Abstract
Cholesterol-imprinted monosize poly(glycidyl methacrylate-N-methacryloyl-(L)-tyrosine methylester) microspheres were embedded into the poly(hydroxyethyl methacrylate) (PHEMA) cryogels and the resulting composite cryogel was used for the selective removal of cholesterol. Composite cryogels were characterized by swelling tests, multipoint BET apparatus, SEM, FTIR and elemental analysis studies. Specific surface area of the PHEMA cryogel was increased from 13 to 72.7 m2/g by embedding of microspheres. Composite cryogels removed 80% of cholesterol from homogenized milk. The maximum adsorption capacity was found as 42.7 mg/g for intestinal mimicking solution. After 20 adsorption–desorption cycles, there was no remarkable decrease in the adsorption capacity.
Introduction
Molecular imprinting is a widely used technique for producing polymers with the specific recognition sites for a target molecule. The polymerization occurs in the presence of the target molecule (the template, the imprint molecule). During the polymerization procedure the functional monomers and the cross-linking agents are copolymerized around the template at suitable conditions. After removal of the template from the obtained polymer network, the specific cavities are left inside the network. These specific cavities not only have size and shape memory for the template but also have chemical functionality complementary to the target molecule. By using this technique, highly selective affinity adsorbents can be produced through their molecular memory properties (Bossi et al. Citation2007, Cutivet et al. Citation2009, Bui and Haupt Citation2010).
Cryogels are sponge-like polymeric structures, which are obtained under the frozen conditions. Cryogels have macro-sized interconnected pores (macropores or supermacropores) and thanks to these large pores that have short diffusion path, low pressure drop and very short residence time (Demiryas et al. Citation2007, Babac et al. Citation2006, Andac et al. Citation2008). Unfortunately, cryogels have considerably low surface area due to their supermacroporous structure; the lower surface area leads to considerably low biomolecule adsorption capacity (Bereli et al. Citation2008). To improve the adsorption capacity of the cryogels, particle embedding is a useful method. We reported in our previous works that this approach combines all the advantages of the cryogels with better binding capacity (Yao et al. Citation2007, Le Noir et al. Citation2007, Baggiani et al. Citation2010).
Cholesterol is an essential sterol molecule for human body, which is required to build and maintain membranes; it has important roles in intracellular transport, protein activity regulations such as cell signaling and nerve conduction. Even though cholesterol is an important molecule for normal cell growth and function, high levels of cholesterol can be very dangerous for human body. In case a high level of cholesterol exists in the blood, cholesterol accumulates in coronary arteries as plaque that thickens the artery walls and causes coronary hearth diseases, such as atherosclerosis, peripheral vascular disease, stroke and death (Rozner and Garti Citation2006).
Studies showed that high consumption of cholesterol in daily diet could increase the plasma cholesterol level (Pyorala Citation1987). There are a lot of cholesterol source in our daily diet and many of the people wonder whether they intake excessive amounts of cholesterol or not. Milk is one of the essential parts of our daily diet, indeed, Christie reported that milk fat contains nearly 310 mg cholesterol/100 g milk fat and he also reported that most of the cholesterol in milk fat presents as free cholesterol form (Christie Citation1983). The cholesterol removal from milk is a highly expensive and time-consuming process. In literature, there are a lot of studies which were performed for reducing cholesterol level in milk samples but unfortunately their selectivity is very poor (Carleton et al. Citation1991, Gurr Citation1992, Sieber Citation1993). In this study, we proposed an alternative process for selective removal of cholesterol from cow milk and intestinal mimicking solution by using poly(hydroxyethyl methacrylate) (PHEMA)-based cryogel with embedded cholesterol-imprinted poly(glycidyl methacrylate-N-methacryloyl-(L)-tyrosine methylester) (PGMAT) monosize microsphere (mipPGMAT/PHEMA). Cholesterol adsorption studies, selectivity studies versus other competitive substances such as estradiol and stigmasterol have been reported here. Finally, repeated use of the mipPGMAT/PHEMA composite cryogel has also been studied.
Experimental
Materials
Homogenized milk sample was supplied from retail store (Ankara, Turkey). Cholesterol, stigmasterol, estradiol, L-tyrosinemethylester, methacryloyl chloride, poly(vinyl pyrrolidone) (PVP) (MW: 58,000) were supplied by Sigma (St Louis, USA). The monomers, glycidyl methacrylate (GMA), N,N’-methylene-bis(acrylamide) (MBAAm), 2-hydroxyethyl methacrylate (HEMA) and ethylene glycol dimethacrylate (EGDMA) were also obtained from Sigma (St Louis, USA), distilled under reduced pressure in the presence of hydroquinone inhibitor and stored at 4°C until use. α,α´-azobisisobutyronitrile (AIBN) and N,N,N’,N’-tetramethylene diamine (TEMED) were obtained from Fluka (Switzerland). Methanol and acetonitrile were HPLC grade and were supplied from Sigma (St Louis, USA). All other chemicals were of reagent grade and were purchased from Merck AG (Darmstadt, Germany). All water used in the experiments was purified using a Barnstead (Dubuque, IA) ROpure LP® reverse osmosis unit with a high flow cellulose acetate membrane (Barnstead D2731) followed by a Barnstead D3804 NANOpure® organic/colloid removal and ion exchange packed-bed system. The resulting purified water (deionized water) has a specific conductivity of 18 mS. Buffer and sample solutions were prefiltered through a 0.2 μm membrane (Sartorius, Göttingen, Germany). All glassware was extensively washed with dilute nitric acid before use.
Preparation of the cholesterol–MAT monomer complex
The MAT was chosen as the functional monomer to prepare monomer complex of cholesterol. The procedure of synthesis and characterization of MAT monomer were reported in our previous studies (Baydemir et al. Citation2007, Baydemir et al. Citation2009). The cholesterol–MAT complex was prepared with the molecular ratio of 1:2 (cholesterol: MAT monomer) so, cholesterol (0.5 mmol) was dissolved in 1 mL of methanol and MAT monomer (1 mmol) was added into this solution, stirred gently and the mixture was stored for 3 h to obtain a stable complex.
Preparation of cholesterol-imprinted monosize mipPGMAT microsphere
Briefly, 9.5 mL GMA, 0.2 mg AIBN, 0.2 mL EGDMA and 5 mL toluene was mixed and stirred for 5 min to obtain homogenous monomer phase and the cholesterol–MAT monomer complex was added into this solution. Dispersion medium was obtained as follows; 4 g of PVP was dissolved in 84 mL ethanol. Then the dispersion medium was added into the monomer phase in a glass reaction vessel (250 mL) and sonicated for about 5 min at 200 W with an ultrasonic water bath (Bransonic 2200, England). Before starting the dispersion polymerization mixture was purged with nitrogen gas for 30 min. Then the reaction vessel was immersed into the water bath at 70°C and the polymerization mixture was stirred by using anchor type stirrer at 760 rpm fixed stirring rate. The polymerization procedure was completed after 16 h and the reactor content was cooled down to room temperature. Molecular imprinted PGMAT microspheres (mipPGMAT) were separated from the dispersion medium by centrifugation at 7500 rpm for 15 min. MipPGMAT microspheres were washed with ethanol:water (30:70) mixture under the same conditions at least ten times to remove unreacted monomers and the other contaminants. Obtained mipPGMAT microspheres were finally washed with water several times and dried in an oven and stored at room temperature. Non-imprinted microspheres (nipPGMAT) were prepared by the same procedure without adding cholesterol into the polymerization mixture.
Preparation of PHEMA cryogel with embedded mip/nipPGMAT microspheres
Preparation of mipPGMAT microsphere-embedded PHEMA cryogel was described in our previous works in detail (Ergun et al. Citation2012). Briefly, monomers (1.6 ml HEMA and 275 mg N,N’-methylene-bis-acrylamide) (MBAAm) were dissolved in deionized water (10 mL) and mipPGMAT microspheres (300 mg) were swelled in deionized water (5 mL). Then microsphere solution was added into polymerization mixture. The cryogel was produced by free radical polymerization. After adding APS (15 mg) the solution was cooled in an ice bath for 2–3 min. TEMED was added and the reaction mixture was stirred for 1 min. Then the reaction mixture was poured in to a plastic syringe (4 mL) with closed outlet at the bottom. The polymerization solution in the syringe was frozen at the − 16°C for 24 h and then thawed at room temperature. After washing with 200 mL of water, the mipPGMAT/PHEMA composite cryogel was contacted with chloroform (15 mL) for the removal of template (cholesterol) molecules from the polymeric structure. For this purpose, chloroform was passed through the mipPGMAT/PHEMA composite cryogel at room temperature for 24 h. This procedure was repeated until no cholesterol leakage was observed from the polymeric structure to the wash solution. The cholesterol-free cryogel was cleaned with ethanol and water at room temperature for 12 h. NipPGMAT/PHEMA composite cryogels were prepared by embedding nonimprinted microspheres into the cryogel.
Characterization of the mip/nip PGMAT/PHEMA composite cryogels
The characterization of the composite cryogels was performed as similar as described in our previous works (Koc et al. Citation2011). Swelling ratio, gelation yield and % porosity were calculated according to the equations given below. All measurements were done in triplicate and the average values are presented.
where S is swelling ratio and W0, Ws are the weight of cryogel before and after uptake of water, respectively.
where mdried is the mass of dried sample and mt is the total mass of monomers.
where mswollen gel is the mass of swollen cryogel and msqueezed gel is the mass of cryogel after squeezing the free water.
The linear flow resistance of water passing through the column was measured at the constant hydrostatic pressure equal to 100 cm water column corresponding to a pressure of ca. 0.01 MPa. At least three measurements were done for each sample.
Surface area of the polymer sample was measured by the nitrogen sorption technique, performed on Flowsorb II, (Micromeritics Insrument Corporation, Norcross, USA). The specific surface area of composite cryogel in dry state was determined by multipoint Brunauer–Emmett–Teller (BET) apparatus (Quantachrome, Nova 2200E, USA). A sample weighing 0.5 g was placed on a sample holder and degassed in a N2-gas stream at 15°C for 1 h. Adsorption of the gas was performed at 21°C and desorption was performed at room temperature. Values obtained from desorption step was used for the specific surface area calculation.
The morphology of a cross section of the cryogel was investigated by scanning electron microscope (SEM). Previously, mipPGMAT microspheres were dried at 40°C in a vacuum oven for 5 days. PHEMA cryogel and mip/nip PGMAT/PHEMA composite cryogel were freeze dried in order to protect pore structures. After the polymer samples were dried, tiny fragments of the polymers were mounted on SEM sample holders on which they were sputter coated for 2 min. The samples were then consecutively mounted in a scanning electron microscope (Model: Jeol JEM 1200 EX, Tokyo, Japan) to visualize the surface structures of each polymers at desired magnification levels.
Fourier Transform Infrared (FTIR) spectra of MAT, cholesterol and the MAT–cholesterol complex were obtained using a FTIR spectrophotometer (FTIR 8000 Series, Shimadzu, Japan). Cholesterol, MAT monomer and MAT–cholesterol complex were dried in a vacuum oven. The dry sample (0.1 g) was thoroughly mixed with KBr (0.1 g, IR Grade, Merck, Germany), pressed into a pellet form and the FTIR spectrum was then recorded.
To evaluate the amount of MAT into the microsphere structure, it was subjected to elemental analysis using a Leco Elemental Analyzer (Model CHNS-932, USA).
Assay of steroids
The detection of cholesterol, estradiol and stigmasterol was followed by using high performance liquid chromatography (HPLC) system (Ultimate-3000, Dionex, USA) equipped with LPG-3000 pump, WPS-3000 autosampler, TCC-3000 column department, PDA-3000 detector and column (Kromasil 100–5, Length/I.D; 150/4.6 mm). Mobile phase was selected as a mixture of acetonitrile–methanol and water. Mobile phases were denoted as A (water), B (methanol) and C (acetonitrile). A linear gradient with the 0.5 mL/min flow rate was performed for the chromatographic separation. A linear gradient started from 10% B, 80% C and 10% A in 5 min, continued with increasing B from 10% to 65% and decreasing C from 80% to 35% in 1 min and finished in 20 min 100 mL of steroid solution was injected into the column. The absorbance was monitored at 280 nm for, cholesterol, estradiol and stigmasterol. The separation was performed at ambient temperature.
The limit of quantification (LOQ) and the limit of detection (LOD) values were calculated by using the following equations:
where s is the standard deviation of response and m is the slope of the corresponding calibration curve.
Cholesterol adsorption studies
Cholesterol removal from intestinal mimicking solution
Preparation of intestinal mimicking solution (A). Firstly 0.2 M potassium dihydrogen phosphate solution (125 mL) and 0.2 M sodium hydroxide solution (95 mL) was mixed. Then 200 mL water was added into this mixture. Then 24.5 g of sodium deoxycholate (NaDC) and 16.5 g sodium cholate (NaC) was added to final mixture. After this step, the pH was adjusted to 7.5 by using NaOH (0.2 M) and the volume of mixture was completed to 500 mL with water. The final solution was purged with nitrogen for 15 min. The obtained intestinal mimicking solution (IMS) was stored in dark at room temperature (Sellergren et al. Citation1998).
Preparation of cholesterol standard solution (B). 900 mg of cholesterol was added into 500 mL of (A) solution and this mixture was sonicated at 50°C for at least 3 h. The final solution was sparged with nitrogen for 15 min and stored in dark at room temperature (Daneshfar et al. Citation2009).
Adsorption studies of cholesterol in IMS. The cholesterol adsorption on to the mipPGMAT/PHEMA composite cryogel was studied in continuous system. The composite cryogel column was washed with 30 mL of water and then equilibrated with 30 mL of IMS A. The adsorption studies were performed as follows: 30 mL of the IMS B was pumped through the cryogel column at room temperature for 2 h by using a peristaltic pump (mass of dry cryogel column = 0.35 g). Cholesterol concentration was measured using HPLC (Dionex, Ultimate HPLC, ABD). Dynamic binding capacity was calculated from the cholesterol breakthrough curves. The experiments were performed in replicates of three and the sample was analyzed in replicates of three as well. The amount of cholesterol adsorption per unit mass of the cryogel was evaluated by using the mass balance.
Cholesterol removal from milk
Milk sample preparation. Before and after treatment of milk with mip/nip PGMAT/PHEMA composite cryogel column, 1 ml sample was taken to determine the amount of cholesterol in milk by Liebermann–Burchard colorimetric method at 620 nm (Xiong et al. Citation2007). Before the determination of cholesterol by colorimetric method, cholesterol was extracted from milk sample. The cholesterol extraction is explained briefly, 0.8 g milk sample was mixed with 8 mL chloroform:methanol 2:1 (v/v) solution. After mixing milk sample with chloroform and methanol by vortex, the sample was centrifuged at 4000 rpm for 15 min and then the supernatant was separated to another tube. One fifth times as much as supernatant volume, 0.02% CaCl2 was added to the sample and mixed by vortex. After that, the solution gave two phases. The upper phase was removed gently and the bottom phase was mixed with the chloroform:methanol:water (2:50:50, v/v) solution. After the phase separation, the upper phase was removed and the bottom phase was used for determination of cholesterol. Additionally, 10, 5 and 2.5 mM cholesterol standard solutions were prepared in chloroform:methanol 1:1 (v/v) solution.
Selectivity experiments
The specificity of mipPGMAT/PHEMA composite cryogel for cholesterol was estimated by using stigmasterol and estradiol as competitor agents since their molecular structures are quite similar with cholesterol. The selectivity studies were performed in water/THF mixture (5:6, v/v) since the steroids estradiol and stigmasterol were insoluble in IMS. The solution (10 mL) was overloaded with estradiol, cholesterol and stigmasterol and applied on mipPGMAT/PHEMA composite cryogel column. After attaining adsorption equilibrium, the concentrations of estradiol and stigmasterol in the remaining solution were measured by HPLC (Daneshfar et al. Citation2009).
The distribution coefficient (Kd) for estradiol and stigmasterol with respect to cholesterol was calculated by Eq. (6).
Where Kd represents the distribution coefficient (mg/mL); Ci and Cf are initial and final concentrations of cholesterol (mg/mL), respectively. V is the volume of IMS (mL) and m is the weight of the cryogel column (g).
The selectivity coefficient (k) for the binding of cholesterol in the presence of the other competitive molecules can be obtained from binding data according to,
where k is the selectivity coefficient and X represent stigmasterol or estradiol. A comparison of the k values of the mipPGMAT/PHEMA composite cryogel with that stigmasterol and estradiol allows an estimation of the effect of imprinting on selectivity. A relative selectivity coefficient, k′, can be defined as;
Desorption and repeated use
Desorption of cholesterol molecules was performed with chloroform and acetonitrile mixture (2:1, v:v). Cholesterol-imprinted mipPGMAT/PHEMA composite cryogel was contacted with elution medium for 2 h at room temperature. Final cholesterol concentration in desorption medium was measured by HPLC. Desorption ratio was calculated from the amount of cholesterol adsorbed on the composite cryogel and the final cholesterol concentration in the desorption medium.
In order to test the reusability of the mipPGMAT/PHEMA composite cryogel, cholesterol adsorption–elution cycle was repeated 20 times by using the same cryogel. In order to regenerate and sterilize the composite cryogel column after elution, it was washed with 50 mM NaOH.
Results and discussion
Characterization studies
(N)-methacryloyl-(L)-tyrosine (MAT) was selected as a functional comonomer for the selective separation of cholesterol and synthesized from the reaction of (L)-tyrosine methylester and methacryloyl chloride (). The 1H NMR spectrum of MAT was given in our previous studies, briefly; 2.05 ppm 3H singlet (-C = C-CH3, vinyl metyl), 2.5 ppm 1H, 3.5 ppm 2H (-C = CH2), 3.65 ppm 2H (-CH2-CH-), 5.4 ppm 1H (-OH), 5.65 ppm 1H (amine protons), 6.6–7.1 ppm aromatic protons. Carboxyl proton was observed between 9.0 and 10.0 ppm as a large peak (Yavuz et al. Citation2007)
FTIR spectrum of MAT has the characteristic stretching vibration amide I and amide II adsorption bands at 1657 cm− 1 and 1517 cm− 1, and carbonyl band at 1733 cm− 1 as shown in . FTIR spectrum of pure cholesterol includes H-bonded alcohol at 3417 cm− 1 and strong hydrocarbon peaks around 2900 cm− 1. Stretching vibration bands are at 1670 and 952 cm− 1. Functional monomer MAT is expected to interact with cholesterol through H-bonds and hydrophobic interactions through aromatic ring. Cholesterol-imprinted particles’ FTIR spectrum exhibits shifts in wave numbers of related peaks (). The observed shift may originate from the formation of complex between cholesterol molecules and MAT monomer. It should be noted that peak intensities were decreased in the resulting polymer.
The mip/nipPGMAT microspheres were synthesized by suspension polymerization. They were sieved and the fractions that fall in the size range of 2–3 μm were used throughout the study. MAT incorporation amount was determined by elemental analysis. The amount of nitrogen determined comes only from incorporated MAT groups into mipPGMAT microspheres. The incorporation of MAT into the mipPGMAT was found to be 460.5 μmol/g polymer using nitrogen stoichiometry.
represents the swelling properties and linear flow resistance (at hydrostatic pressure, ca. 0.01 mPa) of both mip/nipPGMAT/PHEMA composite cryogels. Cryogels have supermacropores, thanks to this; the linear flow resistance through the gel matrix is high. MipPGMAT/PHEMA showed the maximum linear flow resistance at 612 cmh−1 and nipPGMAT/PHEMA showed a linear flow resistance at 624 cmh−1. Both mipPGMAT/PHEMA, nipPGMAT/PHEMA composite cryogels were produced with high gelation yield (about 90%) and had similar swelling properties with a swelling degree of about 7.2 g H2O/g polymer.
Table I. Swelling properties of the mip/nipPGMAT/PHEMA composite cryogels.
The specific surface area of the PHEMA cryogel, nipPGMAT/PHEMA and mipPGMAT/PHEMA composite system was found as 13, 67.1 and 72.7 m2/g by multipoint BET method, respectively. As understood, embedding of the PGMAT microspheres increased the surface area of the polymers considerably. In addition the cavities of template cholesterol molecules of microspheres resulted in polymers having a larger surface area for the mipPGMAT/PHEMA.
The scanning electron microscopy showed the surface morphologies of the mipPGMAT microspheres, mipPGMAT/PHEMA composite cryogels and non-embedded PHEMA cryogels. As seen in , the radius of embedded microspheres is about 2 μm. The macroporosity of the cryogels and also the embedded particles in this structure provides large surface area to cryogels.
Cholesterol removal studies from IMS
Removal of the template
The mipPGMAT/PHEMA composite cryogel was treated with 15 mL of chloroform solution at room temperature for 48 h in order to remove the template from the selective cavities of mipPGMAT microspheres. Composite cryogel was periodically squeezed gently during this procedure. shows the decrease in the amount of template cholesterol released from the mipPGMAT/PHEMA composite cryogel. The peaks were labeled as 1–3 in order to show the amount of cholesterol released from the mipPGMAT/PHEMA cavities. This procedure was performed until no significant cholesterol peak was observed, which was the proof that almost all of the template cholesterol was removed from mipPGMAT/PHEMA composite cryogel.
HPLC was used for the detection of the amount of cholesterol. The calibration curve was created by plotting the absorbance data versus standard concentration of cholesterol (). The LOD value was found as 0.768 mg/mL for cholesterol.
Table II. Statistical evaluation of the calibration data of cholesterol.
Effect of flow rate
The amounts of adsorbed cholesterol at different flow-rates are given in . Results show that the cholesterol adsorption capacity on the mipPGMAT/PHEMA composite cryogel decreased when the flow-rate through the column is increased. The amount of adsorbed cholesterol decreased significantly from 10.7 to 3.0 mg/g polymer with the increase in the flow rate from 0.5 to 4.0 mL/min. This is due to decrease in contact time between the cholesterol molecules and composite cryogel at higher flow-rates. When the flow-rate decreases, the contact time in the column is longer. Thus cholesterol molecules have more time to recognize the cholesterol cavities of embedded MIP microspheres in the PHEMA cryogel structure and to move into the molecular cavities; hence a higher adsorption amount is obtained.
Effect of equilibrium cholesterol concentration
shows the equilibrium concentration dependence on adsorbed amount of cholesterol onto the mipPGMAT/PHEMA composite cryogel. The amount of adsorbed cholesterol is increased with increased cholesterol concentration, which represents saturation of the accessible binding cavities on the mipPGMAT/PHEMA composite cryogel. Maximum adsorption capacity was found as 42.7 mg/g polymer at initial cholesterol concentration of 2.0 mg/mL. The results show that the mipPGMAT/PHEMA composite cryogel have a higher binding capacity for cholesterol than that of the control PHEMA cryogel (non-embedded with MIP microbeads). MipPGMAT/PHEMA composite cryogel showed three times higher capacity to adsorb the cholesterol from IMS than PHEMA cryogel without embedding MIP microbeads suggesting the potential use of this cryogel embedded with MIP microbeads for reducing cholesterol from daily diet products including milk.
Adsorption isotherm
Langmuir and Freundlich isotherms were used for the modeling of the equilibrium adsorption data (Finette et al. Citation1997).
The Langmuir isotherm was expressed by the following equation,
In Eq. (9), Langmuir monolayer adsorption capacity was denoted as q (mg/g), Ce is the equilibrium cholesterol concentration (mg/mL), and b is the Langmuir adsorption equilibrium constant.
Langmuir adsorption model indicates the adsorption mechanism as follows: the adsorbent's surface is uniform, and the molecules adsorbed on the equivalent adsorption site of that uniform surface. So, the adsorbate molecules formed a monolayer structure on this surface and according to that model the adsorbate molecules don't interact with each other.
The second isotherm called Freundlich isotherm is expressed as follows,
In Eq. (10), Kf is the Freundlich adsorption constant (mg/mL), Ceq is the equilibrium cholesterol concentration (mg/mL), and n is the Freundlich exponent. The Freundlich isotherm describes adsorption behavior on heterogeneous system, when the Freundlich exponent n approaches zero it means that the system will be more heterogeneous and if n approaches unity then the system will be more homogenous (Umpleby et al. Citation2001).
The Langmuir and Freundlich adsorption constants with the correlation coefficients are given in . The correlation coefficient of the Langmuir isotherm is higher than that of Freundlich and the Freundlich constant n approaches unity, so it can be concluded that the adsorption of cholesterol onto mipPGMAT/PHEMA cryogel is a monolayer adsorption.
Table III. The Langmuir and Freundlich adsorption isotherm constants.
Adsorption mechanism clarified by using pseudo first and second order kinetic models. These models assume that the concentrations of the adsorbate molecules are equal to adsorbent surface concentration. The adsorption of solute from a liquid solution is expressed by using Lagergren (Cheung et al. Citation2001) first order rate expression,
where qe is the experimental amount of cholesterol adsorbed at equilibrium (mg/g); qt is the amount of cholesterol adsorbed at time t (mg/g); k1 is the rate constant of the pseudo first order adsorption (min− 1). A linear dependence of log (qe−qt) on t suggests the applicability of this kinetic model. Also, in many cases, the pseudo first-order equation of Lagergren does not fit well to the whole range of contact time and is generally applicable over the initial stage of the adsorption processes. The pseudo-second order kinetic model is expressed by Eq. (12):
where k2 is the rate constant of the pseudo second-order adsorption (gmL− 1min− 1). If the pseudo-second order kinetics is applicable, the plot of t/q versus t should be linear. The pseudo-second order kinetic model is favorable, when the adsorption behavior over the whole range of adsorption is in agreement with chemical adsorption being the rate-controlling step (Liang et al. Citation2005).
A comparison of the experimental adsorption capacity and the theoretical values is presented in . The correlation coefficient for the linear plot of log (qeq−qt) versus t for the pseudo-first order equation is lower than the correlation coefficient for the pseudo-second order equation suggesting that the pseudo-second order adsorption mechanism is predominant and that the overall rate of the cholesterol adsorption process appeared to be controlled by binding kinetics rather than by mass transport.
Table IV. The pseudo first/second order kinetic constants for mipPGMAT/PHEMA composite cryogel.
Selectivity experiments
Adsorption capacity of the mipPGMAT/PHEMA composite cryogel for adsorption of cholesterol as well as related molecules stigmasterol and estradiol was evaluated (). The adsorption capacity of corresponding NIP particles towards MIP adsorbents were shown in . The results indicate that MIP microbeads have higher adsorption capacities for cholesterol than that for either stigmasterol or estradiol. The changes of the kd values provide the comparison of mipPGMAT/PHEMA composite cryogel with the control PHEMA cryogel. The results showed an increase in kd for cholesterol while kd decrease for stigmasterol and estradiol. The relative selectivity coefficient gives valuable information about binding affinity of the recognition sites of the mipPGMAT/PHEMA composite cryogel and the target cholesterol molecules. The result shows that relative selectivity coefficients of mipPGMAT/PHEMA composite cryogel for cholesterol/estradiol and cholesterol/stigmasterol were 181.6 and 169.93 (k′) greater than the nipPGMAT/PHEMA matrix, respectively. Estradiol and stigmasterol were less adsorbed by the mipPGMAT/PHEMA composite cryogel because of their fewer chances to interact compared with cholesterol. Nevertheless, the binding specificity for cholesterol from mipPGMAT/PHEMA composite cryogel was sufficient for the recognition of cholesterol from other compounds. summarizes the kd, k and k′ values in selectivity studies.
Figure 9. Adsorption of cholesterol in the presence of competitor estradiol and stigmasterol molecules on mip/nipPGMAT/PHEMA composite cryogels.
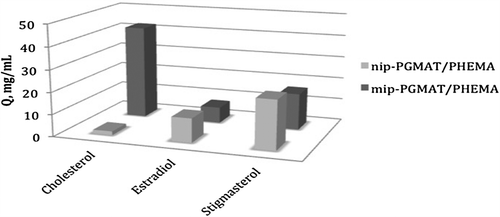
Table V. k, kd and k’ values of estradiol and stigmasterol with respect to cholesterol.
Cholesterol removal from cow milk
Cholesterol removal studies were performed from commercial homogenized milk (3.4% milk fat), which was purchased from a retail store. The cholesterol amount in the sample was determined as 12.3 mg cholesterol/100 g milk sample by using colorimetric method and this value is complying with the cholesterol amount in milk samples given in literature (Han et al. Citation2005). The removal ratio of cholesterol was found as 80% (). The results showed that the cholesterol is removed from the homogenized cow milk successfully by using mipPGMAT/PHEMA cryogel. There are a lot of studies performed for the removal of cholesterol from dairy products such as butter oil (Dias et al. Citation2010), cheese (Han et al. Citation2008), milk (Han et al. Citation2005, Noseda et al. Citation2007) and egg yolk (Garcia Rojas et al. Citation2007) in literature, suggesting many kinds of methods using physical, chemical and biological approaches for reducing the cholesterol level. All these methods reduced cholesterol level in dairy products but the main problem of these methods is lack of selectivity. Han E. et al. removed cholesterol from homogenized milk by using crosslinked B-cyclodextrin by adipic acid and they found the removal percent as 90% (Han et al. Citation2005); in our study cholesterol removal ratio was found as 80%. When compared to that mentioned in the literature, mipPGMAT/PHEMA composite cryogels removed a high extent of cholesterol with a significantly high selectivity.
Table VI. Cholesterol removal from homogenized cow milk.
Elution and repeat use
In order to show the stability and reusability of mipPGMAT/PHEMA composite cryogel an adsorption–elution cycle was repeated 20 times by using the same mipPGMAT/PHEMA composite cryogel in a continuous experimental set up. For sterilization, the cryogel was washed with 50 mM NaOH solution for 30 min after one adsorption–elution cycle. At the end of 20 adsorption–elution cycles there was no remarkable decrease in the cholesterol adsorption capacity (). As seen here, the mipPGMAT/PHEMA composite cryogel is very stable and maintain their adsorption capacity at almost constant value of 94%.
Conclusion
The results presented here demonstrate that the PHEMA cryogels embedded with mipPGMAT microspheres can be used for the recognition and selective removal of cholesterol molecules from IMS and milk. This recognition may be through a multistep binding with the specificity conferred by hydrophobic interactions and shape selectivity. Because the mipPGMAT microspheres are located in, or close to, the macropore surface these mipPGMAT microspheres have good side accessibility toward the target cholesterol molecules in IMS and milk. The mipPGMAT microspheres increased the surface area of the PHEMA cryogel (about 5 fold). Furthermore the large surface area of the microbeads results in higher cholesterol binding capacity of the mipPGMAT/PHEMA composite cryogel.
Declaration of interest
The authors report no declarations of interest. The authors alone are responsible for the content and writing of the paper.
References
- Andac M, Plieva FM, Denizli A, Galaev IY, Mattiasson B. 2008. Poly(hydroxyethyl methacrylate)-based macroporous hydrogels with disulfide cross-linker. Macromol Chem Phys. 209:577–584.
- Babac C, Yavuz H, Galaev IY, Pişkin E, Denizli A. 2006. Binding of antibodies to concanavalin A modified monolithic cryogel. React Funct Polym. 66:1263–1271.
- Baggiani C, Baravalle P, Giovannoli C, Anfossi L, Giraudi G. 2010. Molecularly imprinted poluymer/cryogel composites for solid-phase extraction of bisphenol A from river water and wine. Anal Bioanal Chem. 397:815–822.
- Baydemir G, Andac M, Bereli N, Say R, Denizli A. 2007. Selective removal of bilirubin from human plasma with bilirubin-imprinted particles. Ind Eng Chem Res. 46:2843–2852.
- Baydemir G, Bereli N, Andaç M, Say R, Galaev IY, Denizli A. 2009. Supermacroporous poly(hydroxyethyl methacrylate) based cryogel with embedded bilirubin imprinted particles. React Funct Polym. 69:36–42.
- Bereli N, Andac M, Baydemir G, Say R, Galaev IY, Denizli A. 2008. Protein recognition via ion-coordinated molecularly imprinted supermacroporous cryogels. J Chromatogr A. 1190:18–26.
- Bossi A, Bonini F, Turner AP, Piletsky SA. 2007. Molecularly imprinted polymers for the recognition of proteins: the state of the art. Biosens Bioelectron22:1131–1137.
- Bui BT, Haupt K. 2010. Molecularly imprinted polymers: Synthetic receptors in bioanalysis. Anal Bioanal Chem. 398:2481–2492.
- Carleton RA, Finberg L, Flora J, Goodman DS, Grundy SM, Haven S, et al. 1991. Report of the expert panel on population strategies for blood cholesterol reduction. Circulation. 83:2154–2232.
- Cheung CW, Porter JF, Mckay G. 2001. Sorption kinetic analysis for the removal of cadmium ions from effluents using bone char. Water Res. 35:605–612.
- Christie WW. 1983. The consumption and structure of milk lipids. P.5 in Developments in Dairy Chemistry, Vol. II. Applied Science. New York, N.Y.: Academic Press.
- Cutivet A, Schembri C, Kovensky J, Haupt K. 2009. Molecularly imprinted microgels as enzyme inhibitors. J Am Chem Soc. 131:14699–14701.
- Daneshfar A, Khezeli T, Lotfi HJ. 2009. Determination of cholesterol in food samples using dispersive liquid-liquid microextraction followed by HPLC-UV. J Chromatogr B. 877:456–460.
- Demiryas N, Tuzmen N, Galaev IY, Pişkin E, Denizli A. 2007. Poly(acrylamide-allyl glycidyl ether) cryogel as a stationary phase in dye affinity chromatography. J Appl Polym Sci. 105:1808–1816.
- Dias HMAM, Berbicz F, Pedrochi F, Baesso ML, Matioli G. 2010. Butter cholesterol removal using different complexation methods with beta-cyclodextrin, and the contribution of photoacoustic spectroscopy to the evaluation of the complex. Food Res Int. 43:1104–1110.
- Ergun B, Baydemir G, Andac M, Yavuz H, Denizli A. 2012. Ion imprinted beads embedded cryogels for in vitro removal of iron from β-thalassemic human plasma. J Appl Polym Sci. 125:254–262.
- Finette GMS, Qui-Ming M, Hearn MTW. 1997. Comparative studies on the isothermal characteristics of proteins adsorbed under batch equilibrium conditions to ion-exchange, immobilized metal ion affinity and dye affinity matrices with different ionic strength and temperature conditions. J Chromatogr A. 763:71–90.
- Garcia Rojas EE, Coimbra JSDR, Minim LA, Freitas JF. 2007. Cholesterol removal in liquid egg yolk using high methoxyl pectins. Carbohydr Polym. 69:72–78.
- Gurr M. 1992. Dietary lipids and coronary disease: old evidence, new perspective and progress. Lipid Res. 31:195–243.
- Han EM, Kim SH, Ahn J, Kwak HS. 2005. Cholesterol removal from homogenized milk with crosslinked β-cyclodextrin by adipic acid. Asian-Aust J Anim Sci. 18:1794–1799.
- Han EM, Kim SH, Ahn J, Kwak HS. 2008. Cholesterol-reduced cream cheese manufactured using crosslinked b-cyclodextrin to regular cream cheese. Asian-Aust J Anim Sci. 21:131–137.
- Koc I, Baydemir G, Bayram E, Yavuz H, Denizli A. 2011. Selective removal of 17b-estradiol with molecularly imprinted particle-embedded cryogel systems. J Hazard Mater. 192:1819–1826.
- Le Noir M, Plieva F, Hey T, Guieyse B, Mattiasson B. 2007. Macroporous molecularly imprinted polymer/cryogel composite systems for the removal of endocrine disrupting trace contaminants. J Chromatogr A. 1154:158–164.
- Liang ZP, Feng YQ, Liang ZY, Meng SX. 2005. Adsorption of urea nitrogen onto chitosan coated dialdehyde cellulose under biocatalysis of immobilized urease: equilibrium and kinetic. Biochem Eng J. 24:65–72.
- Noseda DG, Gentili HG, Nani ML, Nusblat A, Tiedtke A, Florin-Christensen J, Nudel CB. 2007. A bioreactor model system specifically designed for Tetrahymena growth and cholesterol removal from milk. Appl Microbiol Biotechnol. 75:515–520.
- Pyorala K. 1987. Dietary cholesterol in relation to plasma cholesterol and coronary heart disease. Am J Clin Nutr. 45:1176–1184.
- Rozner S, Garti N. 2006. The activity and absorption relationship of cholesterol and phytosterols. Colloids Surface A Physicochem Eng Aspects. 282–283:435–456.
- Sellergren B, Wieschemeyer J, Boos KS, Seidel D. 1998. Imprinted polymers for selective adsorption of cholesterol from gastrointestinal fluids. Chem Mater. 10:4037–4046.
- Sieber R. 1993. Cholesterol removal from animal food – Can it be justified? CH-3097 Fed. Dairy Res. Inst. Liebefeld, Switzerland. pp. 375–387.
- Umpleby RJ, Baxter SC, Chen Y, Shah RN, Shimizu KD. 2001. Characterization of molecularly imprinted polyers with the Langmuir-Freundlich isotherm. Anal Chem. 73:4584–4591.
- Xiong Q, Wilson WK, Pang J. 2007. The Liebermann–Burchard reaction: sulfonation, desaturation, and rearrangment of cholesterol in acid. Chem Mater Sci. 42:87–96.
- Yao K, Shen S, Yun J, Wang L, Chen F, Yu X. 2007. Protein adsorption in supermacroporous cryogels with embedded nanoparticles. Biochem Eng J. 36:139–146.
- Yavuz H, Karakoç V, Türkmen D, Say R, Denizli A. 2007. Synthesis of cholesterol imprinted polymeric particles. Int J Biol Macromol. 41:8–15.