Abstract
A series of 3D implants and filling materials prepared from powdered biodegradable polymers, polyhydroxyalkanoates (PHAs), have been designed for the purposes of reparative osteogenesis. The 3D implants are made of resorbable polymer of hydroxybutyric acid (poly-3-hydroxybutyrate, P3HB) and a composite of this polymer with hydroxyapatite (HA) (P3HB/HA). The properties of the implants were studied in vivo in a model of segmental osteotomy and compared with commercial material Bio-Oss®. All implants containing P3HB as the main component facilitate reconstructive osteogenesis. P3HB and P3HB/HA show pronounced osteoplastic properties; their in vivo degradation is slow and corresponds to the growth of a new bone tissue, facilitating normal reparative osteogenesis. Also, powdered P3HB and P3HB/tienam can be used as filling materials for osteoplasty of bone cavities infected by Staphylococcus aureus. Biodegradable 3D implants and P3HB-based filling materials show pronounced osteoplastic properties and degrade in vivo at a slow rate, enabling normal reparative osteogenesis.
Introduction
Bone defects tissue repair and facilitation of healing of bone tissue defects are challenges facing reconstructive medicine. Orthopedic traumas as well as dental and other socially significant bone diseases are among the most commonly occurring disorders. Based on their causation and pathogenesis, bone tissue defects can be divided into several groups: bone defects caused by cancer, inflammatory processes, atrophic processes in bone tissue, infectious complication, and traumas. Diseases and traumas of musculoskeletal system are among the most frequently occurring disorders that impair the quality of life, and their percentage in the total morbidity grows steadily (Alves and Rezende Citation2011, Bourne Citation1999). In spite of the use of modern constructs and treatments, unfavorable clinical outcomes still reach 37% (Rentsch et al. Citation2010, Sackett et al. Citation2000). Chronic osteomyelitis is a serious bone infection; it comprises 3–10% of surgical infectious bone diseases. Such methods as myoplasty, fasciocutaneous flap repair and filling of the cavity with synthetic materials do not enable complete anatomical and functional bone regeneration. Thus, one of the main challenges in the treatment of chronic osteomyelitis is finding a proper technique and a suitable material for bone defect repair (Greenfield and Bechtold Citation2008).
Novel biomaterials for bone tissue repair could be a solution. Healing of bone tissue defects is largely determined by the process of reparative osteogenesis. There are various materials to be used as transplants for bone tissue replacement, which differ in composition, form, and properties (Volva et al. Citation2008). None of them, however, fully conforms to the requirements of modern reconstructive surgery. Thus, new materials need to be found and the available ones should be improved. As currently understood, an ideal bone replacement material must possess the following properties: be osteogenic (i.e., contain cellular sources for osteogenesis); be osteoinductive (induce bone to grow); be osteoconductive (be able to serve as a scaffold on which the bone cells can attach and direct bone growth); be osteoprotective (have mechanical properties similar to those of bone) (Uemura et al. Citation2003, Shin and Mikos Citation2003, Hartman et al. Citation2005).
The most commonly used materials with a pronounced supporting function are calcium phosphate materials that have osteoinductive properties (Urist Citation1965, Urist et al. Citation1984, Damien and Parsons Citation1991, Daculsi Citation1999). The discovery of collagen's ability to induce bone tissue regeneration (Khilkin et al. Citation1976) gave an impetus to studies of biocomposite materials that contained both collagen and hydroxyapatite. Alveloform and Bigraft composites containing purified fibrillar skin collagen (Collagen Corp., Palo Alto, USA) were developed for maxillofacial and dental surgery. Hybrid materials based on calcium phosphates and collagen were produced also in Russia: Gidroxiapol, KollapAn®, Rosdent, Polistom, Indost, etc. The use of these materials to fill large bony defects is limited by their inferior physical-mechanical properties (tensile strength about 6.5 MPa and Young's modulus about 2 GPa). One of the most recent approaches to the improvement of mechanical properties of hydroxyapatite (HA)-based biomaterials (production of materials with lower rigidity and higher elasticity) has been the production of HA composites with synthetic polymers (polyethylene, polysulphone). These composites, however, are much less biocompatible than HA (Vacanti and Vacanti Citation2000). Another solution to this problem may be production of hybrid materials based on HA and biocompatible biodegradable polymers (Mistry et al. Citation2007, Link et al. Citation2008).
PHAs are attractive materials for use in surgical reconstruction of bone tissues. There are literature reports on preparation of mechanically strong PHA/HA composites, proving that incorporation of HA into a PHA enhances polymer strength (Doyle 1990, Knowles and Hastings Citation1993a, Citation1993b, Köse et al. Citation2003a, Citation2003b). Properties of P(3HB)/HA composites were studied by many authors (Luklinska and Bonfield Citation1997, Luklinska and Schluckwerder Citation2003, Coskun et al. Citation2005), who showed that addition of HA improved P(3HB) characteristics. Korkusuz et al. (Citation2001) reported effective therapy of experimental osteomyelitis in rabbits with antibiotic carrier rods constructed of PHAs.
The purpose of this study was to investigate osteoplastic properties of PHAs in experiments on laboratory animals using models of segmental osteotomy and chronic osteomyelitis of long fibular boons.
Materials and methods
Preparation of pure P3HB polymer
The materials used were poly-3-hydroxybutyrate (P3HB) samples synthesized by the bacterium Ralstonia eutropha B5786 (Mw 340 000 Da, crystallinity 76%). The strain is registered in the Russian Collection of Industrial Microorganisms. The culture technique was described elsewhere (Volova and Kalacheva Citation1996). P(3HB) (С4H6O2) is a homopolymer of D(-)-3-b-hydroxybutyric acid, an isotactic polyester with regular (С4H6O2) units. P3HB is extractable from bacterial biomass due to its ability to dissolve in organic solvents and to be then precipitated by alcohols. P3HB was extracted from bacterial biomass with chloroform and precipitated with ethanol. The procedure of re-dissolution and further precipitation of polymer was repeated several times to prepare specimens that would not contain any organic impurities of protein, carbohydrate or lipid nature. The trademark of the material is Bioplastotan (Trademark “BIOPLASTOTAN™” Citation2006).
Preparation of P3HB implants
Three types of implant materials were investigated in the experiment: 1) P3HB, 2) poly-3-hydroxybutyrate/hydroxyapatite hybrid material (P3HB/HA) containing 20% (w/w) HA (Polist Company ®, Moscow), and 3) bone substitute material Bio-Oss® (Geistlich, Switzerland) (used for reference purposes). PHAs were used as 3D implants and powder mixed with antibiotic tienam (Merck Sharp & Dohme, Switzerland).
To prepare a PHA/HA hybrid composite, a mechanical-physical method was used. The flocculent-fibrous polymer extracted from the bacterial biomass was crushed into particles with the following grain sizes: 0.50 mm (70.6% of the particles), 0.32 mm (23.4%), 0.20 mm (3.8%) and 0.14 mm (2.2%). The granulated polymer was mixed with HA and ground in an agate mortar in liquid nitrogen to uniform powder. Then, by cold molding (at a pressure of 120 kgf/cm2), we made 50 and 100 mg solid compact cylinders. As initial components (P3HB and HA) had different molecular weights and structures, the polymer and HA were crushed in an agate mortar in liquid nitrogen to uniform powder. The resulting mixture was weakly activated by grinding with ceramic balls in ceramic grinding jars of an EI-2x150 planetary ball mill for 30 s. The product of mechanical activation was homogenous grayish–white powder, which was processed by cold molding at a pressure of 120 kgf/cm2, using a Carver 3887/4387 Auto pellet press (USA), to produce pellets. The pellets were then placed into a drying oven (105°C) for 25 min, to allow the components to interact with each other. To prepare porous 3D matrices, sucrose was added to the P3HB/HA mixture prior to molding and was then leached out of the matrices by boiling them in water. Filling material was obtained by dividing the polymer particles into a series of narrow particle size fractions followed by determining the ratio of their mass. Sieve analysis was used to determine the particle size distribution, that is, material was scattered into fractions using a set of sieves with different size holes.
Characterization of the surface of implants
Water contact angles of the surface of implants were measured as follows. Distilled water was dropped on the surface of the samples (D = 10) with an automatic pipette. The drop size was 0.01 ml. A computer image of the drop was obtained with a digital camera, and the angular value was determined (the average angular value was calculated from the measurement of ten drops of each volume). The surface properties of the nanofibrous mats such as free surface energy (γS), free interface energy (γSL), and cohesive force (WSL) (erg/cm2) were calculated on the basis of the measured water contact angles (WCA) (θ, degrees), using the conventional equations (De Jennes Citation1987):
Free surface energy of the samples (erg/cm2) was calculated from the equation:
in which γL is the free surface tension of the water, 72.8 erg/cm2.
Free composite-water interface energy (γSL, erg/cm2) was calculated from the equation: γSL = γS + γL−WSL, in which γSL is the criterion of residual interphase energy and γS and γL are free surface energies of the sample and of the water, respectively.
Cohesive force, which characterizes the strength of the adhesive bond between the phases, was calculated from the formula:
The samples were packed using an NS 1000 shrink- wrapping machine (Hawo Gmbh, Germany) and sterilized with H2O2 plasma in the Sterrad NX system (Johnson & Johnson, US) for 45 min.
Animal model of segmental osteotomy
Experiments were conducted on 70 adult female Wistar rats (200–240 g each) in accordance with the international and Russian ethical rules for laboratory animal care. The rats were kept in an animal facility and fed a standard diet in accordance with the directive on maintaining animals and experimenting on them (Genin et al. Citation2001). The rats were divided into six groups (12 rats in each group): three treatments (with P3HB-based implants) and two controls (with implants prepared from commercial materials). Bone defects (1.5–3.0 mm in diameter and 1.0–3.5 mm in depth) were created in the sites that are known to provide the most representative data on bone formation in rats and enable correct evaluation of the effects of implants on the repair of the defect in the metaepiphysis of the tibia (Arseniev Citation2007, Barchenko et al. Citation2006, Leont'ev et al. Citation1995). The rats were given volatile inhalation anesthetic, condyles and the upper third of the right shin were uncovered, and a bone defect of 1.0–3.0 mm in diameter and 1.0–3.5 mm in depth was made with an osteotome of the 2.5 mm diameter at the metaepiphysis of the tibia, under continuous cooling using physiological saline. Then the defect was tightly filled with the implants. The postoperative wound was closed layer by layer. The initial area and the volume of P3HB-based implants were 23.56 mm2 and 29.45 mm3, respectively. Such parameters as the state of the animals, the support ability of the affected leg, and the state of the tissues at the site of implantation were monitored throughout the experiment. At days 14, 30, 60, and 90 post surgery, the rats were euthanized with a lethal dose of anesthesia, bone sites were explanted and investigated histologically.
Animal model of chronic osteomyelitis
The experiments were carried out using 4–5-month-old male chinchilla rabbits; 60 rabbits were used. The rabbits were divided into three groups (20 rabbits in each group): two treatment groups and one control group. The rabbits were anesthetized with ketamine–droperidol and a 3-cm long aperture was created through the anteromedial approach to the tibia. The muscles were bluntly separated and fixed. Osteotomy was performed through a 0.5-cm incision in the anterior surface of the metadiaphyseal region of the tibia; limited separation of periosteum was carried out; the medullary cavity was opened up and bone marrow was removed using Volkmann's curette. A gauze wick with Staphylococcus aureus culture (109 cells) was placed into the wound. The postoperative wound was closed layer by layer. Formation of the model of experimental osteomyelitis took 1 month; the model was 100% reproduced; primary chronic osteomyelitis was established.
Then, treatment of bone defects was performed in the rabbits with chronic osteomyelitis. Skin incision was made, and debridement of postoperative scars, fistula, and sinus tracts was performed. Purulent discharges were drained; necrotic tissues and sequestra were removed. During the surgery, samples were collected to identify the causative agent and determine antibiotic sensitivity. Trepanation of the bone was performed to reach the apparently healthy bone; medullary cavities were opened up. The resulting trough-shaped bone cavity and the adjacent medullary cavities were thoroughly curetted using a Volkmann's spoon. After all nonviable tissues were removed, the cavity was washed with an antiseptic solution (plivasept, chlorhexidine solution). Counterincisions were made and irrigators for postoperative drainage were placed at the surgery site. After that, the bone cavity was filled with bone substitute materials: demineralized autologous graft bone taken from the iliac crest (the control group) and experimental materials: P3HB and a P3HB/HA composite (the treatment groups). The wound was completely closed layer by layer. Suction drains were connected to a vacuum apparatus. During the postoperative period, the limbs were immobilized using plaster splints or orthoses. The state of the animals (appetite, locomotor activity, the state of the limbs that had been operated on) was monitored throughout the experiment. After termination at 30, 60, and 90 days postoperatively, bone specimens were retrieved and examined to estimate the state of the surrounding soft tissues, periosteum, cortical layer, and medullary cavity. Bacteriological plating on Chistovich medium (egg-yolk salt agar) was performed at the defect site. Results were evaluated at days 2–4.
Computer radiography
Radiography was performed using an X-ray machine (RUM-20) at 44 mA, 0.1 kV, exposure time 1 s. Radiography data obtained at days 15, 30, 60, 90, and 120 were analyzed to estimate the size and shape of the bone defect, the homogeneity of the structure of the regenerating tissue, and the state of periosteum, cortical layer, and medullary cavity.
Histological examination
Samples for histological examination were retrieved by carefully separating muscles from bones and isolating 1–1.5-cm-long bone segments with regenerating bone tissue. The condition of tissues was investigated using conventional histological techniques. Sections of tissues of internal organs were excised; the samples were fixed in 10% formalin, decalcified in a Trilon-V solution, dehydrated in a graded series of ethanol, and embedded in paraffin. Five to ten micrometers thick microtome sections were prepared. The tissues excised from one animal usually yielded 10–18 serial sections, which were stained with hematoxylin and eosin. Morphological examination was performed using an Axioskop 40 Pol. transmitted light-polarizing microscope (Carl Zeiss, Germany) equipped with an AxioCam MRc-5 digital camera. Investigation of the new bone tissue structure and estimation of the state of implanted materials and their resorption kinetics were based on image analysis and morphometric examination of sections using an Image Analysis System “Carl Zeiss” software package (Germany).
Statistical analysis
The results were analyzed statistically using the standard software package of Microsoft Excel. Arithmetic means and standard deviations were found. Significant differences between average values in control and treatment groups were tested using the Mann–Whitney U-test (significance level P < 0.05)
Results
Characterization of implants
shows photographs and SEM images of P3HB-based implants. The pore size ranged from 100 to 200 μm; P3HB/HA composite implants had a slightly higher porosity than P3HB ones (). SEM images show interconnected porosity, but the pore structure was somewhat irregular.
Figure 1. The upper row – a photograph of P3HB filling material (I), porous 3D implants: life-size (II), and × 10 magnified (III); the lower row – SEM images of P3HB porous implant.
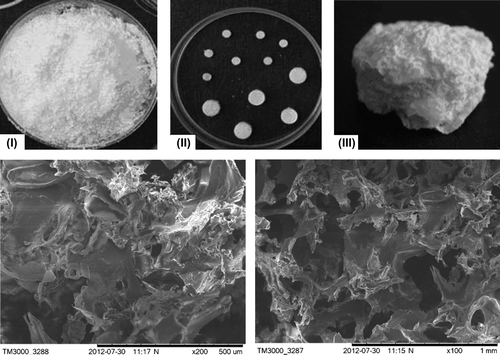
Table I. Characterization of P3HB-based 3D implants.
The implants had rather similar properties, with insignificant differences in the density and water contact angle. The densities of P3HB and P3HB/HA were 0.90 and 0.96 g/cm3, respectively. Characterization of the surface of the samples showed that free interface (composite/water) energy of the composite P3HB/HA drops to 7.5 erg/cm2, compared to 8.7 erg/cm2 recorded for P3HB, while the cohesive force, which characterizes the strength of the adhesive bond between the surface of the composite and the aqueous phase, and the wettability of the surface increase. This suggests the formation of a composite structure, different from the more hydrophobic samples of P3HB.
In vivo research
A study of P3HB in the animal model of segmental osteotomy.
All animals responded adequately to surgery; no significant complications occurred in the postoperative period. All wounds healed through primary intention. In 7–8 days after the surgery, the animals fully loaded the operated limb. In one animal of the control group, full weight-bearing capacity was observed 5 days later than in other animals. In the group with P3HB implants, one animal exhibited hypertrophic growth of the new bone tissue at the site of the model defect. In the other animals, the postoperative picture at the defect site was similar. At all time points of the observation period, all implants stayed at the site of bone defect. No tissue infection or inflammatory reaction was observed at the implantation site.
The effectiveness of osteogenesis employing P3HB implants was estimated using radiography (). X-ray examination did not show any lysis zones or formation of fibrous capsules around the implants. The images show that the employment of the P3HB and P3HB/HA implants resulted in more complete repair of the model defect of bone tissue than the employment of the commercial material Bio-Oss. At day 60, for most animals of the treatment group, radiography showed regeneration of the cortical plate and medullary cavity; the bone structure was entirely normal. At days 90–120, radiography showed regeneration of the anatomical structure of the bone in all rabbits. In the control group, at days 90–120, the regenerating bone tissue still had nonuniform structure, the periosteum was thickened, and the medullary cavity was undetectable.
Figure 2. Cross section samples of the bones at the defect site; implants: I – P3HB, II – P3HB/HA composite (HA 20 wt%), III – Bio-Oss® (arrows).
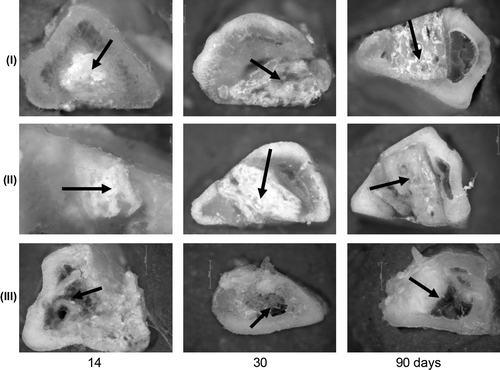
Healing of bone defects in all animals occurred in phases characteristic of reparative osteogenesis, including posttraumatic changes in tissue elements, regeneration, and adaptive remodeling. There were some differences in reparative osteogenesis, depending on which implant had been used to fill the model defect. shows that filling of the defect with the new bone tissue occurred with different rates, depending on the type of the implant used. In animals whose bone defects were filled with Bio-Oss®, osteogenesis was less active. Histological examination of the tissue sections at the site of the model defect also showed that implantation of PHA gave better results than implantation of reference materials.
Figure 3. Radiological data on the regeneration dynamics of the model defects of bone tissue in experiments with different implants.
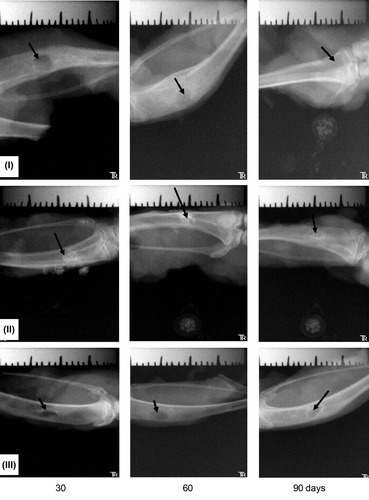
Histological tissue sections from the sites of model defects are shown in . In 30 days after bone defects were filled with P(3HB), bone lamellae were being rearranged into compact bone. That was confirmed by the presence of osteons with distinct cement lines. The P(3HB) implanted material was considerably reduced, with the area of polymer particles on histological sections decreased to about 60% of its initial size (). Growth of osteogenic tissue around the implants was observed in some sections. At the end of the experiment (90 days), new mature bone tissue was actively developing at the site of P(3HB) implantation; the tissue had lamellar structure; developing osteons were detected. The sections contained large particles of the degrading polymer, which were not incorporated into the osteogenic tissue and were grouped in clusters. The presence of the polymer at this time point suggests the low rate of its resorption. No inflammatory reaction or capsule formation was observed at the implant/native bone interface.
Figure 4. New tissue at the implantation site: I – P3HB, II – P3HB/HA, III – Bio-Oss – at different time points after surgery. Magnification: × 100 hematoxylin–eosin.
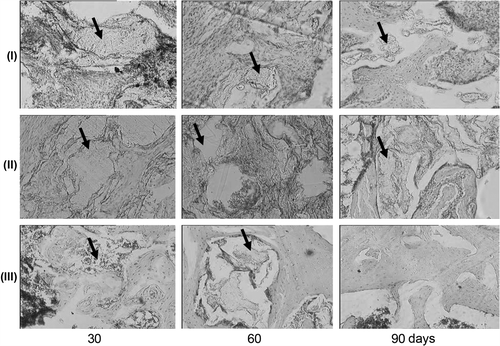
Reparative osteogenesis was somewhat different in the experiment with the P3HB/HA composite implanted to the bone defect. At day 30, the amount of osteogenic tissue was noticeably larger, but the position of bone lamellae was chaotic; the Haversian system was less pronounced than in the experiment with pure P(3HB), at the same time point (). The sections clearly exhibit particles of the composite material in osseous lacunas, with the red bone marrow and mesenchymal cells not integrated in the new bone tissue. Increased generation of hematopoietic cells was observed. The developing cortical bone contained large lacunas and was rather loose. Hence, its biomechanical properties must be much worse than those of the new bones in the animals with P(3HB) implants. The resorption of the composite P(3HB)/HA matrix occurred at a lower rate than the P(3HB) resorption. By the end of the experiment, at day 90, the cortical bone had been formed almost completely, with the Haversian system, osteocytes, and osteoclasts. In some regions of histological sections, the direction of bone lamellae of the newly formed tissue was rather chaotic than longitudinal. The sections still showed HA and P(3HB) particles. There were particles in which HA was separated from P(3HB) by a layer of mesenchymal cells.
In the experiment with Bio-Oss® implants, at day 30, the sections mainly showed compact bone with distinct Haversian systems. There were very few trabeculae and the proliferation activity of osteogenic cells and osteoblasts was very weak. The periosteum was thickened due to the fibrous layer. The intramedullary cavity was wide and filled with bone marrow. At day 90, similarly to day 30, the sections mainly presented compact bone with a wide intramedullary cavity filled with bone marrow (). There were no bone trabeculae. The endosteum was lined with osteogenic cells. The periosteum was fibrosed. In this experiment, morphological signs of bone repair were generally very weakly expressed. The amount of the new bone was lower than in the experiments with the implants based on P(3HB).
Results of in vivo experiments with pure poly(3-hydroxybutyrate) and P3HB loaded with HA, using the model of segmental osteotomy, showed that osteoplastic properties of P3HB were more pronounced than those of Bio-Oss; its in vivo degradation was slow and corresponded to the growth of new bone tissue, which gradually replaced the biomaterial, resulting in normal reparative osteogenesis.
A study of PHA in the animal model of chronic osteomyelitis
During the early postoperative period (up to 3 days), the rabbits remained sluggish, moved very little, did not load the affected limb. At days 3–5, the rabbits recovered their appetite and locomotor activity, but they did not load the affected limb. At days 5–12, 19 rabbits had soft tissue edema at the surgery site; 12 rabbits showed local dermal hyperemia. At day 16.5 ± 3.2, on average, the edema and hyperemia were reduced. The rabbits began to load the affected limbs at day 6.22 ± 1.72 post surgery. Surgical wounds healed at day 11 ± 1.32, on average. No animals died during the postoperative period. In the experiments with P3HB used as a filling material for the experimental osteomyelitis, we observed significantly more rapid healing of postoperative skin wounds and recovery of the support function of the affected limb than in the control group. In the treatment groups, healing of the surgical skin wound took 7.4 ± 0.9 days, on average, after surgery and reduction of the edema and hyperemia − 8.75 ± 0.96 days; while in the control group these processes took 9.9 ± 1.7 and 11.7 ± 1.8 days, respectively. Recovery of the support function of the affected limb was observed at days 4.28 ± 0.9 and 5.56 ± 1.2 in the treatment and control groups, respectively.
Radiography of the operated limbs revealed the following (): at day 30 after P3HB and P3HB/tienam were used to fill the bone defects, the projection image of the bone defect showed clear round areas with distinct boundaries and a cloud-like shadow in the center. At day 90, radiography showed regeneration of the anatomical structure of the bone in all rabbits. In the control group, at day 90, the regenerating bone tissue still had nonuniform structure, the periosteum was thickened, and the medullary cavity was undetectable.
Figure 5. Radiological data on the regeneration dynamics of the defects of bone tissue infected with Staphylococcus aureus in experiments with different implants: I – bone allograft; II – powdered P3HB; III – powdered P3HB/tienam.
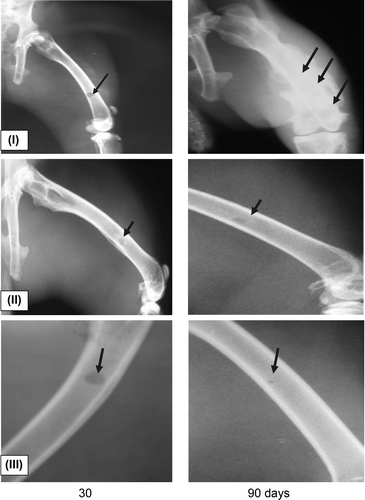
Results of microbiological investigation of the samples retrieved from the defect sites are given in . The microbial profile of the control group was investigated by plating microbial associations at days 30 and 90. These associations mainly contained S. aureus (48.2%) and associations of Gram-positive and Gram-negative anaerobic microorganisms and E. coli. In the P3HB treatment group, microbial associations were plated at day 30; they mainly contained S. aureus (44.1%) and associations of Gram-positive and Gram-negative anaerobic microorganisms and E. coli (55.9%). At day 90, bacterioscopic examination of the samples retrieved from this group gave a negative result, that is, the hydrophobic P3HB powder suppressed the infection. Bacterioscopic examination of samples of the material retrieved from the P3HB/tienam group did not show any growth of microorganisms at all time points.
Table II. Results of microbiological investigation of the samples.
Recovery of the anatomical structure of the bone tissue was only observed in the treatment groups. In the control group, the bone structure was not completely regenerated. Histological examination of tissue sections from the sites of model defects of the treatment groups (with P3HB and P3HB/tienam filled defects) showed that at day 15, the center of the defect was filled with fibrous connective tissue with pronounced perifocal proliferation of capillary-like vessels and focal lymphohistiocytic infiltration (). Primitive osteogenesis, with the formation of osteoid-like masses and bone trabeculae with architectural deformations and chaotic arrangement of osteocytes, was mainly observed around the new vessels. Osteogenesis regions showed a high degree of basophilia of cells and osseomucoid of the developing bone tissue. At this time point, in the control group the defect was filled by loose connective tissue and, partly, by granulation and fibro-reticular tissue and residual detritus. We observed pronounced leukocytic infiltration of the new tissue, hyperemia of blood vessels with the stasis of blood cells, and perivascular edema; vascular network was weakly developed.
Figure 6. New tissue at the site of implantation of I – powdered bone allograft, II – P3HB, and III – P3HB/tienam at different time points after surgery. Magnification: × 100 hematoxylin–eosin.
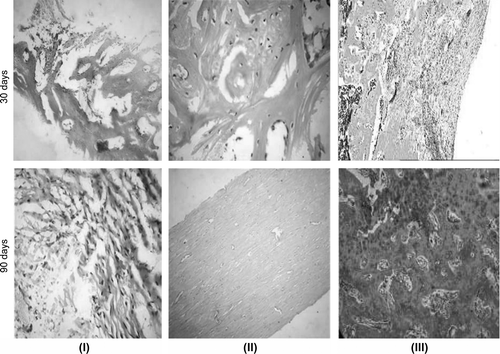
In the treatment groups, at day 30, the defect became smaller and was filled by fibrous, bone, and cartilage tissues (–III). Intercellular substance was arranged in concentric layers around the vessels of the new osteons; bone lamellae were being formed. Towards the periphery of the defect, the bone tissue was more mature and had an osteon-trabecular structure; the osteons were arranged irregularly; the Haversian canals had different widths and were hyperemic; no perivascular edema was observed. In the control group, the defect on the periphery was filled by immature bone tissue, with fibrous scar and cartilage tissue in the center. The new tissue and the surrounding bone tissue were weakly vascularized. The periosteum was thickened and infiltrated with macrophages. At day 90, all histological sections of the treatment groups showed signs of complete recovery of the bone structure. The periosteum was completely formed and consisted of the outer and inner layers. The bone tissue showed different degrees of maturity and had a laminar structure; osteons were arranged irregularly; the number of mature fibrocytes and osteocytes increased. Interstitial substance became optically denser and homogenous(). In the control group, at day 90, the defect site was seen as the narrowing of the bone at the surgery site. Microscopic examination of the sections showed that the defect was filled by rather mature bone tissue, with some interlayers of cartilage tissue(). Dense bone tissue with a few osteons prevailed; the intercellular substance was less homogenous and loose. The cells were mainly represented by mature fibroblasts and osteocytes; no basophilia of cells and intercellular substance was observed. The cortical plate was narrowed; the bone tissue was more mature; the cells were not numerous; sinusoid blood vessels were observed in intertrabecular spaces; the medullary cavity was either dramatically narrowed or covered with a layer of hyaline cartilage.
Thus, the experiments showed that using P3HB to fill the bone defect complicated by chronic osteomyelitis considerably facilitated the curbing of inflammation, bone defect repair, and recovery of the functional properties of the affected limbs and was significantly more effective than using bone allograft. Repair processes in experimental cavities of rabbits’ tibias filled with PHA resulted in anatomical and functional bone regeneration by day 60 post surgery. The filling of the bone cavity with demineralized autologous bone graft did not result in the regeneration of the bone structure.
Discussion
Diseases of musculoskeletal system are among the most frequently occurring disorders that impair the quality of life, and their percentage in the total morbidity grows steadily. New approaches to prevention of these disorders and treatment of vast bone defects resulting from serious traumas or diseases should involve the use of biologically active materials. Polymers of natural origin hold great promise for bone tissue regeneration. Collagen, polylactides, and polyglycolides are the most extensively studied materials intended for this application, but recently considerable attention has been given to polyhydroxyalkanoates (PHAs) (Shishatskaya et al. Citation2008). PHAs are attractive materials for use in surgical reconstruction of bone tissues as their bioresorption rates are low and biocompatibility is high. Another advantage of PHAs as materials for reconstructive osteogenesis is their piezoelectric effect (Knowles et al. Citation1992). Knowles and Hastings (Citation1993a, Citation1993b) studied the piezoelectric characteristics of PHA composites with glass fiber and found the piezoelectric potential output of these composites to be fairly close to that of bone. The authors noted a soft tissue reaction to subcutaneous composite implants and development of a new bone on the implant surface.
In this study, we used implants prepared from P3HB (the best studied PHA) and commercial material to repair experimental defects of bone tissue in laboratory animals and compared the results obtained. We also studied the effectiveness of using P3HB as a material for treatment of bone defects complicated by experimental chronic osteomyelitis.
We studied the effectiveness of using implants prepared from P3HB and a composite of P3HB and biological hydroxyapatite versus the bone substitute material Bio-Oss for repairing experimental defects of bone tissue in laboratory animals. Using the model of segmental osteotomy, we showed that filling of the defects with any P3HB-based 3D implants prevented ingrowth of loose connective tissue into the defect site and facilitated formation of mature bone tissue with distinctly laminar structure and developed osteons (Volva et al. Citation2009). The slowly degrading P3HB implants showed enhanced osseointegrative properties as compared to the bone substitute material Bio-Oss and, thus, can be used to repair bone tissue defects. Rentsch et al. (Citation2010) also reported the ability of P3HB to facilitate osteogenesis at the implantation site in a study of osteogenic potential of embroidered P3HB scaffolds in rats.
Studies reporting the use of PHA composites with calcium-phosphate materials are more numerous. The addition of hydroxyapatite improves the properties of P3HB, enhancing the strength of the material and making it similar to bone. Osteoblasts penetrate into the porous composite and proliferate there (Doyle et al. Citation1990, Luklinska and Bonfield Citation1997, Holland et al. Citation1990b).
In our study, P3HB/HA composite implants were resorbed at a slower rate than the implants prepared from pure PHA, and, hence, bone formation in the early phases of the experiment occurred somewhat slower. HA particles were slowly resporbed and were mainly found among bone marrow cells; small P3HB particles resulting from the degradation of larger ones were also found there. We recorded increased production of hematopoietic cells; the developing cortical bone, still having large lacunae, was rather loosely structured, which suggested that its biomechanical properties were inferior to those of the bones in the P3HB group. By the end of the experiment, at day 90, however, the cortical bone had been formed almost completely, with the Haversian system, osteocytes, and osteoclasts. Undegraded HA (40–45% of the initial amount) was still detected in the implantation site, and this percent was almost twice higher than the percentage of P3HB remaining at the implantation site in the P3HB group.
A study of biocompatibility, osteointegration, and osteoinduction of P3HB/HA composites (containing 10, 25, and 50 wt% HA) implanted in the model defect of bone tissue was reported by Carlo et al. (Carlo and Borges Citation2009). In the control group the defects were left unfilled. No significant differences were found between the groups in bone defect regeneration during the 90-day observation period. All variants of the composite were found to be biocompatible and capable of integration into bone. Alves et al. (Citation2010), however, evaluated the tissue response to a 70% P3HB and 30% hydroxyapatite composite in the form of a bone implant, placed intracortically in the distal metaphyseal of the right femur in cats, and showed that the composites triggered a chronic local inflammatory response, despite their clinical acceptance. In the study by Reis et al. (Citation2010), P3HB/HA (75/25%) composites were implanted in the defect made in the olecranon in rabbits for a time period of 90 days. Histological analysis showed new trabecular bone arising from the medullar area, between the composite and the cortical bone, connective tissue and new vascularized trabecular bone with no inflammatory infiltrate. These results proved biocompatibility of the composite. Promising results were obtained in a study of a composite of hydroxyapatite and P3HB/HV. Molecular weight of the composite implanted to laboratory animals gradually decreased for several months. As pores developed in the composite matrix, its biodegradation rate increased; new bone tissue development occurred within a normal period of time (Shishatskaya et al. Citation2005). Thus, most authors agree that PHA-based implants have pronounced osteoplastic properties, their in vivo degradation is slow and corresponds to the growth of a new bone tissue, which gradually replaces the biomaterial, resulting in normal reparative osteogenesis (Shishatskaya et al. Citation2006).
The second purpose of the study was to evaluate the effectiveness of using PHA to repair chronic osteomyelitis bone defects. The literature data on the effectiveness of using PHAs for this purpose are scant. Türesin et al. (Citation2001) reported a study of the release rate of antibiotics from model rods made of PHA and containing two antibiotics, Sulperazone® and Duocid®. Then, Korkusuz et al. (Citation2001) evaluated the use of such constructions in experiments on animals. Antibiotic carrier rods were constructed of different PHAs and contained antibiotics (1:1 w/w). The rods were implanted to rabbits infected with S. aureus, into the medullary area of rabbit tibia. The effectiveness of therapy was apparent at week 6 for all categories tested. The suppression of inflammation and tissue regeneration occurred simultaneously with the degradation of implants and release of antibiotic.
In this study, rabbits were experimentally infected with chronic osteomyelitis by inoculation of S. aureus into the bone cavity. After surgical debridement (draining of purulent discharges, removal of necrotic tissues and sequestra, washing of the wound with antiseptic solutions), bone cavities were filled with powdered P3HB and P3HB/tienam. In the control group, bone cavities were filled with bone substitute material: demineralized autologous bone graft taken from the iliac crest. The rabbits were observed for 90 days. Filling of infected bone defects of long bones with P3HB-based materials resulted in quicker suppression of infection, healing of postoperative wounds, and recovery of the support ability of the affected limb than in the control group of animals. Bactericidal properties of P3HB and P3HB/ bioactive glass composites were reported by Misra et al. (Citation2010). The authors studied the effect of porous scaffolds on the growth of S. aureus (NCTC 6571) and reported more effective suppression of Staphylococcus colonies on the composite scaffolds, probably due to the release of ionic products that result from the dissolution of bioglass particles and an increase in the pH of the medium. P3HB scaffolds, however, also suppressed the growth of Staphylococcus colonies.
In our study, in the postoperative period, the occurrences of soft tissue inflammations were significantly lower in the treatment groups than in the control; the incidence of S. aureus infection in the bone defect was also lower in the P3HB-treated animals. Histological analysis and radiography showed recovery of the anatomical structure of the bones in the treatment groups at day 90, while in the control group the medullary cavity was undetectable, the regenerating bone tissue still had nonuniform structure, and the periosteum was thickened.
Conclusion
Porous 3D implants prepared from P3HB and P3HB/HA composites have osteoinductive properties and effectively regenerate model defects of bone tissue. Powdered P3HB and P3HB/tienam can be used as filling materials for osteoplasty of bone cavities infected by S. aureus. Biodegradable 3D implants and P3HB-based filling materials show pronounced osteoplastic properties and degrade in vivo at a slow rate, enabling normal reparative osteogenesis. The results obtained in this study suggest that PHAs can be used as osteoplastic materials for regeneration of bone tissue defects.
Acknowledgments
This study was financially supported by Project “Biotechnologies of novel biomaterials” (Agreement No. 11.G34.31.0013) in accordance with Resolution No. 220 of the Government of the Russian Federation of April 9, 2010, “On measures designed to attract leading scientists to the Russian institutions of higher learning”
The project initiated by the Government of the Russian Federation (Decree No. 220 of 09.04.2010) for governmental support of scientific research conducted under the guidance of leading scientists at Russian institutions of higher learning (Agreement No. 11.G34.31.0013) and the Program of the President of Russia for young Doctors of Sciences (Grant № MD-3112.2012.4)
Declaration of interest
The authors report no conflicts of interest. The authors alone are responsible for the content and writing of the paper.
References
- Alves E, Rezende C. 2011. Orthopedic implant of a polyhydroxybutyrate (PHB) and hydroxyapatite composite in cats. J Feline Med Surg. 13:546–552.
- Alves E, Rezende C, Oliveira HP, Borges NF, Mantovani PF, Lara JS. 2010. Evaluation of the mechanics of polyhydroxybutyrate and hydroxyapatite composite plates in bone models of cat. J Arquivo Brasileiro de Medicina Veterinaria e Zootecnia 62:1367–1374.
- Arseniev IG. 2007. Experimental morphological study of clinical application bioimplantatov degraded in treatment of fractures and pseudarthrosis of long bones. The Dissertation for the degree of candidate of honey. Science. FSI CITO them NN Priorova, Moscow (in Russian).
- Barchenko GN, Kesyan GA, Urazgildyaev ZZ. 2006. Comparative experimentally-morphological study of the influence of some use in trauma and orthopedic practice calcium phosphate materials to enhance reparative osteogenesistion. J Bulletin of the East-Siberian Scientific Center, Siberian Branch of the Academy of Medical Sciences 4:327–332.
- Bourne RB. 1999. Fractures of the patella after total knee replacement. J Orthop Clin North Am 2:287–291.
- Carlo E, Borges A. 2009. Comparison of in vivo properties of hydroxyapatite-polyhydroxybutyrate composites assessed for bone substitution. J Craniofac Surg. 20:853–859.
- Coskun S, Korkusuz F, Hasirci V. 2005. Hydroxyapatite reinforced poly(3-hydroxybutyrate) and poly(3-hydroxybutyrate-co-3- hydroxyvalerate) based degradable composite bone plate. J Biom Sci Pol Ed. 16:1485–1502.
- Daculsi G. 1999. New technology for calcium phosphate bioactive ceramics in bone repair. J Med Biol Eng Com. 37:1598–1599.
- Damien CJ, Parsons JR. 1991. Bone graft and bone graft substitutes: a review of current technology and applications. J Appl Biomater 2:187–208.
- De Jennes PG. 1987. Adv Phys Sci. 151:619.
- Doyle C, Tanner ET, Bonfield W. 1990. In vitro and in vivo evaluation of polyhydroxybutyrate and polyhydroxyvalerate reinforced with hydroxyapatite. J Biomater. 11:206–215.
- Genin A, Iil`inand A, Kaplanskii A. 2001. Aviakosm Ekol Med. 35:14 (in Russian).
- Greenfield M, Bechtold J; Implant Wear Symposium 2007 Biologic Work Group. 2008. What other biologic and mechanical factors might contribute to osteolysis? J Am Acad Ortho Surg. 16:56–62.
- Hartman HM, Vehof JW, Spauwen PH, Jansen YA. 2005. Ectopic bone formation in rats: the importance of the carrier. J Biomater. 6:1829–1835.
- Holland SJ, Yasin M, Tighe BJ. 1990b. In vitro and in vivo evaluation of polyhydroxybutyrate and polyhydroxyvalerate reinforced with hydroxyapatite. J Biomed Eng 12:102–104.
- Khilkin AM, Shekhter AB, Istranov LP. 1976. Collagen and its applications in medicine. Medicina (in Russian) 210:98.
- Knowles JC, Hastings GW, Ohta H, Niwa S, Boeree N. 1992. Development of a degradable composite for orthopedic use: in vivo biomechanical and histological evaluation of two bioactive degradable composites based on the polyhydroxybutyrate polymer. J Biomater. 13:491–496.
- Knowles JC, Hastings GW. 1993a. In vitro and in vivo investigation of a range of phosphate glass-reinforced polyhydroxybutyrate based on degradable composites. J Material Sci. 4:102–106.
- Knowles JC, Hastings GW. 1993b. Physical properties of a degradable composite for orthopaedic use which attaches bone. Proceeding, First International Conference on Intelligent Materials. Lancaster: Technomic Publishing Co, 495–504.
- Korkusuz F, Korkusaz P, Eksiog lu F, Gürsel I, Hasirci V. 2001. In vivo response to controlled antibiotic release systems. J Biomed Mater Res. 55:217–228.
- Köse G, Kenar H, Hasirci N, Hasirci V. 2003a. Macroporous poly(3- hydroxybutyrate-co-3-hydroxyvalerate) matrices for bone tissue engineering. J Biomater. 24:1949–1958.
- Köse GT, Ber S, Korkusuz F, Hasirci V. 2003b. Poly(3-hydroxybutyric acid-co-3-hydroxyvaleric acid) based tissue engineering matrices. J Mater Sci. 14:121–126.
- Leont'ev VK, Volozhin AI, Kurdyumov SG. 1995. “Gidroksiapol” and “Kolapol” in dentistry. J News Dent. 5:32–35.
- Link DP, van den Dolder J, van den Beucken JJ, Cuijpers VM, Wolke JG, Mikos AG, Jansen JA. 2008. Evaluation of the biocompatibility of calcium phosphate cement/PLGA microparticle composites. J Biomed Mat Res. 87:760–769.
- Luklinska ZB, Bonfield W. 1997. Morphology and ultrastructure of the interlace between hy-droxyapatite-polyhydroxybutyrate composite implant and bone. J Mater Sci. 8:379–383.
- Luklinska ZB, Schluckwerder H. 2003. In vivo response to HA– polyhydroxybutyrate/polyhydroxyvalerate composite. J Elect Microscopy 211:121–129.
- Misra SK, Ansari TI, Valappil SP, Mohn D, Philip SE, Stark WJ, et al. 2010. Poly(3-hydroxybutyrate) multifunctional composite scaffolds for tissue engineering applications. J Biomater. 31:2806–2815.
- Mistry AS, Mikos AG, Jansen JA. 2007. Degradation and biocompatibility of a poly(propylene fumarate)–based/alumoxane nanocomposite for bone tissue engineering. J Bio Mat Res. 83: 940–953.
- Reis E, Borges A, Fonseca C, Martinez M, Eleotério R, Morato G, Oliveira P. 2010. Biocompatibility, osteointegration, osteoconduction, and biodegradation of a hydroxyapatite-polyhydroxybutyrate composite. J Braz Arch Biol Techn. 53:817–826.
- Rentsch C, Rentsch B, Breier A, Hofmann A, Manthey S, Scharnweber D, et al. 2010. Evaluation of the osteogenic potential and vascularization of 3D poly(3)hydroxybutyrate scaffolds implanted subcutaneously in nude rats. J Biomed Mater Res. 1, 185–195.
- Sackett K, Hendricks C, Pope R. 2000. Collaboration: an innovative education/business partnership. Case Manager. 6:40–48.
- Shin H, Mikos AG. 2003. Biomimetic materials for tissue engineering. J Biomater. 24:4353–4364.
- Shishatskaya EI, Markelova NM, Vinnik YS, Gavrilenko ES. 2008. Experimental formation of intestinal anastomosis using new absorbable suture material based on linear polyester of 3- hydroxybutyric acid. Health and Education in the 21st century; Proceedings of the IX International Congress, Russian.
- Shishatskaya EI, Volova TG, Gordeev SA, Puzyr AP. 2005. Degradation of P(3HB) and P(3HB-co-3HV) in biological media. J Biom Sci Pol Ed. 16:643–657.
- Shishatskaya EI. 2006. Biocompatible and functional properties of a poly(3-hydroxybutyrate/hydroxyapatite hybrid composite. Bull Transplant Artif Organs. 3:34–38 (in Russian).
- Trademark ‘BIOPLASTOTAN™’ 2006. Registration Certificate No. 315652 of the Federal Institute for Patent Examination for Application No 2006703271/50 (in Russian).
- Türesin F, Gürsel I, Hasirci V. 2001. Biodegradable polyhydroxyalkanoate implants for osteomyelitis therapy: in vitro antibiotic release. J Biom Sci Pol Ed. 12:195–207.
- Uemura T, Dong Y, Wang Y, Kojima H, Saito T, Iejima D, et al. 2003. Transplantation of cultured bone cells using combinations of scaffolds and culture techniques. J Biomater. 24:2277–2286.
- Urist MR, Leitze A, Davidson E. 1984. B–tricalcium phosphate delivery system for bone morphogenetic protein. J Clinical Ortopedic. 187:277–279.
- Urist MR. 1965. Bone: Formation by autoinduction. J Sci. 50:893–899.
- Vacanti CA, Vacanti JP. 2000. The science of tissue engineering. J Orthop Clin North Am. 31:351–356.
- Volova TG, Kalacheva GS. 1996. A Method for production of β-hydroxybutyric acid polymer. RF. Patent 2051967.
- Volova TG, Markelova NM, Vinnik YS, Cherdantsev DV, Gavrilenko ES, Kuznetsov MN, et al. 2008. Preliminary results of using biodegradable suture material based on a linear polyester of 3-hydroxybutyric acid. Adv Contemp Sci. 9:106–109 (in Russian).
- Volova TG, Shishatskaya EI, Mironov PV, Goreva AV. 2009. Structure and physicochemical properties composite polyxydroxybyturate/wollastonite. J Perspect Mater 1:43–50.