue innervated by the donor nerve. Altogether, we suggest that such multicollateral regeneration model may be useful in peripheral nerve reconstruction.
However, the assessment of the function recovery in this experiment was based on the histological and electrophysiological methods. Although the slices showed the successful regeneration of the distal injured nerve and electrical stimulation responded with contraction of the reinnervated muscles, it could only be a proof of structural reinnervation. Axonal pruning is commonly considered to be at the basis of the functional adaptation to the new connections. The functional recovery needs not only the connection between the peripheral nerve and its effectors, but also the effective control from the central nerve system on the peripheral reinnervated effectors. It is obvious that when collaterals were used to repair the injured nerve, the original father nerve would control not only the effectors innervated by itself, but also control other effectors after reconstruction. Sometimes these effectors may have opposite functions like antagonistic muscles in the locomotive system. So, brain reorganization related to major changes in the peripheral connections is a more important neuroscientific issue for the real function recovery of the reconstructed nerves. What happens to the central neuronal circuitries? What are the brain plasticity mechanisms for adapting to environmental perturbations? Evidences from the present experiment could not give a good answer to these questions.
Declaration of interest
The authors report no declarations of interest. The authors alone are responsible for the content and writing of the paper.
This work was supported by a grant from Chinese National Natural Science Youth Fund (31100860), Chinese National Natural Science Fund (30971526, 31171150), Chinese National Natural Science Fund (81171146).
References
- Amr SM, Moharram AN. 2005. Repair of brachial plexus lesions by end-to-side side grafting neurorrhaphy: experience based on 11 cases. Microsurgery. 25:126–146.
- Belzberg AJ, Campbell JN. 1998. Evidence for end-to-side sensory nerve regeneration in a human. Case report. J Neurosurg. 89: 1055–1057.
- Chuang DC. 1995. Neurotization procedures for brachial plexus injuries. Hand Clin. 11:633–645.
- Dahlin LB, Lundborg G. 2001. Use of tubes in peripheral nerve repair. Neurosurg Clin N Am. 12:341–352.
- Evans GR, Brandt K, Widmer MS, Lu L, Meszlenyi RK, Gupta PK, et al. 1999. In vivo evaluation of poly(L-lactic acid) porous conduits for peripheral nerve regeneration. Biomaterials. 20:1109–1115.
- Frostick SP, Yin Q, Kemp GJ. 1998. Schwann cells, neurotrophic factors, and peripheral nerve regeneration. Microsurgery. 18: 397–405.
- Jiang BG, Yin XF, Zhang DY, Fu ZG, Zhang HB. 2007. Maximum number of collaterals developed by one axon during peripheral nerve regeneration and the influence of that number on reinnervation effects. Eur Neurol. 58:12–20.
- Kostakoglu N. 1999. Motor and sensory reinnervation in the hand after an end-to-side median to ulnar nerve coaptation in the forearm. Br J Plast Surg. 52:404–407.
- Lundborg G, Dahlin L, Danielsen N, Zhao Q. 1994. Trophism, tropism, and specificity in nerve regeneration. J Reconstr Microsurg. 10:345–354.
- Madihally SV, Matthew HW. 1999. Porous chitosan scaffolds for tissue engineering. Biomaterials. 20:1133–1142.
- Maldonado CJ, Barker JH. 2003. The use of end-to-side nerve grafts to reinnervate the paralyzed orbicularis oculi muscle. Plast Reconstr Surg. 111:2255–2264.
- Matsumoto K, Ohnishi K, Kiyotani T, Sekine T, Ueda H, Nakamura T, et al. 2000a. Peripheral nerve regeneration across an 80-mm gap bridged by a polyglycolic acid (PGA)-collagen tube filled with laminin-coated collagen fibers: a histological and electrophysiological evaluation of regenerated nerves. Brain Res. 868: 315–328.
- Matsumoto K, Ohnishi K, Sekine T, Ueda H, Yamamoto Y, Kiyotani T, et al. 2000b. Use of a newly developed artificial nerve conduit to assist peripheral nerve regeneration across a long gap in dogs. AsaioJ46:415–420.
- McCallister WV, Tang P, Trumble TE. 1999. Is end-to-side neurorrhaphy effective?A study of axonal sprouting stimulated from intact nerves. J Reconstr Microsurg. 15:597–603.
- Oberlin C, Beal D, Leechavengvongs S, Salon A, Dauge MC, Sarcy JJ. 1994. Nerve transfer to biceps muscle using a part of ulnar nerve for C5-C6 avulsion of the brachial plexus: anatomical study and report of four cases. J Hand Surg Am. 19:232–237.
- Okajima S, Terzis JK. 2003. Ultrastructure of early axonal regeneration in an end-to-side neurorrhaphy model. J Reconstr Microsurg. 16:313–323.
- Seckel BR. 1990. Enhancement of peripheral nerve regeneration. Muscle Nerve. 13:785–800.
- Sungpet A, Suphachatwong C, Kawinwonggowit V, Patradul A. 2000. Transfer of a single fascicle from the ulnar nerve to the biceps muscle after avulsions of upper roots of the brachial plexus. J Hand Surg Br. 25:325–328.
- Tung TH, Mackinnon SE. 2001. Flexor digitorum superficialis nerve transfer to restore pronation: two case reports and anatomic study. J Hand Surg Am. 26:1065–1072.
- Vasconcelos BC, Gay-Escoda C. 2000. Facial nerve repair with expanded polytetrafluoroethylene and collagen conduits: an experimental study in the rabbit. J Oral Maxillofac Surg. 58:1257–1262.
- Viterbo F, Trindade JC, Hoshino K, Mazzoni A. 1994. Two end-to-side neurorrhaphies and nerve graft with removal of the epineural sheath: experimental study in rats. Br J Plast Surg. 47: 75–80.
- Yin XF, Kou YH, Wang YH, Zhang P, Zhang DY, Fu ZG, et al. 2011b. Can “dor to dor+ rec neurorrhaphy” by biodegradable chitin conduit be a new method for peripheral nerve injury?Artif Cells Blood Substit Immobil Biotechnol. 39:110–115.
- Yin XF, Kou YH, Wang YH, Zhang P, Zhang HB, Jiang BG. 2011a. Portion of a nerve trunk can be used as a donor nerve to reconstruct the injured nerve and donor site simultaneously. Artif Cells Blood Substit Immobil Biotechnol. 39:304–309.