Abstract
In the biosensor construction, 3-mercaptopropionic acid (3-MPA) and 6-aminocaproic acid (6-ACA) were used for forming self-assembled monolayer (SAM) on a gold disc electrode and pyruvate oxidase was immobilized on the modified electrode surface by using glutaraldehyde. Biosensor response is linearly related to pyruvate concentration at 2.5–50 μM, detection limit is 1.87 μM and response time of the biosensor is 6 s for differential pulse voltammograms. From the repeatability studies (n = 6) for 30.0 μM pyruvate revealed that the average value (), standard deviation (S.D) and coefficient of variation (CV %) were calculated to be 31.02 μM, ± 0.1914 μM and 0.62%, respectively.
Introduction
Pyruvate is a very important molecule in glycolysis, the tricarboxylic acid cycle and some metabolic processes (Situmorang et al. Citation2002). Determination of the pyruvate is especially important in the clinical, bioprocess, and food analysis. Pyruvate oxidase (POX)-based electrochemical biosensors have been used for this aim (Gajovic et al. Citation1999, Ghica and Brett Citation2006, Akyilmaz and Yorganci Citation2007). According to the enzymatic reaction given below POX uses oxygen and phosphate for catalyzing oxidative decarboxylation of pyruvate to acetyl phosphate and hydrogen peroxide.
For the catalyzing reaction, POX also required some cofactors such as flavin adenine dinucleotide (FAD), thiamine pyrophosphate (TPP), and magnesium which is needed for catalytic activity of enzyme (Muller et al. Citation1994, Muller and Schulz Citation1993). Different types of biosensors have been developed for the determination of pyruvate. Researchers have utilized different strategies and also transducer systems in the construction of these biosensors. An amperometric biosensor based on oxygen electrode (Kubo et al. Citation1991), a bienzyme-modified carbon paste electrode for amperometric detection (Bergmann et al. Citation1999), an amperometric biosensor with a polyazetidine prepolymer matrix (Mascini and Mazzei Citation1987), oxygen electrode combined with a porous acetyl cellulose membrane (Mizutani et al. Citation1980), gold microelectrodes (Revzin et al. Citation2002), oxygen electrode based on chemical covalent immobilization of POX (Zapata-Bacri and Burstein Citation1987-1988), a polyion complex membrane systems (Mizutani et al. Citation2000) have been developed for the determination of pyruvate. In addition, some biosensors have been developed for the determination of phosphate such as bilayer membrane systems with a poly (vinyl alcohol)/polyion complex (Mak et al. Citation2003), a screen-printed electrode (Kwan et al. Citation2005), and a porous conductive carbon electrode in conjunction with a polyelectrolyte stabilized recombinant POX (Gavalas and Chaniotakis Citation2001).
Self-assembled monolayer systems (SAMs) provide a convenient, flexible, and simple system to tailor the interfacial properties of metal electrodes, especially gold electrodes. The molecules or ligands that form the SAMs have a chemical functionality, or “head group”, with a specific affinity for a substrate. This head group has a high affinity for the surface and displaces adsorbed random organic materials from the surface (Love et al. Citation2005). Variation of the end group of the monolayers determines the chemical behavior of the modified surface, which is essential in the design of new interfaces with more interesting properties. Terminal groups such as carboxylic acid or amine groups are the most employed to perform reactions that normally bounds proteins on SAM thought their amino acids residues (Mendes et al. Citation2008).
In this study we have developed a new biosensor based on POX for the determination of pyruvate. 3-mercaptopropionic acid (3-MPA) which have functional compound linked with sulfide have been synthesized to form the SAM on gold electrode. Modified gold disc electrode with 3-MPA molecular structures of disulfide linked derivatives separated by the spacer 6-aminocaproic acid (6-ACA). Finally, POX was immobilized on the modified electrode surface by using cross-linking agent, glutaraldehyde.
Materials and methods
Chemical and reagents
POX (bacterial; E.C1.2.3.3), 3-MPA, 6-ACA, sodium pyruvate, potassium hexacyanoferrate(III) (K3Fe(CN)6), glutaraldehyde, and all other chemicals were purchased from Sigma-Aldrich (St. Louis, MO, USA). All chemicals and solvents used were of analytical grade. Ultra-pure water was used in the preparation of the solutions used in the experiments. The prepared solutions were kept at 4°C before use.
Apparatus
Measurements were carried out with a PalmSens Instruments system (the Netherland). A three-electrode system was used containing a platinum wire auxiliary electrode (CHI 115 model), a saturated Ag/AgCl reference electrode (CHI 111 model) and the gold working electrode (2 mm dia.) (CHI 101 model). The pH measurements were made with a pH meter (Hanna Instruments HI 221 –Italy). Sonication was made with an Ultrasonic LC 30 (Germany). Ultra-pure water was obtained with a Milipore Corporation Mili-Q system (USA).
Preparation of the pyruvate biosensor and measurements
Prior to coating, Au electrode (AuE) surface was first polished with 1.0 and 0.3 μm alumina slurry on microfiber cloth to obtain a mirror surface. After that, it was thoroughly rinsed with water and sonicated first in absolute ethanol and then in bidistilled water for 10 min to remove adsorbed particles, and then allowed to dry at room temperature. Finally, the electrode was transferred to an electrochemical cell for cleaning by cyclic voltammetry (CV) between − 1.0 and + 1.0 V potentials vs. Ag/AgCl at 50 mVs− 1 in 0.1 M HCl until a stable CV profile was obtained. 3-MPA/ 6-ACA-SAMs were formed by immersion of the clean gold disc electrode in the 25 mM 3-MPA solution for 4 h and then in the 5 mM 6-ACA solutions for 2 h. After that the modified electrode was rinsed with double-distilled water and allowed to dry at room temperature. Ten microliter of POX (0.356 UmL− 1; in phosphate buffer, pH 7.0, 50 mM) was dropped on to the 3-MPA/6-ACA modified gold electrode surface and was dried in the room temperature and the obtained electrode was carefully washed with ultra-pure water. Finally the electrode was immersed in 2.5% glutaraldehyde solution (GA) (in phosphate buffer, pH 7.0; 50 mM) for 2.5 min at 25°C.
shows electrochemical detection of pyruvate is based on the catalytic reaction of POX enzyme. The measurement was performed in the presence of an electron mediator potassium hexacyanoferrate(III). In the presence of POX and its cofactor FAD pyruvate was oxidized into acetyl phosphate while FAD was reduced to FADH2 which subsequently reduced from Fe (CN)64− to Fe(CN)63−. Pyruvate was determined by using differential pulse voltammograms (DPVs) at potential range between − 0.3 and 0.6 V in 5.0 mM potassium hexacyanoferrate(III) prepared in 50 mM phosphate buffer (pH 6.0 and 0.1 M KCl). Experimental solutions used in the reaction cell were purged with highly purified nitrogen gas for at least 5 min to remove dissolved oxygen.
Results and discussion
Electrochemical characterization of pyruvate biosensor by CVs
CV measurements can provide useful information on the changes of the electrode surface during the fabrication process. It brings very important information to investigate the changes of electrode behavior after each assembly step. The electrochemical behavior of bare, 3-MPA/ 6-ACA-modified and 3-MPA/6-ACA/POX/GA-modified gold electrodes were studied in 5 mM potassium hexacyanoferrate (III) prepared in 50 mM phosphate buffer (pH 6.0 and 0.1 M KCl), CVs were carried out at a potential range between − 0.4 and 0.5 V. shows CVs obtained for the bare AuE (a), AuE/3-MPA/6-ACA (b), AuE/3-MPA/ 6-ACA/POX/GA in the absence of pyruvate (c), and AuE/ 3-MPA/6-ACA/POX/GA in the presence of pyruvate (d). Bare AuE showed a characteristic reversible redox peaks resulted from ferricyanide. There was no any clear redox peaks by AuE/3-MPA/6-ACA-modified electrode because of barrier effect of SAM surface on the electrode. AuE/3-MPA/6-ACA/POX/GA in the absence of pyruvate showed slight peaks at around + 0.25 V and + 0.05 V potentials. Therefore, AuE/3-MPA/6-ACA/POX/GA-modified electrode displayed a pair of clear redox peaks at + 0.3 V and at nearly + 0.05 V potentials related to enzymatic reaction in the presence of pyruvate. The anodic peak current is nearly equal to the cathodic peak current. The peak potential separation (DEp) was 295 mV. So, the electrochemical reaction is a surface-controlled process.
Figure 1. Cyclic voltammograms of bare AuE (a), AuE/3-MPA/6-ACA (b), AuE/3-MPA/6-ACA/POX/GA in the absence of pyruvate (c) and AuE/ 3-MPA/6-ACA/POX/GA in the presence of pyruvate (d) in phosphate buffer (pH 6.0, 50 mM containing 0.1 M KCl and 5.0 mM K3Fe(CN)6) at a scan rate of 50 mVs− 1 vs. Ag/AgCl.
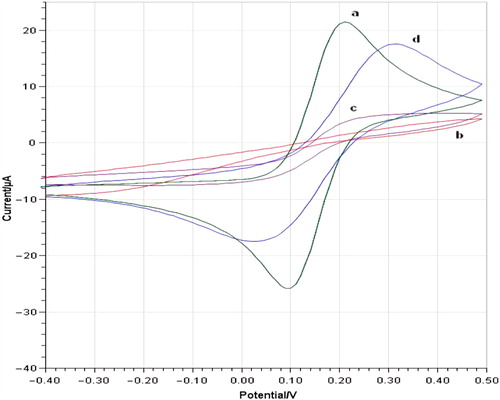
Linear range
In order to obtain calibration curves for pyruvate two different methods, amperometric and DPVs, were used in the experiments. The calibration curves obtained for the pyruvate are displayed in and . DPVs obtained for different concentrations of pyruvate were given in . When we consider the , it can be said that the biosensor responses depended linearly on pyruvate concentration between 2.5 and 50 μM with (y = 0.8889x + 13.739) and R2 = 0.9976. The detection limit of the biosensor is 1.87 μM. shows the amperometric results obtained from the experiments for different pyruvate concentrations. The biosensor responses depend linearly on pyruvate concentration between 1.58 and 10.71 μM with a (y = 0.3894x) and R2 = 0.9969. The detection limit of the biosensor is 0.56 μM. Both methods give sensitive results for pyruvate detection. A wide concentration range was obtained by DPVs method for pyruvate but better detection limit was carried out by amperometric method.
Figure 2. DP Voltammograms obtained for different concentration of pyruvate; a (0 μM), b (2.5 μM), c (10 μM), d (20 μM), e (30 μM), f (40 μM), g (50 μM). Inset shows the relationship between catalytic currents and pyruvate concentrations. Conditions: pH 6.0, 50 mM phosphate buffer (containing 0.1 M KCl and 5.0 mM K3Fe(CN)6) at 30°C. The percentage of glutaraldehyde and the activity of pyruvate oxidase were kept constant to be 2.5% and 0.356 UmL− 1, respectively.
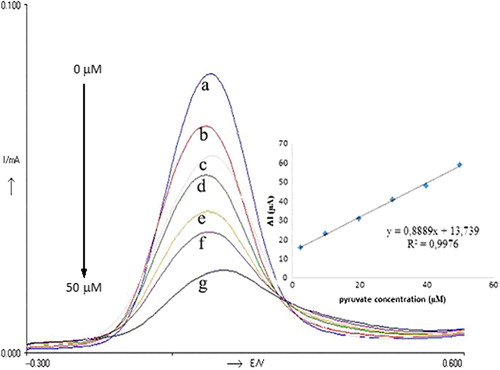
Figure 3. Current–time curves obtained with the biosensor upon successive addition of 1 mL 30 μM pyruvate. Inset shows the relationship between catalytic currents and pyruvate concentrations. Conditions: pH 6.0, 50 mM phosphate buffer (containing 0.1 M KCl and 5.0 mM K3Fe(CN)6) at the applied potential of 30 mV. The percentage of glutaraldehyde and the activity of pyruvate oxidase were kept constant to be 2.5% and 0.356 UmL− 1, respectively.
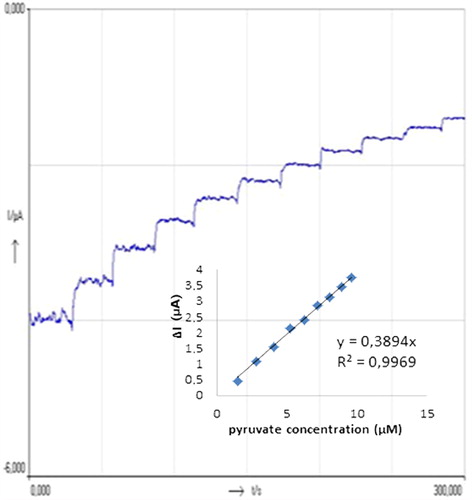
Effect of the activity of pyruvate oxidase, duration of SAM formation, and working pH on biosensor response
The activity of enzyme can affect the sensitivity and also response time of the biosensor. To detect the effect of the enzyme activity on the biosensor response different activity of POX were used in the construction of the biosensor. For this purpose, three biosensors were prepared. The biosensors contained 0.178, 0.356, and 0.712 UmL− 1 activities of POX. In the experiments amperometric measurements were made at 70 mV potential. The measurements were made to obtain standard curves for pyruvate by using the biosensors prepared. The most useful calibration curve was obtained by using the biosensor, which was prepared with 0.356 UmL− 1 activity of pyruvate oxidase. When the POX activity was decreased from 0.356 to 0.178 UmL− 1, lower biosensor responses were obtained. When the activity of POX was increased from 0.356 to 0.712 UmL− 1, higher biosensor responses were obtained but there was no a suitable calibration curve for pyruvate. From the results it can be said that, the most suitable biosensor responses and also linear calibration curves were obtained by using 0.356 UmL− 1 activity of POX.
The density of hydrocarbon chains was assigned by the duration of SAM deposition. For the investigation of the duration of SAM formation the electrodes were immersed in 25 mM 3-MPA solution for 2, 4, and 8 h. Biosensor that immersed for 4 h showed highest biosensor responses (y = 0.8889x + 13.739; R2 = 0.9976) for pyruvate. When the electrode had been immersed for 2 h, responses of the biosensor decreased (y = 0.6368x + 13.178, R² = 0.982) as a result of not enough and suitable formations of SAM on the electrode surface related to duration time. When the electrode had been immersed for 8 h, linearity of calibration curve was deteriorated (y = 0.1193x + 5.9035, R² = 0.6885). Four hour is the best time for both enough and suitable formations of the molecules on the electrode surface and also the best enzymatic reaction. From the results of the experiments, it can be concluded that the most suitable biosensor responses and also calibration curves were obtained with 4-h duration of SAM.
In order to investigate the pH effect on biosensor responses two different buffer systems were prepared. For this purpose 50 mM phosphate buffers at different pH values (pH: 6.0, 6.5, 7.0, 7.5, and 8.0) and 50 mM concentration of citrate (pH 5.0–5.5) were prepared and used. According to the results, optimum pH value was found to be 6.0 for POX. Below and above this pH value decreases in the biosensor responses were observed. If we consider the optimum pH value (pH 5.0–7.0) of the free POX it can be said that the immobilization procedure did not much affect the optimum pH value of the enzyme and also biosensor responses.
Repeatability of biosensor
The repeatability of biosensor was examined by the detection of 30 μM pyruvate (n = 6). From the experiments, the average value (), standard deviation (S.D), and variation coefficient of the biosensor (C.V. %) were calculated to be 31.02 μM, ± 0.1914 μM, and 0.62%, respectively. It is obvious that pyruvate can be determined sensitively by using the biosensor.
Comparison of the biosensor with previously reported biosensor
A number of analytical characteristics of the biosensor were compared with previously reported biosensors. Comparisons of performances of the biosensors developed for pyruvate are shown in . As can be seen, 3-MPA/6-ACA/POX/GA-modified AuE shows better response time than previous reports and also good detection limits and linear ranges.
Table I. Comparison of performances of some biosensors developed for determination of pyruvate.
Conclusions
In this study, we described a new strategy for developing pyruvate biosensors by modified SAM method for determination of pyruvate. Immobilization method used in the construction of the biosensor is the first for POX. The biosensor developed has several attractive advantages such as fast response, good linearity, and a low detection limits. When we consider the determination limit of pyruvate and also response time of the biosensor, it could be said that the biosensor is much more sensitive than the other biosensors reported. On the other hand, the immobilization procedure of the enzyme on the electrode surface was very easy and useful. Response time of the biosensor was only 6 s for DPVs and 2 s for amperometric measurements. In the characterization studies in addition to repeatability operational and storage stability of the biosensor were also determined. After 15 assays, the biosensor sustained 90.5% of its initial performance and after 10 days, the biosensor lost 26% of its initial performance. As a result, the biosensor can be used for sensitive and accurate determination of pyruvate.
Declaration of interest
The authors report no declarations of interest. The authors alone are responsible for the content and writing of the paper.
References
- Akyilmaz E, Yorganci E. 2007. Construction of an amperometric pyruvate oxidase enzyme electrode for determination of pyruvate and phosphate. Electrochim Acta. 52:7972.
- Akyilmaz E, Yorganci E. 2008. A novel biosensor based on activation effect of thiamine on the activity of pyruvate oxidase. Biosens Bioelectron. 23:1874.
- Arai G, Noma T, Habu H, Yasumori I. 1999. Pyruvate sensor based on pyruvate oxidase immobilized in a poly(mercapto-p- benzoquinone) film. J Electroanal Chem. 464:143.
- Bergmann W, Rudolph R, Spohn U. 1999. A bienzyme modified carbon paste electrode for amperometric detection of pyruvate. Anal Chim Acta. 394:233.
- Gajovic N, Habermüller K, Warsinke A, Schuhmann W, Scheller FW. 1999. A pyruvate oxidase electrode based on an electrochemically deposited redox polymer. Electroanal. 11:1377.
- Gavalas VG, Chaniotakis NA. 2001. Phosphate biosensor based on polyelectrolyte-stabilized pyruvate oxidase. Anal Chim Acta. 427:271.
- Ghica ME, Brett CMA. 2006. Development of a novel glucose and pyruvate biosensors at poly(neutral red) modified carbon film electrodes. Application to natural samples. Electroanal. 18:748.
- Kubo I, Inagawa M, Sugawara T, Arikawa Y, Karube I. 1991. Phosphate_ sensor composed from immobilized pyruvate oxidase and an oxygen electrode. Anal Lett. 24:1711.
- Kwan RCH, Leung HF, Hon PYT, Cheung HCF, Hirota K, Renneberg R. 2005. Amperometric biosensor for determining human salivary phosphate. Anal Biochem. 343:263.
- Love JC, Estroff LA, Kriebel JK, Nuzzo RG, Whitesides. GM 2005. Self-assembled monolayers of thiolates on metals as a form of nanotechnology. Chem Rev. 105:1103.
- Mak WC, Chan C, Barford J, Renneberg R. 2003. Biosensor for rapid phosphate monitoring in a sequencing batch reactor (SBR) system. Biosens Bioelectron. 19:233.
- Mascini M, Mazzei F. 1987. Amperometric sensor for pyruvate with immobilized pyruvate oxidase. Anal Chim Acta. 192:9.
- Mendes RK, Carvalhal RF, Kubota LT. 2008. Effects of different self-assembled monolayers on enzyme immobilization procedures in peroxidase-based biosensor development. J Electroanal Chem. 612:164.
- Mizutani F, Tsuda K, Karube I, Suzuki S, Matsumoto K. 1980. Determination of glutamate pyruvate transaminase and pyruvate with an amperometric pyruvate oxidase sensor. Anal Chim Acta. 118:65.
- Mizutani F, Yabuki S, Sato Y, Sawaguchi T, Iijima S. 2000. Amperometric determination of pyruvate, phosphate and urea using enzyme electrodes based on pyruvate oxidase-containing poly(vinyl alcohol)/polyion complex-bilayer membrane. Electrochim Acta. 45:2945.
- Muller YA, Schulz GE. 1993. Crystal structures of two mutants of adenylate kinase from Escherichia coli that modify the Gly-loop. Science. 259:965.
- Muller YA, Schumacher G, Rudolph R, Schulz GE. 1994. The refined structures of a stabilized mutant and of wild-type pyruvate oxidase from Lactobacillus plantarum. J Mol Biol. 237:315.
- Nakamura H, Ikebukuro K, McNiven S, Karube I, Yamamoto H, Hayashi K, et al. 1997. A chemiluminescent FIA biosensor for phosphate ion monitoring using pyruvate ox dase. Biosens Bioelectron. 12:959.
- Rahman AM, Park DS, Chang SC, McNeil CJ, Shim YB. 2006. The biosensor based on the pyruvate oxidase modified conducting polymer for phosphate ions determinations. Biosens Bioelectron. 21:1116.
- Revzin AF, Sirkar K, Simonian A, Pishko MV. 2002. Glucose, lactate, and pyruvate biosensor arrays based on redox polymer/ oxidareductase nanocomposite thin films deposited on photolithographically patterned gold microelectrodes. Sens Actuators B Chem. 81:359.
- Situmorang M, Gooding JJ, Hibbert DB, Barnett D. 2002. The development of a pyruvate biosensor using electrodeposited polytyramine. Electroanal. 14:17.
- Zapata-Bacri AM, Burstein C. 1987–1988. Enzyme electrode composed of the pyruvate oxidase from Pediococcus species coupled to an oxygen electrode for measurements of pyruvate in biological media. Biosensors. 3:227.